Left ventricular distension and venting strategies for patients on venoarterial extracorporeal membrane oxygenation
Introduction
Venoarterial extracorporeal membrane oxygenation (VA ECMO) is now an established method of short-term mechanical support for patients with severe, refractory, cardiogenic shock. The number of patients being treated with VA ECMO has grown significantly, with recent data from the Extracorporeal Life Support Organization (ELSO) demonstrating nearly 10,000 patients were placed on ECMO during the 2017 calendar year, up from around 2,500 patients a decade prior (1). As our experience with ECMO grows, we are paying closer attention to previously underappreciated nuances of VA ECMO support, including left ventricular (LV) distension. VA ECMO generates increased afterload in the aorta, and the impaired LV has to pump against this supraphysiologic afterload. Given its already weakened state, the LV may not be able to generate sufficient power to eject blood, and the aortic valve (AV) remains closed throughout the cardiac cycle. LV distension may result, culminating in elevated pulmonary artery pressures, pulmonary edema, and hindered LV recovery.
In this review, we will analyze the physiologic basis of LV distension during VA ECMO and indications for LV venting in this critically ill patient population. We will also discuss the various methods of LV venting and the venting strategy we use at Columbia.
Physiologic basis and clinical manifestations of LV distension on VA ECMO
During VA ECMO, the arterial outflow cannula generates retrograde flow towards the AV, resulting in higher afterload on the heart than a normal physiologic state. This marked increase in afterload leads to LV distension, increased LV wall stress, and increased myocardial oxygen demands (Figure 1). The high LV end diastolic pressure (LVEDP) results in ongoing subendocardial ischemia, hindering LV recovery (2). This further impairs LV function, making LV recovery even more difficult, particularly in patients with acute cardiogenic shock due to myocardial infarction or myocarditis (3). Acute cardiogenic shock patients have a non-compliant LV and may have a competent mitral valve, and are at the greatest risk for LV distension. This is in comparison to patients with acutely decompensated chronic heart failure, with ventricles that are dilated at baseline and mitral valves that may be incompetent due to annular dilatation. Mitral regurgitation may serve as a “pop-off” for the LV, but lead to pulmonary edema. Thus, the incidence of LV distension may vary among patient populations.
LV distension results from blood filling the heart, but why does the LV get filled with blood during VA ECMO and what are the sources of blood? Two conditions must exist: (I) there must be a source of blood to fill the LV; and (II) the heart must be unable to completely eject it. For the latter, as discussed above, the AV may be unable to open in the setting of increased afterload from the ECMO circuit and decreased LV contractility from the acute cardiac injury. The trans-valvular gradient is high; in other words, the aortic pressure is significantly higher than LVEDP, and the AV is unable to open and eject blood, and blood stays within the LV. This is the answer to the first condition. In terms of blood source for the LV, this may include any aortic regurgitation, myocardial blood flow from the Thebesian veins and bronchial blood return, and, most significantly, any systemic venous return that is not captured by the ECMO venous cannula (4). This systemic venous return that enters the LV in antegrade fashion via the mitral valve is the most significant source of blood flow into the LV during VA ECMO. This scenario differs compared to that seen on cardiopulmonary bypass (CPB) with its reservoir and typical central cannulation with shorter, larger diameter cannulas; on CPB, the LV is completely decompressed. Given the lack of reservoir on VA ECMO, and longer, thinner peripheral venous cannulas with higher impedance, clinicians must turn to other options for managing circulating volume, including diuresis or central veno-venous hemodialysis (CVVH).
If the transvalvular gradient is too great, the AV will remain closed throughout the cardiac cycle, leading to blood stasis in the aortic root and potential thrombus formation, particularly in patients with peripheral VA ECMO cannulation (Figure 2). This is somewhat less likely to happen in patients with central cannulation, as there will be less aortic root blood stasis given the location of the outflow cannula or graft on the central aorta. However, root thrombus can lead to a catastrophic embolization down the coronary arteries, head vessels or body. The distended LV and elevated LV pressures will subsequently result in elevated left atrial and pulmonary pressures, leading to pulmonary edema, possible pulmonary hemorrhage, and subsequent systemic, cerebral and myocardial hypoxia (5-7). For all of these reasons, it is important to properly vent the LV during VA ECMO.
