Bleeding in continuous flow left ventricular assist device recipients: an acquired vasculopathy?
Durable mechanical circulatory support (MCS) has long held appeal as a solution to the growing imbalance between donor availability for heart transplantation and the increasing population of advanced heart failure (HF) as—unlike donor organs—it can be produced and utilized on demand. Despite steady and at times remarkable progress over the past half century, MCS did not become a reality until the advent of the continuous flow left ventricular assist devices (CF LVAD) in the early 2000s. It is now clear that CF LVADs improve not only survival but also functional capacity and quality of life in medically refractory HF. This has translated into exponential growth in CF LVAD utilization and for the first time in 2012, this therapy outpaced heart transplantation. While there will be continued refinement to this technology over the coming years, the overall principle will remain unchanged—a rotary pump with only one moving part to propel blood from the ventricle to the aorta. For this reason, the issue of hemocompatibility, i.e., the consequences of blood elements interfacing with the device during transit, will continue to challenge the field. Hemo(mal)compatibility during CF LVAD has to balance two opposing effects: device thrombosis and mucosal bleeding. While device thrombosis in an intravascular cavitary device was predictable and remains of concern, mucosal bleeding during CF LVAD was unanticipated and remains poorly understood.
At present, mucosal bleeding is the most common adverse event after CF LVAD, primarily manifesting as gastrointestinal bleeding (GIB). Numerous single center series have documented the annual incidence rate to range between 20% and 40% with the majority of initial cases occurring within the first 3 months of implantation. Although the exact pathophysiology of this process is still unclear, it has become apparent that there are two key factors that together account for the excess bleeding: (I) hematologic perturbations, specifically a CF LVAD induced bleeding diathesis and (II) anatomic lesions in the form of arteriovenous malformations (AVM). In this paradigm, the very high rate of AVMs remains an enigma and until now mechanistic data was scarce. For this reason, recent work from investigators at the University of Chicago which has shown a link between upregulated angiogenesis and AVM formation in CF LVAD subjects is a welcome stimulation for the field and paves the way for new investigation into this disease. In the current perspective, we aim to review the existing literature on mucosal bleeding, angiogenesis, and AVMs during CF LVAD and outline the next steps needed to help solve this enigma.
GIB in CF LVAD patients: two strikes and you are out?
Strike 1: CF LVAD induced bleeding diathesis
There is now strong evidence to support a bleeding diathesis as a result of hematologic perturbations induced during CF LVAD support. The most well-established of these is the acquired von Willebrand syndrome (avWS) (1). During CF LVAD support, blood is exposed to high shear stress during transit through the pump leading to a conformational change of von Willebrand factor (vWF), thus exposing the ADAMTS-13 cleavage site and ultimately increasing proteolysis of vWF (2,3). The consequence is a specific loss of the high molecular weight multimers (HMWM) of vWF which are considered to be a potent bridge in platelet adhesion to vascular endothelial surfaces, especially in areas of high blood velocity. All rotary pumps induce avWS and all patients are affected to some degree; however, not all patients experience clinical bleeding. Indeed, one study found that there was no correlation between the degree of decrease in HMWMs and the tendency for bleeding in patients with CF LVAD (4). Moreover, a recent study by Netuka et al. compared the effects of the HeartMate 3 and HeartMate II on degradation of HMWMs and found that, although the HeartMate 3 demonstrates a significantly greater preservation of HMWMs, platelet aggregation remained attenuated (5). Along these lines, an analysis from Klovaite et al. found that of 16 patients with a HeartMate II, 11 had a history of bleeding events, all of whom had impaired ristocetin-induced platelet aggregation (6). The authors found that this was a consequence of both avWS and attenuated platelet function. These observations suggest that the bleeding diathesis of CF LVADs is defined by both the avWS and impaired platelet function.
