Monocytes and macrophages in cardiac injury and repair
Monocytes/macrophages during cardiac homeostasis
In the steady state the heart is populated with cardiac resident macrophages (Figure 1A) (1-3). These cells exhibit a spindle-like shape and are interspersed between cardiomyocytes (4). Under physiological and pathological conditions, cardiac resident macrophages appear not to be a homogenous population, but rather comprise distinct subsets, depending on expression levels of Ly6C, CCR2, CD11c, and histocompatibility-2 (5). In mice, these macrophages populate the heart during embryogenesis and originate primarily from yolk sac progenitors. Once residing in the heart, these macrophages self-maintain through local proliferation and seem to be independent of bone marrow (BM) derived blood monocytes (3,5). However, it has been proposed that blood monocyte-derived macrophages also seed the heart and gradually replace cardiac resident macrophages (6). The function of cardiac resident macrophages during cardiac homeostasis is less well investigated and may include phagocytosis of aged or dying cardiomyocytes, angiogenesis, fibrosis, and immunosurveillance (antigen processing and presenting) (7).
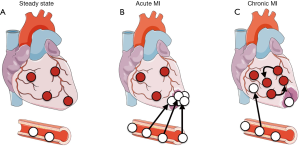
Monocytes/macrophages during acute myocardial infarction (MI)
MI, the most dreadful complication of atherosclerosis, occurs after atherosclerotic plaque rupture/erosion resulting in atherothrombosis which blocks the supply of oxygenated blood to the heart. Resident macrophages from the ischemic area disappear, probably because they become necrotic and/or apoptotic (Figure 1B) (8,9). MI inflicts a sterile wound on the heart that recruits hundreds of thousands of leukocytes from the blood to the ischemic heart (8). After a neutrophil peak, inflammatory blood Ly6Chigh monocytes are recruited and accumulate in the infarct area. Recruited Ly6Chigh monocytes give rise to inflammatory macrophages, which both remove dead cells and extracellular matrix debris by phagocytosis and secret proteases and reactive oxygen species.
Blood monocytes contribute to up to 10% of the total blood leukocyte counts in both mice and humans (2) and are a heterogeneous population (10). There are two subsets of circulating CD115+ monocytes in mice, Ly6Chigh (CCR2+CD62L+CX3CR1mid) and Ly6Clow (CCR2−CD62L−CX3CR1hi) monocytes.
The inflammatory Ly6Chigh monocytes are recruited to the heart following ischemic injury at a high rate, produce high levels of proinflammatory cytokines [such as tumor necrosis factor-α (TNFα) and interleukin-1β (IL-1β)], and differentiate into inflammatory macrophages after extravasation (11). Recruitment of Ly6Chigh monocytes relies on the interaction of monocytic CCR2 and endothelial chemokine (C-C motif) ligand 2 (CCL2)/chemokine (C-C motif) ligand 7 (CCL7) (12). Apart from these chemokines, cell adhesion molecules expressed on both, blood monocytes and cardiac endothelial cells, also contribute to enhanced leukocyte recruitment. It was reported that intercellular adhesion molecule 1 (ICAM1), vascular cell adhesion protein 1 (VCAM1), E- and P-selectin are upregulated in the remote myocardium (the non-ischemic part) in mice with heart failure with reduced ejection fraction (HFrEF) (3) and that simultaneous blocking of endothelial ICAM1, ICAM2, VCAM1, E- and P-selectin reduced monocyte recruitment in the setting of acute MI (13).
The Ly6Clow monocytes patrol the vascular lumen, adhere to and move along the endothelium, both clearing damaged cells and triggering inflammatory responses (14-16). In the blood, Ly6Chigh monocytes can convert to Ly6Clow monocytes (17). Whether Ly6Clow monocytes are recruited to the heart and contribute to the cardiac macrophage pool is currently discussed (8,18).
The human blood harbors three subsets of monocytes: Classical inflammatory monocytes (CD14+CD16−, resembling murine Ly6Chigh monocytes), non-classical monocytes (CD14lowCD16+ monocytes, resembling murine Ly6Clow monocytes), and an intermediate monocyte subset (CD14++CD16+CCR2+CX3CR1++CCR5+) (19).