Our colleague Marc Dickstein has examined the impact of VA ECMO support on LV distension using computer modeling of the Starling curve (8). The Starling curve plots LV end-diastolic pressure against stroke volume, and explains the dependence of cardiac output on volume status. He found that in cases where VA ECMO is used to support patients in acute cardiogenic shock, the shift in the Starling curve is substantial, particularly due to the sensitivity of the impaired LV to afterload generated by the VA ECMO circuit. He concludes that there will be a dramatic rise in pulmonary capillary wedge pressure (PCWP) with LV distension as afterload is increased (8). The Starling relationship is unchanged by the addition of VA ECMO support, but the degree of LV distension and value of PCWP will depend on the patient’s LV contractility and afterload seen by the left heart. For patients in acute cardiogenic shock, the addition of VA ECMO with its consequent increase in afterload will result in significant increases in PCWP and LV distension.
Indications for LV venting
While on VA ECMO, if the patient’s heart is able to eject blood during the cardiac cycle, with the AV opening with every beat, and the arterial line maintains pulsatility of greater than 10 mmHg between the systolic and diastolic values, and PCWP remain low, this clinical scenario suggest that the LV may be adequately decompressed. Inotropes, vasopressors, and diuretics or CVVH can each be carefully titrated to achieve this goal. Additionally, ECMO flows may be titrated to the lowest acceptable level that generates adequate blood pressure, facilitating better ejection of blood from the heart, as afterload will be decreased with lower ECMO flows. This ‘partial flow’ strategy has been previously described as a successful approach to managing patients in post-cardiotomy shock (7). Patients in acute cardiogenic shock from myocarditis or ischemia would likely require more support than a partial flow strategy provides, and LV recovery in these patients should be optimized by a LV vent.
Yet there is no universally accepted definition of LV distension so it is crucial that ongoing clinical investigation be performed. LV distension can be reliably diagnosed on echocardiography, as evidenced by a dilated and hypocontractile LV, with or without severe mitral valve insufficiency. Stagnation of blood within the LV as seen on echo should also be a cause of concern. The use of a Swan-Ganz catheter is important, and can provide both pulmonary artery diastolic pressure and PCWP. In lieu of direct measurement of an LVEDP, PCWP is the best proxy. A pulmonary artery diastolic pressure greater than 25 mmHg and an elevated PCWP suggests that the LV is not properly decompressed. A distended LV may also potentiate arrhythmias; thus, the presence of ventricular arrhythmias is another indication for LV venting. In sum, our indications for placing an LV vent include any of the following: (I) an elevated PCWP; (II) a distended, hypocontractile LV or stagnation of blood in the LV as seen on echo; (III) an AV that remains closed throughout the cardiac cycle; (IV) marginal oxygenation and persistent pulmonary edema as evidenced on chest X-ray; and (V) refractory ventricular arrhythmias (9).
The importance of LV venting has been well-demonstrated. Initially described in detail in the pediatric ECMO literature in the late 1980s and early 1990s, LV hypertension hindered the usefulness of VA ECMO in neonatal respiratory failure patients and for circulatory support for post-cardiotomy failure in pediatric cardiac surgery (10-12). LV decompression was initiated in patients who after initial stabilization on VA ECMO, subsequently developed pulmonary edema and subsequent pulmonary hemorrhage from persistently high LV pressures. Various surgical and catheter-based techniques were developed, including the creation of nonrestrictive atrial septal defects by balloon or blade catheter. This use of left atrial unloading has proven beneficial by reducing the preload on the LV only. Since the LA drain is typically spliced into the venous limb of the circuit, this brings more blood back into the circuit and returns it to the patient via the arterial cannula, and therefore does not decrease the LV afterload and may in fact increase it. Thus, our techniques have continued to evolve, and our methods of venting now includes the full spectrum of surgical LV vents to percutaneous LV assist devices.
Over time, as ECMO use increased in the adult population, the prevalence and need for LV venting was re-established. In a recent study, we identified an incidence of LV distension mandating immediate decompression of 7%, and a “subclinical” (not warranting immediate decompression) incidence of 22%, in patients undergoing VA ECMO. In this same study, we noted that greater degrees of LV distension were associated with higher rates of device escalation and lower likelihood of achieving myocardial recovery. In particular, we found that in all refractory cardiogenic shock (RCS) patients on ECMO, and in those RCS patients that survived to discharge, increasing degrees of LV distension conferred a decreased likelihood of myocardial recovery (9). We believe that this was the first study that clearly demonstrated the negative impact of LV distension on myocardial recovery.
Treatment of LV distension
Medical management
Traditional methods for LV decompression range from medical management to surgical venting to percutaneous methods performed under fluoroscopy.