Strike 2: AVM
As experience with CF LVADs grew, it became apparent that a bleeding diathesis alone—even in conjunction with anticoagulation—could not fully explain the high rates of GIB. In particular, the observation that bleeding was often recurrent and frequently caused by AVMs (an otherwise uncommon source of GIB) suggested a new pathophysiology possibly induced by the CF pump (7). AVMs are characterized by angiodysplastic vessels that are ectatic and have thin walls with little or no smooth muscle. A variety of disease states are associated with AVMs, but in relation to CF LVAD, the parallels to Heyde’s syndrome provide the most commonly proposed mechanism. Heyde’s syndrome, originally described in 1958 refers to a high incidence of GIB from AVMs in the setting of shear stress induced avWs in patients with aortic stenosis (8). Similar to aortic stenosis, the decreased pulse pressure during continuous flow physiology may attenuate intestinal mucosal perfusion causing regional hypoxia which in turn triggers formation of new dysplastic vessels (9). Early studies in patients on non-pulsatile cardiopulmonary bypass circulation appear to at least corroborate the hypoxia portion of this theory, as it was shown that it can cause a 46% reduction in gastric mucosal blood flow (10). Whether this triggers angiogenesis and AVM formation, however, remains speculative.
In regard to the particular scenario of AVM bleeding in patients with avWS, it is interesting to note that prior literature in non-LVAD subjects has suggested that avWS may be a necessary pre-requisite for AVM bleeding. In a single center study, the vWF profiles of nine patients with bleeding AVMs of the GI tract were compared to nine patients with an incidental finding of AVMs (non-bleeding) (11). The investigators found that 8 of the 9 AVM bleeders had loss of the HMWMs consistent with avWS (seven of which were due to aortic stenosis) while all the non-bleeding AVM subjects had complete preservation of their HMWMs. These findings best support a 2-hit hypothesis whereby development of AVMs presents the initial lesion, however, in order for clinically significant bleeding to occur from these dysplastic vessels, one needs to have an additional dysfunction of platelets or the coagulation system as a “second hit”. In relation to CF LVAD, the development of avWS as one hit is well understood; however, what remains unclear is why AVMs are so prevalent?
Recent work from the University of Chicago (12) may provide an answer to this. Based on previous studies in gastrointestinal and brain AVMs (13) as well as angiogenesis in cancer (14), the authors postulated that CF LVAD bleeding is in part due to abnormal angiogenesis driven by dysregulation of the angiogenic factors angiopoietin (Ang)-1 and Ang-2. Ang-1 and Ang-2 are cytokines with a central role in angiogenesis. While Ang-1 is a critical factor in vessel maturation and endothelial quiescence, Ang-2 disrupts the connections between the endothelial and perivascular cells in the context of inflammation and promotes sprouting angiogenesis in conjunction with vascular endothelial growth factor (VEGF) (15). Ang-1 is released from pericytes and vascular smooth muscles cells upon stimulation with VEGF, while Ang-2 is stored in the Weibel-Palade bodies of endothelial cells (EC) and can be rapidly released in response to hypoxia, catecholamines, thrombin, histamine, and variety of proinflammatory mediators (16). A change in the ratio of Ang-1 to Ang-2 in favor of Ang-2 will promote endothelial activation and sprouting angiogenesis in the context of an endothelium primed by the VEGF and Notch pathways, a so called “angiogenic switch” (17). Furthermore, Ang-2 functions as a vessel-destabilizing autocrine molecule that promotes the endothelial actions of other cytokines such as TNF-alpha during inflammation (18).
In a series of elegant experiments, the investigators outlined a pathway by which elevated thrombin levels in CF LVAD patients, presumably from the intrinsic coagulation system, stimulate production of Ang-2 from ECs, which in turn leads to AVMs that predispose to nonsurgical bleeding (Figure 1). In the first set of experiments, they demonstrated that the blood from CF LVAD patients had statistically significantly higher levels of thrombin and Ang-2 compared to samples obtained from HF or orthotopic heart transplant (OHT) patients. Moreover, they found that factor XIIa and XIa where much higher in the CF LVAD group which suggests that the contact (intrinsic) coagulation system is responsible for the elevated thrombin levels.