Splenic monocyte release
The infarct recruits hundreds of thousands of leukocytes per day, a process that depletes the blood pool quickly. In order to meet this high demand, leukocytes are released from emergency reservoirs. In mice, the spleen functions as such a monocyte reservoir and contributes ~50% of myeloid cells that reside in the infarct in the early hours after coronary ligation (20). Monocyte release from the red pulp of the spleen thereby depends on angiotensin II/angiotensin 1 receptor interplay. Angiotensin II administration mimicked the release of splenic monocytes into the blood as observed after MI (21). Moreover, splenectomy leads to adverse infarct healing indicating that splenic monocytes may have a protective role (22). Accordingly, administration of the angiotensin-converting-enzyme inhibitor enalapril early after MI did not improve survival (23).
Splenic monocyte production
Apart from releasing leukocytes in the setting of MI (20), the spleen can function as a leukocyte production site (extramedullary hematopoiesis) especially during later stages of MI (24). The MI activates the sympathetic nervous system of the BM which releases its neurotransmitter norepinephrine. Norepinephrine signals to BM parenchymal cells that lower the expression levels of Cxcl12, Scf, and Vcam1. This in turn results in release of hematopoietic stem and progenitor cells into the blood. These cells seed the spleen and induce extramedullary hematopoiesis (24).
BM monocyte production
The increased demand for myeloid cells also fuels emergency hematopoiesis. Monocytes are mononuclear phagocytes that are generated at medullary or extramedullary sites in a process termed myelopoiesis. Granulocyte/macrophage precursors (GMPs) originate from hematopoietic stem and progenitor cells and mature into macrophage (monocyte)/dendritic cell precursors (MDPs), which give rise to Ly6Chigh monocytes through common monocyte progenitors (cMoPs) (25).
The role of the BM in the inflammatory response that follows MI was recently investigated (26). BM hematopoietic stem cells (HSC) are activated after MI and give rise to a subset of CCR2+ HSCs that proliferate vigorously in response to ischemia increasing the production of myeloid cells (monocytes, macrophages, neutrophils). These myeloid cells are deployed to the cardiac wound and promote infarct healing. In patients with acute MI, a similar BM activation was observed after ischemia (26).
Further, the signals that drive this BM activation after MI were studied (27). A crosstalk of heart and BM is established after MI: the pro-inflammatory cytokine IL-1β is released from the ischemic heart, signals to the BM and drives HSC proliferation leading to an increase in production of myeloid cells. BM reconstitution experiments revealed that this increase in HSC proliferation occurs through a direct action of IL-1β on HSC, but also indirectly by altering the HSC microenvironment (HSC niche). Here, niche cells lower the expression of HSC maintaining factors and hence trigger proliferation and release of HSC. Anti-IL-1β treatment dampened BM activation, decreased production of myeloid cells and ultimately lowered the content of inflammatory cells in the infarcted tissue. In turn, this resulted in a better post-MI remodeling (27).
BM monocyte release
Monocyte release from the BM depends on CCL2/CCR2 and CCL7/CCR2 signaling (28,29). Upon stimulation, BM stromal cells produce CCL2 that can be sensed by CCR2+ monocytes, which then egress out of the BM into the circulation (30). Genetically modified mice lacking CCR2 do not only show an impaired recruitment of leukocytes to sites of inflammation, they also exhibit a pronounced blood monocytopenia because their monocytes are retained in the BM (29). Furthermore, CXCL1/CXCR2 signaling also contributes to blood monocytosis. In mice fed a high-cholesterol diet, CXCL1 neutralization dampens expansion of blood Ly6Chigh monocytes (31).
Monocytes/macrophages in the post-MI phase
The early inflammatory phase is then succeeded by a reparative phase (starting on day 3 after MI) (32). Here, macrophages functionally shift from promoting inflammation to supporting repair and produce growth factors that recruit and activate mesenchymal reparative cells (mainly myofibroblasts and vascular cells) (33). This transitioning from inflammatory to reparative macrophages resembles in vitro polarization from the so-called “M1” to “M2” macrophages. After ingesting dead cells (a process called efferocytosis) macrophages decrease production of pro-inflammatory cytokines, such as IL-1β and TNFα, and increase production of anti-inflammatory and pro-fibrotic cytokines, such as IL-10 and transforming growth factor-β (TGFβ) (34,35). This indicates that efferocytosis may cause a shift in macrophage activation toward M2-like cells (36). However, macrophages display a high plasticity and can rapidly adapt their phenotype to different stimuli. Hence, the classic M1/M2 macrophage characterization, which originates from in vitro studies, most likely does not reflect phenotypic changes macrophage undergo in the infarct and needs careful re-evaluation (37).