To begin with medical management, use of inotropes is essential in this patient population. Dobutamine and milrinone are the “go-to” agents that we use in our practice, however given that they provide only a modest increase in cardiac output and decrease in LV distension, we are quick to look to mechanical circulatory support. Other medical adjuncts include the use of diuretics and CVVH to help manage volume and vasopressors to maintain blood pressure (13).
Intra-aortic balloon pump (IABP)
In terms of mechanical circulatory support, one available percutaneous option is the IABP, which aids in LV decompression. The IABP has a well-known two-fold benefit: augmentation of coronary blood flow during diastole, and decreasing afterload. Animal studies have demonstrated a significantly decreased LV afterload in a VA ECMO model (14). Moreover, some centers routinely place an IABP for all patients on VA ECMO, convinced of its significant reduction of pulmonary artery wedge pressure. One European study demonstrated an important reduction in radiographic signs of pulmonary edema and more days off mechanical ventilation in patients with combined IABP-ECMO versus those patients that were just on VA ECMO (15). However, a recent large pooled analysis of greater than 1,500 patients showed no survival benefit with adding IABP to VA ECMO (16). Moreover, the negative results of this study fit with recent observational and randomized studies of IABP for patients with myocardial infarction and cardiogenic shock (17). Given that it does not directly vent the LV, we do not typically place an IABP for LV decompression.
Percutaneous left atrial vents
There is a myriad of other percutaneous options beyond that of the IABP, placed with the aid of fluoroscopy. Case reports and small case series dating back to the late 1980s and early 1990s have documented the feasibility of nonsurgical left heart venting in ECMO patients (18-20). These include trans-septal puncture and insertion of left atrial drain; trans-septal balloon and blade septostomy, and percutaneous insertion of a pulmonary artery or retrograde trans-aortic catheter functioning as a vent (21). Trans-septal balloon and blade septostomy remains a common practice in the pediatric population, but is less commonly performed in adults.
TandemHeart
The TandemHeart (LivaNova, Inc., London, England) is another method for circulatory support and indirect LV decompression during ECMO (22). The TandemHeart provides up to 5 LPM of support via a continuous flow centrifugal pump. The left atrium is accessed via a transseptal puncture, after femoral venous access has been established. Oxygenated blood is aspirated from the left atrium and can be connected into the venous limb of the ECMO circuit. This results in reduction of preload into the LV, but is not actually direct LV drainage. Moreover, the TandemHeart system requires a relatively large cannula, and a transseptal puncture can lead to a left-to-right shunt. Atrial suction events can occur more frequently than in a device with ventricular placement.
Open surgical placement of LV vent
A concomitant open chest procedure predisposes us to perform surgical venting. For instance, in patients who have failed to wean off CPB and are transitioned to VA ECMO, a surgical vent placed into the LV via the right superior pulmonary vein may be incorporated into the ECMO circuit via a Y-connector to the venous drainage limb. Intra-operative transthoracic echocardiography (TTE) can help assess LV distension and need for vent, and also guide placement. A vent placed into the LV apex is the most direct means of decompressing, however this must be performed with meticulous surgical technique and adequate hemostasis must be obtained. In non-post-cardiotomy patients, a surgical vent can still be placed into the LV apex via a left anterolateral thoracotomy.
Impella
Impella (Abiomed, Danvers, MA, USA) is a miniaturized micro-axial flow pump driven by an electrical motor with an inflow cannula. The pump is placed across the AV into the LV, aspirates blood from the LV cavity and expels it in the ascending aorta. Thus, it decreases the preload seen by the LV, while not increasing the afterload, as the bloodstream is directed away from the heart. The amount of cardiac output generated depends on pump speed and physiological afterload, and the position of the device is crucial for optimal function (23). The Impella has a built-in pressure signal that signifies correct pump placement and echocardiography may confirm this. It is placed via a percutaneous femoral arterial approach, through which the pump is placed into the LV over a stepwise-series of wires under fluoroscopic guidance. The pump is turned on, pump rotation is started and gradually increased. Drainage is from the LV and return is into the ascending aorta, and is continuous through the cardiac cycle and independent of arrhythmias. We believe this is one of the main benefits of the Impella: that it directly drains the LV. Correct positioning must be maintained to ensure adequate LV decompression. Full anticoagulation is required, however, and incorrect positioning can result in a significant risk of hemolysis (23).
