Bone scan findings of chest wall pain syndrome after stereotactic body radiation therapy: implications for the pathophysiology of the syndrome
Case report
A 72-year-old woman presented with an 11 mm adenocarcinoma of the left lower lobe. Her prior history is significant for a history of smoking and chronic obstructive pulmonary disease (COPD), as well as previous pT1N0, stage I non-small cell lung cancer (NSCLC) of the left upper lobe treated ten years prior with lobectomy alone. Physical exam revealed a well-appearing female breathing comfortably at rest and was otherwise normal with a body mass index (BMI) of 21. The current lesion was detected on surveillance imaging. There was no imaging evidence of hilar or mediastinal adenopathy. A positron emission tomography-computed tomography scan (PET-CT) confirmed local disease only. A CT-guided biopsy of the left lung mass showed moderately differentiated adenocarcinoma. She was staged as cT1cN0, stage I. She was evaluated for resection by thoracic surgery and was not thought to be a surgical candidate due to poor lung function and an extensive cardiac history inclusive of two prior myocardial infarctions and three-vessel coronary artery bypass grafting. Her history at this time was notable for significant dyspnea on exertion with no shortness of breath at rest. She endorsed a chronic mild dry cough and she denied chest pain.
She underwent stereotactic body radiation therapy using a modified dynamic arc technique using six arcs. The patient was treated supine with the arms down per institutional protocol to limit intrafractional motion. The planning target volume, which measured 22.3 cc, was generated from an internal target volume. The chest wall was an organ at risk and the V30 (V30: volume of organ at risk receiving 30 Gy or more) was 17.5 cc, the V40 was 5.3 cc, and the V60 (V60: volume of organ at risk receiving 60 Gy or more) was 0.09 cc. The max dose to the chest wall was 64.43 Gy. She was treated to 54 Gy in three fractions over five days without incident. Figure 1 shows the axial and coronal isodose curves of the delivered plan.
Six weeks after radiotherapy, she noticed erythema corresponding to the higher dose isodose lines (“isodose” erythema) over the lateral chest wall and adjacent arm. Coincident with this, she began to experience grade I chest wall discomfort in the treated area. Over the ensuing six months, this pain increased to Grade II with interference with sleep in the left lateral decubitus position and housework. She also began to appreciate pain radiating around the left side of the chest underneath the breast in a dermatomal distribution. Restaging CT scans failed to note any disease recurrence or abnormality in the left chest wall. At nine months a bone scan showed nonspecific and heterogeneous increased radiotracer uptake in the left thorax most prominent in the lateral sixth and seventh ribs as shown in Figure 2. Dosimetry was reviewed and the area of the bone scan uptake was found to correlate extremely well with the V30 of the ribs. The left panel of Figure 3 shows a color wash representation of surface dose to the ribs (V30) from the delivered plan. The right panel of Figure 3 shows a lateral bone scan view showing excellent concordance between the V30 and the area of scintigraphic uptake.
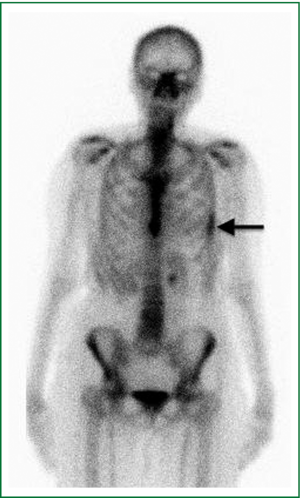
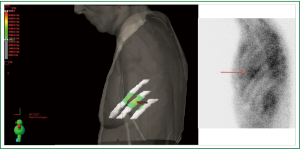
Discussion
Long-term chest wall complications of SBRT can include chest wall pain, rib fracture, and skin changes including fibrosis and erythema. The rate of long-term grade one or higher chest wall toxicity after SBRT is 17% to 21% for lesions near the chest wall and 4% for non-peripheral lesions (1,2). Chest wall pain syndrome can manifest months to years after treatment with a median onset of greater than 6 months. It is characterized by positional pain that can be pleuritic and is often worsened by activity. The pain can range from mild to severe. It is sometimes accompanied by rib fracture, although pain does occur in the absence of fracture, and many patients with rib fracture are pain free (3,4).