The question arises whether macrophage phenotype/function is solely dictated by the local environment that surrounds these cells or also depends on their origin/ontogeny (resident vs. blood monocyte derived macrophages) (32). A recent study using fate mapping experiments revealed that macrophages from different origins in heart failure mice also display distinct phenotypes (3). Although it was not evaluated whether macrophages from varying sources reside in distinct locations in the heart, and are consequently embedded in different environments.
Ly6Clow macrophages facilitate wound healing and regeneration by (I) promoting transdifferentiation of fibroblasts into secretory and contractile myofibroblast (α-smooth muscle actin-positive fibroblast that are the major source of collagen for extracellular matrix deposition) through TGFβ secretion; (II) promoting angiogenesis through VEGF secretion (33); and (III) altering the extracellular matrix built-up/break-down through regulating the balance of matrix metalloproteinases (MMPs) and their inhibitors (tissue inhibitors of MMPs). Subsequently, the infarct matures and a collagen-based scar is formed. Depletion of monocytes/macrophages impairs scar formation by attenuating collagen deposition and angiogenesis (33).
Although the inflammatory phase is essential for removal of dead cell and debris, a timely repression of the inflammatory response is critical for effective healing. Exacerbated and prolonged inflammation may impede the healing process, as it was shown in infarcted mice with atherosclerosis that displayed adverse cardiac remodeling and blood monocytosis (38). Accordingly, elevated blood monocyte counts in patients after MI negatively correlate with ejection fraction (39). Along these lines, humans and mice with heart failure also display a blood leukocytosis (3,40,41). In general, elevated white blood cell numbers are an independent risk factor for CAD (42-44) and more specifically, higher monocyte counts independently predict risk for atherosclerosis after adjustment for conventional risk factors (45-49).
Macrophages in post-MI heart failure
Ventricular remodeling (defined as alterations in size, shape, structure and physiology) of both the infarcted and non-infarcted (remote) myocardial segments is closely linked with the development of post-MI heart failure (4). Recently, the involvement of monocytes/macrophages in the development and progression of ischemic cardiomyopathy was described (3,50). It was reported that macrophages gradually expand within the remote myocardium during the course of heart failure, while macrophage numbers within the scar wane (Figure 1C) (3). This remote macrophage expansion is caused by (I) increased local proliferation and (II) increased blood monocyte recruitment. It was reported that mechanical strain can stimulate macrophage proliferation via activating the MAPK pathway, indicating that higher mechanical strain in the failing heart may promote local macrophage proliferation. Furthermore, in HFrEF mice an increased sympathetic tone elevates systemic myeloid cell production in the BM causing the observed blood leukocytosis. Recruited monocytes contribute about one third to the increase in total cardiac macrophages. Using RNAi nanoparticle technology, the authors reduced post-MI monocyte recruitment to the remote myocardium, which attenuated adverse left ventricular remodeling. These data provide evidence that inflammatory BM derived cardiac macrophages augment adverse post-MI heart remodeling.
Conclusions
The post-MI phase is characterized by an early inflammatory stage where dead cells and debris are cleared from the infarct area and a subsequent reparative stage where fibrosis and angiogenesis occurs. Recent findings revealed the contribution of immune cells to cardiac injury and repair. Monocyte/macrophages are highly plastic cells that can rapidly adapt their phenotype/function and hence contribute differently to each stage of the disease. During the inflammatory phase they phagocytose necrotic cells and fuel inflammation by releasing proteases, pro-inflammatory cytokines/chemokines, and reactive oxygen species. In the reparative phase macrophages undergo transformation and exert pro-fibrotic functions by promoting collagen production and neovascularization. A timely resolution of each phase is crucial since an overzealous and prolonged inflammation and/or fibrosis may be deleterious and determines whether progression to heart failure or repair of cardiac tissue occurs. These experimental observations may pave the way for clinical studies that aim to target this biphasic response to improve outcomes following MI.
Acknowledgements
HB Sager and T Kessler were supported by the German Research Foundation in the context of the collaborative research center "Sonderforschungsbereich 1123 (B02)" and the German Center For Cardiovascular Research (DZHK rotation grants for medical doctors).
Footnote
Conflicts of Interest: The authors have no conflicts of interest to declare.