A secondary and perhaps underappreciated benefit of the Impella is that given its antegrade flow into the aortic root, it prevents aortic root stasis and thrombus formation, an otherwise devastating complication that can lead to coronary embolism, stroke, or other vascular complications. This is particularly important in patients without pulsatility on the arterial line, which signifies that there is no AV opening or blood being ejected from the heart. The Impella also will allow for continued coronary circulation, given its transvalvular location.
ECpella
For patients in acute cardiogenic shock, particularly those subsequent to acute myocardial infarction, with evidence of LV distension, our resuscitation strategy of choice is through the simultaneous placement of VA ECMO and Impella CP, or “ECpella” (24) (Figure 3). This may be performed in the catheterization lab or operating room using fluoroscopic guidance. If the patient is undergoing bedside extracorporeal cardiopulmonary resuscitation (ECPR), we typically perform percutaneous ECMO cannulation, and then travel to the cath lab for Impella placement upon seeing evidence of LV distension. In other cases, where mean arterial blood pressure is stable, we may cut-down for placement of VA ECMO, and then move on to Impella under fluoroscopic guidance. In patients with small or diseased femoral arteries and we are concerned about inadequate flow to the legs on either the Impella side or the ECMO cannula side, we pre-emptively gain access to the superficial femoral artery also, to subsequently place a distal perfusion cannula.
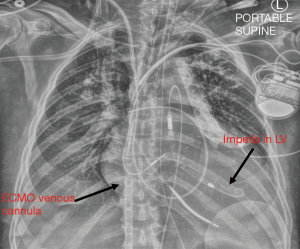
In general, while on ECpella support, we expect the Impella to generate between 1.5 to 2 LPM of flow to vent the heart, and adjust our power setting to achieve this goal. Whenever feasible, we use partial flow VA ECMO support of 3–4 LPM to preserve native myocardial contractility, keeping in mind the goal of end organ perfusion. The patient must have a right radial arterial line for oxygenation monitoring, and a Swan-Ganz catheter in place, which we use to check mixed venous saturation, PCWP, and pulmonary arterial pressure. Daily chest X-rays should be obtained to help assess degree of pulmonary edema, Impella position, and ECMO venous cannula position. Transesophageal echocardiography (TEE) or TTE may also be used to ensure the Impella remains in the correct position and that the left ventricle is adequately decompressed.
Once pressors have been weaned to low levels, the ECpella system may start to be weaned. We typically leave the Impella pump at 1.5 to 2 LPM, and gradually bring down the ECMO flows. Recovery of systolic function typically manifests as increasing pulsatility on the arterial wave form, with a steady increase in pulse pressure. ECMO is removed first, leaving the Impella in and maintaining some level of inotropy. If the patient needs additional level of support, the Impella can be uptitrated to higher flows, as the Impella CP can generate approximately 4 LPM of flows.
We have had success with this strategy. We recently demonstrated that with the use of combined Impella-VA ECMO support, our patients had greater rates of reduction of pulmonary arterial pressure and improved mixed venous oxygenation. Survival rates were similar between patients on VA ECMO support alone compared to those on combined ECpella support, despite the latter group of patients being sicker. This suggests that this combined approach may preserve survival in an otherwise challenging cohort of patients. Complications were minimal and equivalent between groups, with the exception of a greater rate of hemolysis in the ECpella group (24,25).
Other groups have reported similar results. Patel and colleagues reported a significantly lower 30-day all-cause mortality in patients on combined Impella-VA ECMO support which extended to one year, with no difference in secondary outcomes apart from a higher use of inotropes in VA ECMO support alone. The authors attributed the improved survival benefit with the direct LV venting from the Impella device, as demonstrated through their statistical analysis (26). Similarly, Tepper and associates from Washington University School of Medicine in St. Louis compared surgical venting and LV venting with an Impella for patients on VA ECMO. They concluded that use of an Impella was an effective means of LV unloading, and prevented worsened pulmonary edema, with outcomes and complications that are comparable to a surgical LV vent (27). Finally, from our European colleagues, Pappalardo et al. reported a significantly lower hospital mortality in patients in the VA ECMO and Impella group, compared to those patients just on ECMO. Furthermore, the VA ECMO-Impella patients had a higher rate of successful bridging to either further recovery or further therapy. As somewhat expected, there was a higher rate of hemolysis in the ECpella group, attributed to the Impella (28).