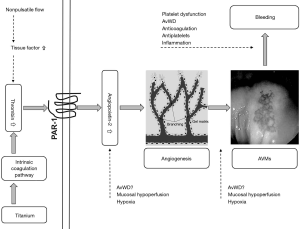
Next, in a set of in vitro experiments, the investigators showed that the high plasma levels of thrombin were likely responsible for Ang-2 over expression. Plasma from LVAD patients induced more Ang-2 gene expression in cultured ECs compared with the HF and OHT groups. Importantly, this effect was partially attenuated after co-incubation with the thrombin receptor blocker vorapaxar. In a subsequent step, they showed that serum from patients with LVADs induced more endothelial tube formation after incubation with cultured ECs than did serum from HF or OHT patients. This effect in the LVAD group was eliminated in the presence of an Ang-2 blocking antibody suggesting that Ang-2 is responsible for the observed increased angiogenesis.
Finally, in an attempt to connect the findings to clinical bleeding, the authors examined the relationship between mucosal bleeding and serum Ang-2 levels. In a retrospective analysis, they found that in the first 3 months after implant, 5 of the 13 subjects with an Ang-2 level above the group mean had experienced a bleeding event whereas none of the 25 subjects with a level below the mean had experienced bleeding (P=0.003). Importantly, all bleeding events were confirmed to be from an AVM source. Somewhat perplexingly, the relationship between Ang-2 and bleeding disappeared at 6 months.
Ultimately, the investigators in a series of sophisticated experiments have attempted to make a link between high thrombin levels, angiogenesis and mucosal bleeding from AVMs in CF LVAD patients. Of course, these in vitro experiments do not allow us to make direct connections and these findings need to be clinically validated. Nonetheless, the results of this work have added to our current understanding of CF LVAD induced AVM bleeding and as a result a number of new issues have arisen and deserve further investigations. Below, we have highlighted what we feel are the most pressing ones.
Unanswered questions
Role of pulsatility
In the context of the existing data, it is difficult to fully eliminate low pulsatile flow from the bleeding equation. There is now strong evidence from multiple centers documenting the relationship between non-invasive measures of pulsatility and AVM GIB. This was first described by Wever-Pinzon et al. who reviewed a single center experience of 134 patients and found that the HeartMate II Pulse Index (maximum flow-minimum flow/average flow over 15 seconds) was associated with the risk of non-surgical bleeding in the first 90 days after implantation (19). In a specific analysis of AVM-related GIB the investigators also found a significant association when the Pulse Index was examined as a continuous variable. Since this original publication, we have found similar results in our own patient cohort (unpublished data) as have other investigators (20).
Although these studies have demonstrated an association between pulsatility and AVM bleeding, mechanistic studies linking the two are lacking. In this regard, one potential mechanism that deserves investigation is the question of thrombin origin. In the current study, the investigators postulated that thrombin was elevated due to activation of the intrinsic coagulation system from the titanium components in the device. This assumption was based solely on the finding of elevated factor XIIa in LVAD subjects. Although this is fully plausible, it has also been shown that steady shear stress, as a characteristic of non-pulsatile blood flow, leads to an overexpression of tissue factor on EC and thus increased production of thrombin through activation of extrinsic coagulation cascade (21,22). This may suggest an alternative hypothesis in that thrombin is up-regulated by low pulsatile flow and, in light of the findings of Tabit et al., could link pulsatility to AVM formation. This is a hypothesis that deserves prioritization for study.
Mechanism of Ang-2 production
An additional unsolved problem is the mechanism of increased Ang-2 production from EC. The authors, by utilizing a protease activated receptor 1 (PAR-1) blocker, demonstrated that Ang-2 gene expression is significantly but not fully driven by the increased levels of thrombin in CF LVAD patients. Therefore, it is quite possible that other pathways leading to Ang-2 gene expression could be involved. Most interesting is the novel observation that in vitro loss of vWF can induce Ang-2 release from EC resulting in sprouting angiogenesis (23). Whether a selective decrease of the HMWMs of vWF as seen in CF LVAD subjects can cause a similar effect is unknown and warrants investigation. It is also important to note that 10% of patients with congenital vWD develop AVMs (24,25). When considered together, these are intriguing findings and, contrary to the proposed paradigm, may imply that vWD is not only the second hit but also causal in AVM formation.