At present the exact dose volume parameters for chest wall toxicity are uncertain. Several retrospective reports have identified dosimetric and clinical factors as predictors for chest wall toxicity. Stephans et al. reviewed 134 patients treated with 60 Gy in three fractions and found that the V30 and V60 to the chest wall were statistically significant predictors of chest wall pain syndrome on multivariate analysis (5). They suggest dose limits to the chest wall of V30 <30 cc and a V60 <3 cc to keep late chest wall toxicity risk at 10% to 15%. In another large retrospective analysis, Andolino et al found that a V40 <5 cc resulted in a 10% risk of any grade toxicity while a V40 >15 cc resulted in a 30% risk (1). They also found a sharp increase in toxicity when the max dose to the chest wall was >50 Gy.
Certain patient characteristics such as obesity, diabetes mellitus, and smoking have been found inconsistently to be related to chest wall toxicity (2,5). Smoking and diabetes may contribute to increased inflammation, vasculopathy and delayed healing. Obesity may be associated with increased hot spots in the chest wall in cases where the entrance dose needs to be elevated in order to overcome tissue attenuation and deliver tumor ablative doses to the PTV, and it is uncertain whether this is an independent factor in chest wall toxicity.
At present, the pathogenesis of chest wall pain syndrome following radiation therapy is poorly understood, although cortical thinning has been described (4). It has been proposed that peripheral nerve injury might be a component of this syndrome (5). Metastatic bone disease is felt to be painful in part due to loss of structural integrity of the bone with resultant mechanical weakness (6). Physical stresses applied to a weakened bone then result in pain. It may well be that a similar pathogenesis is responsible here, with radiotherapy causing weakening of the bone and pain from mechanical stress.
This is the first report in the literature of chest wall pain syndrome accompanied by findings on bone scan. Heterogeneous uptake was observed on bone scan along the 6th and 7th ribs over a diffuse area corresponding to treated chest wall (Figure 2). While the precise reason for increased scintigraphic uptake in the treated area cannot be known, the relationship may be postulated. Bone scans use Technetium 99m labeled organophosphate (such as Tc99m MDP) intravenously, which preferentially binds to hydroxyapatite moieties in bone. Differential binding has been suggested to be due to the amount of osteoid formation, blood flow, mineralization of osteoid, and/or sympathetic nerve supply (7). To explain the scintigraphic uptake seen in this case, we would propose that SBRT results in bone degeneration, which prompts an increase in bone repair via increased osteoblastic deposition of osteoid and mineralization. This could be combined with an increased inflammatory state associated with increased blood flow. Given this possible pathogenesis, bisphosphonate therapy could be investigated as a useful treatment option in patients with chest wall pain following SBRT.
In conclusion, this is the first report in the literature of bone scan findings of chest wall pain syndrome following SBRT. This suggests a possible pathogenesis for this syndrome whereby SBRT weakens the bone leading to pain that is associated with osteoblastic bone remodeling. Further study is needed to replicate this finding and to determine the clinical usefulness of bone scan in this setting. Based on the present report, bone scan, while often ordered because of suspicion of bony metastatic disease, may also be helpful in supporting the diagnosis of chest wall pain syndrome.
Acknowledgements
Disclosure: The authors declare no conflict of interest.
References
- Andolino DL, Forquer JA, Henderson MA, et al. Chest wall toxicity after stereotactic body radiotherapy for malignant lesions of the lung and liver. Int J Radiat Oncol Biol Phys 2011;80:692-7.
- Welsh J, Thomas J, Shah D, et al. Obesity increases the risk of chest wall pain from thoracic stereotactic body radiation therapy. Int J Radiat Oncol Biol Phys 2011;81:91-6.
- Pettersson N, Nyman J, Johansson KA. Radiation-induced rib fractures after hypofractionated stereotactic body radiation therapy of non-small cell lung cancer: a dose- and volume-response analysis. Radiother Oncol 2009;91:360-8.
- Voroney JP, Hope A, Dahele MR, et al. Chest wall pain and rib fracture after stereotactic radiotherapy for peripheral non-small cell lung cancer. J Thorac Oncol 2009;4:1035-7.
- Stephans KL, Djemil T, Tendulkar RD, et al. Prediction of Chest Wall Toxicity From Lung Stereotactic Body Radiotherapy (SBRT). Int J Radiat Oncol Biol Phys 2012;82:974-80.
- Chow E, Finkelstein JA, Coleman RE. Metastatic cancer to the bone. In: DeVita VT, Lawrence TS, Rosenberg SA, eds. DeVita, Hellman, and Rosenberg's Cancer: Principles & Practice of Oncology. 8th ed. Philadelphia, Pa: Lippincott Williams & Wilkins;2008:2510-22.
- Mettler F, Guiberteau, MJ. Essentials of nuclear medicine imaging. Saunders/Elsevier, Philadelphia, PA 2006.