Minimally invasive LV venting with CentriMag LVAD and ECMO
We have developed a minimally invasive approach to LV venting through the use of a CentriMag ventricular assist device (VAD) integrated with ECMO (29) (Figure 4). In brief, this combines venous drainage from the femoral or jugular vein with drainage from the LV apex, and they are connected with together as inflow. Blood return is via the axillary artery or ascending aorta through hemi-sternotomy incision. There is an oxygenator spliced into the circuit as well, and an adjustable tube clamp is used to titrate the amount of drainage from each of the two inflow limbs (venous and LV apex) (Figure 4A). This approach has several benefits beyond ECpella including (I) perfusing oxygenated blood antegradely from the upper body; (II) unloading both the right and left ventricles efficiently; (III) potential for mobilizing the patient by removing the oxygenator and venous limb from the circuit (Figure 4B); (IV) potentially avoiding sternotomy, thereby avoiding a re-operative sternotomy for subsequent durable VAD placement or heart transplant.
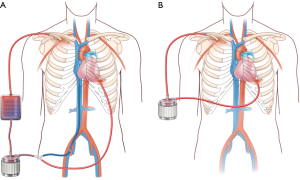
Conclusions
LV distension is an increasingly appreciated nuance of VA ECMO support. The consequences of failing to anticipate, recognize, and treat LV distension are grave, and may worsen an already distended and hypocontractile LV, making myocardial recovery more difficult, in addition to creating pulmonary edema and contributing to LV thrombus formation from blood stasis. We believe that direct venting is the best strategy for management of LV distension, as it decreases both preload and afterload as seen by the LV. We anticipate that in the future, smaller devices for LV venting will be developed, and be incorporated early on during the course of VA ECMO support, particularly for patients in acute cardiogenic support. Earlier recognition and aggressive management of LV distension is paramount in helping care for this critically ill patient population.
Acknowledgements
None.
Footnote
Conflicts of Interest: The authors have no conflicts of interest to declare.
References
- Extracorporeal life support registry report. International Summary, January 3, 2018. Extracorporeal Life Support Organization.
- Werdan K, Gielen S, Ebelt H, et al. Mechanical circulatory support in cardiogenic shock. Eur Heart J 2014;35:156-67. [Crossref] [PubMed]
- Barbone A, Malvindi PG, Ferrar P, et al. Left venticular unloading by percutaneous pigtail during extracorporeal membrane oxygenation. Interact Cardiovasc Thorac Surg 2011;13:293-5. [Crossref] [PubMed]
- Rajagopal K. Left ventricular distension in veno-arterial extracorporeal membrane oxygenation: from mechanics to therapies. ASAIO J 2019;65:1-10. [Crossref] [PubMed]
- Chen YS, Lin JW, Yu HY, et al. Cardiopulmonary resuscitation with assisted extracorporeal life-support versus conventional cardiopulmonary resuscitation in adults with in-hospital cardiac arrest: an observational study and propensity analysis. Lancet 2008;372:554-61. [Crossref] [PubMed]
- Combes A, Leprince P, Luyt CE, et al. Outcomes and long-term quality-of-life of patients supported by extracorporeal membrane oxygenation for refractory cardiogenic shock. Crit Care Med 2008;36:1404-11. [Crossref] [PubMed]
- Landes E, Truby L, Mundy L, et al. Partial flow with 15f arterial cannula provides sufficient circulatory support in veno-arterial extracorporeal membrane oxygenation. J Heart Lung Transplant 2014;33:S250. [Crossref]
- Dickstein ML. The Starling relationship and veno-arterial ECMO: ventricular distension explained. ASAIO J 2018;64:497-501. [Crossref] [PubMed]
- Truby LK, Takeda K, Mauro C, et al. Incidence and implications of left ventricular distention during venoarterial extracorporeal membrane oxygenation support. ASAIO J 2017;63:257-65. [Crossref] [PubMed]
- Koenig PR, Ralston MA, Kimball TR, et al. Balloon atrial septostomy for left ventricular decompression in patients receiving extracorporeal membrane oxygenation for myocardial failure. J Pediatr 1993;122:S95-9. [Crossref] [PubMed]