In addition to thrombin and vWF loss, numerous other proinflammatory and proangiogenic factors can either up regulate production or increase release of Ang-2 from Weibel-Palade bodies. As there is significant evidence of the presence of inflammation in CF LVAD recipients (26), it is possible that proinflammatory cytokines contribute to the increased production of Ang-2. Finally, although none of the patients in the study had systemic hypoxia, we cannot exclude the development of regional hypoxia at the mucosal level due to low pulsatile flow, which then triggered Ang-2 production. Understandably, pursuing this hypothesis was not practical within the scope of their study but nevertheless should be explored further.
Ang-2 and AVM in vivo
As noted earlier, the authors found a relatively weak clinical temporal association between Ang-2 levels and AVM bleeding (i.e., present at 3 months but not 6 months). There are a number of possible explanations for this discrepancy including changes in antiplatelet medications, INR level, small sample size and the cross-sectional nature of the study. Nonetheless, this is a very important limitation since the primary clinical relevance of their work is to define the pathway leading to AVM development. Furthermore, as the authors note, we do not have information on the existence of asymptomatic AVMs in non-bleeders which would be critical to truly establish the association between Ang-2 levels and AVMs. Such an in vivo analysis will never be practical due to the length and accessibility of the GI tract. We feel for future studies to be of substantive value, this limitation needs to be overcome and thus surrogates of gastrointestinal AVMs must be identified. Whether this is accomplished through evaluation of the nasal mucosa, as we have recently shown (27), or through the microcirculation (24) requires further study.
Translational outlook
If the findings of this study are clinically validated, a number of potential therapeutic interventions could be considered. The authors propose a very interesting possibility of using pharmacological inhibition of Ang-2 with the goal of reducing AVM formation. Currently, there are multiple drugs for the treatment of neoplastic diseases in development targeting this pathway, some of which are already in phase III clinical trials. While this seems like an appealing option, the side effects of the long-term therapy in non-cancer patients may be unfavorable in context of multiplicity of Ang-2 regulatory roles in vascular remodeling, inflammation, and lymphatic function (28). This is an area that bears watching in the near future.
There are alternative therapeutic approaches that can also be considered, including treatment directed at the level of thrombin or its receptor PAR-1. For example, use of voraxapar, a PAR-1 blocker, in lieu of aspirin as an antiplatelet agent; though the observed increased risk of intracranial hemorrhage noted in patients with a history of stroke and treated with voraxapar is concerning (29). A more intriguing option is the use of a direct thrombin inhibitor such as dabigatran instead of warfarin. It has been previously shown that there is residual thrombin activity in patients on fully therapeutic anticoagulation with warfarin (30). This opens the question of whether treatment with dabigatran may serve not only as anticoagulation to prevent pump thrombosis but also to reduce mucosal bleeding via greater inhibition of thrombin mediated Ang-2 production. While there is some concern with such an approach in light of the failed use of dabigatran in patients with mechanical valves (31), a recent small series by Terrovitis et al. showed that dabigatran is safe and effective in patients with the HeartMate II (32). Of course the benefits of switching anticoagulation from the time-tested warfarin are hypothetical and require careful evaluation in the clinical arena.
Conclusions
CF LVAD support induces a new and unique vascular and hematological pathology ultimately leading to an increased incidence of mucosal bleeding. At present, the precise mechanisms underlying this pathophysiology remain to be elucidated to formulate preventative or therapeutic options. In the interim, Tabit et al. are to be congratulated for leading the way with their elegant study.
Acknowledgements
None.