References
- Epelman S, Liu PP, Mann DL. Role of innate and adaptive immune mechanisms in cardiac injury and repair. Nat Rev Immunol 2015;15:117-29. [Crossref] [PubMed]
- Dutta P, Nahrendorf M. Monocytes in myocardial infarction. Arterioscler Thromb Vasc Biol 2015;35:1066-70. [Crossref] [PubMed]
- Sager HB, Hulsmans M, Lavine KJ, et al. Proliferation and Recruitment Contribute to Myocardial Macrophage Expansion in Chronic Heart Failure. Circ Res 2016;119:853-64. [Crossref] [PubMed]
- Hulsmans M, Sam F, Nahrendorf M. Monocyte and macrophage contributions to cardiac remodeling. J Mol Cell Cardiol 2016;93:149-55. [Crossref] [PubMed]
- Epelman S, Lavine KJ, Beaudin AE, et al. Embryonic and adult-derived resident cardiac macrophages are maintained through distinct mechanisms at steady state and during inflammation. Immunity 2014;40:91-104. [Crossref] [PubMed]
- Molawi K, Wolf Y, Kandalla PK, et al. Progressive replacement of embryo-derived cardiac macrophages with age. J Exp Med 2014;211:2151-8. [Crossref] [PubMed]
- Pinto AR, Godwin JW, Rosenthal NA. Macrophages in cardiac homeostasis, injury responses and progenitor cell mobilisation. Stem Cell Res 2014;13:705-14. [Crossref] [PubMed]
- Nahrendorf M, Swirski FK, Aikawa E, et al. The healing myocardium sequentially mobilizes two monocyte subsets with divergent and complementary functions. J Exp Med 2007;204:3037-47. [Crossref] [PubMed]
- Heidt T, Courties G, Dutta P, et al. Differential contribution of monocytes to heart macrophages in steady-state and after myocardial infarction. Circ Res 2014;115:284-95. [Crossref] [PubMed]
- Sager HB, Nahrendorf M. Inflammation: a trigger for acute coronary syndrome. Q J Nucl Med Mol Imaging 2016;60:185-93. [PubMed]
- Auffray C, Sieweke MH, Geissmann F. Blood monocytes: development, heterogeneity, and relationship with dendritic cells. Annu Rev Immunol 2009;27:669-92. [Crossref] [PubMed]
- Dewald O, Zymek P, Winkelmann K, et al. CCL2/Monocyte Chemoattractant Protein-1 regulates inflammatory responses critical to healing myocardial infarcts. Circ Res 2005;96:881-9. [Crossref] [PubMed]
- Sager HB, Dutta P, Dahlman JE, et al. RNAi targeting multiple cell adhesion molecules reduces immune cell recruitment and vascular inflammation after myocardial infarction. Sci Transl Med 2016;8:342ra80. [Crossref] [PubMed]
- Auffray C, Fogg D, Garfa M, et al. Monitoring of blood vessels and tissues by a population of monocytes with patrolling behavior. Science 2007;317:666-70. [Crossref] [PubMed]
- Carlin LM, Stamatiades EG, Auffray C, et al. Nr4a1-dependent Ly6C(low) monocytes monitor endothelial cells and orchestrate their disposal. Cell 2013;153:362-75. [Crossref] [PubMed]
- Alard JE, Ortega-Gomez A, Wichapong K, et al. Recruitment of classical monocytes can be inhibited by disturbing heteromers of neutrophil HNP1 and platelet CCL5. Sci Transl Med 2015;7:317ra196. [Crossref] [PubMed]
- Yona S, Kim KW, Wolf Y, et al. Fate mapping reveals origins and dynamics of monocytes and tissue macrophages under homeostasis. Immunity 2013;38:79-91. [Crossref] [PubMed]
- Hilgendorf I, Gerhardt LM, Tan TC, et al. Ly-6Chigh monocytes depend on Nr4a1 to balance both inflammatory and reparative phases in the infarcted myocardium. Circ Res 2014;114:1611-22. [Crossref] [PubMed]
- Gerhardt T, Ley K. Monocyte trafficking across the vessel wall. Cardiovasc Res 2015;107:321-30. [Crossref] [PubMed]
- Swirski FK, Nahrendorf M, Etzrodt M, et al. Identification of splenic reservoir monocytes and their deployment to inflammatory sites. Science 2009;325:612-6. [Crossref] [PubMed]
- Leuschner F, Panizzi P, Chico-Calero I, et al. Angiotensin-converting enzyme inhibition prevents the release of monocytes from their splenic reservoir in mice with myocardial infarction. Circ Res 2010;107:1364-73. [Crossref] [PubMed]