- O’Connor TA, Downing GJ, Ewing LL, et al. Echo-cardiographically guided balloon atrial septostomy during extracorporeal membrane oxygenation (ECMO). Pediatr Cardiol 1993;14:167-8. [Crossref] [PubMed]
- Ward KE, Tuggle DW, Gessouroun MR, et al. Transseptal decompression of the left heart during ECMO for severe myocarditis. Ann Thorac Surg 1995;59:749-51. [Crossref] [PubMed]
- Touchan J, Guglin M. Temporary mechanical circulatory support for cardiogenic shock. Curr Treat Options Cardiovasc Med 2017;19:77. [Crossref] [PubMed]
- Sauren LD, Reesink KD, Selder JL, et al. The acute effect of intra-aortic balloon counterpulsation during extra-corporeal life support: an experimental study. Artif Organs 2007;31:31-8. [Crossref] [PubMed]
- Bréchot N, Demondion P, Santi F, et al. Intra-aortic balloon pump protects against hydrostatic pulmonary oedema during peripheral venoarterial-extracorporeal membrane oxygenation. Eur Heart J Acute Cardiovasc Care 2018;7:62-9. [Crossref] [PubMed]
- Cheng R, Hachamovitch R, Makkar R, et al. Lack of Survival Benefit Found With Use of Intraaortic Balloon Pump in Extracorporeal Membrane Oxygenation: A Pooled Experience of 1517 Patients. J Invasive Cardiol 2015;27:453-8. [PubMed]
- Thiele H, Zeymer U, Neumann FJ, et al. Intraaortic balloon counterpulsation in acute myocardial infarction complicated by cardiogenic shock (IABP-SHOCK II): final 12 month results of a randomised, open-label trial. Lancet 2013;382:1638-45. [Crossref] [PubMed]
- Frazier EA, Faulkner SC, Paul PM, et al. Prolonged extracorporeal life support for bridging to transplant: technical and mechanical considerations. Perfusion 1997;12:93-8. [Crossref] [PubMed]
- Kurihara H, Kitamura M, Shibuya M, et al. Effect of transaortic catheter venting on left ventricular function during venoarterial bypass. ASAIO J 1997;43:M838-41. [Crossref] [PubMed]
- Vlasselaers D, Desmet M, Desmet L, et al. Ventricular unloading with a miniature axial flow pump in combination with extracorporeal membrane oxygenation. Intensive Care Med 2006;32:329-33. [Crossref] [PubMed]
- Seib PM, Faulkner SC, Erickson CC, et al. Blade and balloon atrial septostomy for left heart decompression in patients with severe ventricular dysfunction on extracorporeal membrane oxygenation. Catheter Cardiovasc Interv 1999;46:179-86. [Crossref] [PubMed]
- Nagpal AD, Singal RK, Arora RC, et al. Temporary Mechanical Circulatory Support in Cardiac Critical Care: A State of the Art Review and Algorithm for Device Selection. Can J Cardiol 2017;33:110-8. [Crossref] [PubMed]
- Lim HS. The effect of Impella CP on cardiopulmonary physiology during venoarterial extracorporeal membrane oxygenation. Artificial Organs 2017;41:1109-12. [Crossref] [PubMed]
- Akanni OJ, Takeda K, Truby LK, et al. EC-VAD: Combined use of extracorporeal membrane oxygenation and percutaneous microaxial pump left ventricular assist device. ASAIO J 2019;65:219-26. [Crossref] [PubMed]
- Koeckert MS, Jorde UP, Naka Y, et al. Impella LP 2.5 for left ventricular unloading during venoarterial extracorporeal membrane. J Card Surg 2011;26:666-8. [Crossref] [PubMed]
- Patel SM, Lipinski J, Al-Kindi SG, et al. Simultaneous venoarterial extracorporeal membrane oxygenation and percutaneous left ventricular decompression therapy with Impella is associated with improved outcomes in refractory cardiogenic shock. ASAIO J 2019;65:21-8. [Crossref] [PubMed]
- Tepper S, Masood MF, Garcia MB, et al. Left ventricular unloading by Impella device versus surgical vent during extracorporeal life support. Ann Thorac Surg 2017;104:861-7. [Crossref] [PubMed]
- Pappalardo F, Schulte C, Pieri M, et al. Concomitant implantation of Impella on top of veno-arterial extracorporeal membrane oxygenation may improve survival of patients with cardiogenic shock. Eur J Heart Fail 2017;19:404-12. [Crossref] [PubMed]
- Takeda K, Garan AR, Ando M, et al. Minimally invasive CentriMag ventricular assist device support integrated with extracorporeal membrane oxygenation in cardiogenic shock patients: a comparison with conventional CentriMag biventricular support configuration. Eur J Cardiothorac Surg 2017;52:1055-61. [Crossref] [PubMed]