Footnote
Provenance: This is an invited Editorial commissioned by the Section Editor Kai Zhu (Department of Cardiac Surgery, Zhongshan Hospital Fudan University, Shanghai, China).
Conflicts of Interest: The authors have no conflicts of interest to declare.
References
- Uriel N, Pak SW, Jorde UP, et al. Acquired von Willebrand syndrome after continuous-flow mechanical device support contributes to a high prevalence of bleeding during long-term support and at the time of transplantation. J Am Coll Cardiol 2010;56:1207-13. [Crossref] [PubMed]
- Tsai HM, Sussman II, Nagel RL. Shear stress enhances the proteolysis of von Willebrand factor in normal plasma. Blood 1994;83:2171-9. [PubMed]
- Geisen U, Heilmann C, Beyersdorf F, et al. Non-surgical bleeding in patients with ventricular assist devices could be explained by acquired von Willebrand disease. Eur J Cardiothorac Surg 2008;33:679-84. [Crossref] [PubMed]
- Meyer AL, Malehsa D, Budde U, et al. Acquired von Willebrand syndrome in patients with a centrifugal or axial continuous flow left ventricular assist device. JACC Heart Fail 2014;2:141-5. [Crossref] [PubMed]
- Netuka I, Kvasnička T, Kvasnička J, et al. Evaluation of von Willebrand factor with a fully magnetically levitated centrifugal continuous-flow left ventricular assist device in advanced heart failure. J Heart Lung Transplant 2016;35:860-7. [Crossref] [PubMed]
- Klovaite J, Gustafsson F, Mortensen SA, et al. Severely impaired von Willebrand factor-dependent platelet aggregation in patients with a continuous-flow left ventricular assist device (HeartMate II). J Am Coll Cardiol 2009;53:2162-7. [Crossref] [PubMed]
- Demirozu ZT, Radovancevic R, Hochman LF, et al. Arteriovenous malformation and gastrointestinal bleeding in patients with the HeartMate II left ventricular assist device. J Heart Lung Transplant 2011;30:849-53. [PubMed]
- Warkentin TE, Moore JC, Anand SS, et al. Gastrointestinal bleeding, angiodysplasia, cardiovascular disease, and acquired von Willebrand syndrome. Transfus Med Rev 2003;17:272-86. [Crossref] [PubMed]
- Crow S, John R, Boyle A, et al. Gastrointestinal bleeding rates in recipients of nonpulsatile and pulsatile left ventricular assist devices. J Thorac Cardiovasc Surg 2009;137:208-15. [Crossref] [PubMed]
- Ohri SK, Somasundaram S, Koak Y, et al. The effect of intestinal hypoperfusion on intestinal absorption and permeability during cardiopulmonary bypass. Gastroenterology 1994;106:318-23. [Crossref] [PubMed]
- Veyradier A, Balian A, Wolf M, et al. Abnormal von Willebrand factor in bleeding angiodysplasias of the digestive tract. Gastroenterology 2001;120:346-53. [Crossref] [PubMed]
- Tabit CE, Chen P, Kim GH, et al. Elevated Angiopoietin-2 Level in Patients With Continuous-Flow Left Ventricular Assist Devices Leads to Altered Angiogenesis and Is Associated With Higher Nonsurgical Bleeding. Circulation 2016;134:141-52. [Crossref] [PubMed]
- Hashimoto T, Lam T, Boudreau NJ, et al. Abnormal balance in the angiopoietin-tie2 system in human brain arteriovenous malformations. Circ Res 2001;89:111-3. [Crossref] [PubMed]
- Nasarre P, Thomas M, Kruse K, et al. Host-derived angiopoietin-2 affects early stages of tumor development and vessel maturation but is dispensable for later stages of tumor growth. Cancer Res 2009;69:1324-33. [Crossref] [PubMed]
- Fagiani E, Christofori G. Angiopoietins in angiogenesis. Cancer Lett 2013;328:18-26. [Crossref] [PubMed]
- Fiedler U, Scharpfenecker M, Koidl S, et al. The Tie-2 ligand angiopoietin-2 is stored in and rapidly released upon stimulation from endothelial cell Weibel-Palade bodies. Blood 2004;103:4150-6. [Crossref] [PubMed]
- Felcht M, Luck R, Schering A, et al. Angiopoietin-2 differentially regulates angiogenesis through TIE2 and integrin signaling. J Clin Invest 2012;122:1991-2005. [Crossref] [PubMed]
- Fiedler U, Reiss Y, Scharpfenecker M, et al. Angiopoietin-2 sensitizes endothelial cells to TNF-alpha and has a crucial role in the induction of inflammation. Nat Med 2006;12:235-9. [Crossref] [PubMed]
- Wever-Pinzon O, Selzman CH, Drakos SG, et al. Pulsatility and the risk of nonsurgical bleeding in patients supported with the continuous-flow left ventricular assist device HeartMate II. Circ Heart Fail 2013;6:517-26. [Crossref] [PubMed]
- Jabbar HR, Abbas A, Ahmed M, et al. The Incidence, Predictors and Outcomes of Gastrointestinal Bleeding in Patients with Left Ventricular Assist Device (LVAD). Dig Dis Sci 2015;60:3697-706. [Crossref] [PubMed]
- Yin W, Shanmugavelayudam SK, Rubenstein DA. The effect of physiologically relevant dynamic shear stress on platelet and endothelial cell activation. Thromb Res 2011;127:235-41. [Crossref] [PubMed]
- John R, Panch S, Hrabe J, et al. Activation of endothelial and coagulation systems in left ventricular assist device recipients. Ann Thorac Surg 2009;88:1171-9. [Crossref] [PubMed]
- Starke RD, Ferraro F, Paschalaki KE, et al. Endothelial von Willebrand factor regulates angiogenesis. Blood 2011;117:1071-80. [Crossref] [PubMed]
- Koscielny JK, Latza R, Mürsdorf S, et al. Capillary microscopic and rheological dimensions for the diagnosis of von Willebrand disease in comparison to other haemorrhagic diatheses. Thromb Haemost 2000;84:981-8. [PubMed]
- Duray PH, Marcal JM Jr. Gastrointestinal angiodysplasia: a possible component of von Willebrand's disease. Hum Pathol 1984;15:539-44. [Crossref] [PubMed]
- Grosman-Rimon L, Billia F, Fuks A, et al. New therapy, new challenges: The effects of long-term continuous flow left ventricular assist device on inflammation. Int J Cardiol 2016;215:424-30. [Crossref] [PubMed]
- Patel SR, Madan S, Saeed O, et al. Association of Nasal Mucosal Vascular Alterations, Gastrointestinal Arteriovenous Malformations, and Bleeding in Patients With Continuous Flow Left Ventricular Assist Devices. JACC Heart Fail 2016. [Epub ahead of print]. [Crossref] [PubMed]
- Gale NW, Thurston G, Hackett SF, et al. Angiopoietin-2 is required for postnatal angiogenesis and lymphatic patterning, and only the latter role is rescued by Angiopoietin-1. Dev Cell 2002;3:411-23. [Crossref] [PubMed]
- Morrow DA, Alberts MJ, Mohr JP, et al. Efficacy and safety of vorapaxar in patients with prior ischemic stroke. Stroke 2013;44:691-8. [Crossref] [PubMed]
- Massicotte P, Leaker M, Marzinotto V, et al. Enhanced thrombin regulation during warfarin therapy in children compared to adults. Thromb Haemost 1998;80:570-4. [PubMed]
- Eikelboom JW, Connolly SJ, Brueckmann M, et al. Dabigatran versus warfarin in patients with mechanical heart valves. N Engl J Med 2013;369:1206-14. [Crossref] [PubMed]
- Terrovitis JV, Ntalianis A, Kapelios CJ, et al. Dabigatran etexilate as second-line therapy in patients with a left ventricular assist device. Hellenic J Cardiol 2015;56:20-5. [PubMed]