- Leuschner F, Rauch PJ, Ueno T, et al. Rapid monocyte kinetics in acute myocardial infarction are sustained by extramedullary monocytopoiesis. J Exp Med 2012;209:123-37. [Crossref] [PubMed]
- Swedberg K, Held P, Kjekshus J, et al. Effects of the early administration of enalapril on mortality in patients with acute myocardial infarction. Results of the Cooperative New Scandinavian Enalapril Survival Study II (CONSENSUS II). N Engl J Med 1992;327:678-84. [Crossref] [PubMed]
- Dutta P, Courties G, Wei Y, et al. Myocardial infarction accelerates atherosclerosis. Nature 2012;487:325-9. [Crossref] [PubMed]
- Varol C, Mildner A, Jung S. Macrophages: development and tissue specialization. Annu Rev Immunol 2015;33:643-75. [Crossref] [PubMed]
- Dutta P, Sager HB, Stengel KR, et al. Myocardial Infarction Activates CCR2(+) Hematopoietic Stem and Progenitor Cells. Cell Stem Cell 2015;16:477-87. [Crossref] [PubMed]
- Sager HB, Heidt T, Hulsmans M, et al. Targeting Interleukin-1beta Reduces Leukocyte Production After Acute Myocardial Infarction. Circulation 2015;132:1880-90. [PubMed]
- Serbina NV, Pamer EG. Monocyte emigration from bone marrow during bacterial infection requires signals mediated by chemokine receptor CCR2. Nat Immunol 2006;7:311-7. [Crossref] [PubMed]
- Tsou CL, Peters W, Si Y, et al. Critical roles for CCR2 and MCP-3 in monocyte mobilization from bone marrow and recruitment to inflammatory sites. J Clin Invest 2007;117:902-9. [Crossref] [PubMed]
- Shi C, Pamer EG. Monocyte recruitment during infection and inflammation. Nat Rev Immunol 2011;11:762-74. [Crossref] [PubMed]
- Drechsler M, Duchene J, Soehnlein O. Chemokines control mobilization, recruitment, and fate of monocytes in atherosclerosis. Arterioscler Thromb Vasc Biol 2015;35:1050-5. [Crossref] [PubMed]
- Swirski FK, Robbins CS, Nahrendorf M. Development and Function of Arterial and Cardiac Macrophages. Trends Immunol 2016;37:32-40. [Crossref] [PubMed]
- Frangogiannis NG. The inflammatory response in myocardial injury, repair, and remodelling. Nat Rev Cardiol 2014;11:255-65. [Crossref] [PubMed]
- Fadok VA, Bratton DL, Konowal A, et al. Macrophages that have ingested apoptotic cells in vitro inhibit proinflammatory cytokine production through autocrine/paracrine mechanisms involving TGF-beta, PGE2, and PAF. J Clin Invest 1998;101:890-8. [Crossref] [PubMed]
- Wan E, Yeap XY, Dehn S, et al. Enhanced efferocytosis of apoptotic cardiomyocytes through myeloid-epithelial-reproductive tyrosine kinase links acute inflammation resolution to cardiac repair after infarction. Circ Res 2013;113:1004-12. [Crossref] [PubMed]
- Howangyin KY, Zlatanova I, Pinto C, et al. Myeloid-Epithelial-Reproductive Receptor Tyrosine Kinase and Milk Fat Globule Epidermal Growth Factor 8 Coordinately Improve Remodeling After Myocardial Infarction via Local Delivery of Vascular Endothelial Growth Factor. Circulation 2016;133:826-39. [Crossref] [PubMed]
- Nahrendorf M, Swirski FK. Abandoning M1/M2 for a Network Model of Macrophage Function. Circ Res 2016;119:414-7. [Crossref] [PubMed]
- Panizzi P, Swirski FK, Figueiredo JL, et al. Impaired infarct healing in atherosclerotic mice with Ly-6C(hi) monocytosis. J Am Coll Cardiol 2010;55:1629-38. [Crossref] [PubMed]
- Maekawa Y, Anzai T, Yoshikawa T, et al. Prognostic significance of peripheral monocytosis after reperfused acute myocardial infarction:a possible role for left ventricular remodeling. J Am Coll Cardiol 2002;39:241-6. [Crossref] [PubMed]
- Engström G, Melander O, Hedblad B. Leukocyte count and incidence of hospitalizations due to heart failure. Circ Heart Fail 2009;2:217-22. [Crossref] [PubMed]
- Dixon DL, Griggs KM, Bersten AD, et al. Systemic inflammation and cell activation reflects morbidity in chronic heart failure. Cytokine 2011;56:593-9. [Crossref] [PubMed]
- Danesh J, Collins R, Appleby P, et al. Association of fibrinogen, C-reactive protein, albumin, or leukocyte count with coronary heart disease: meta-analyses of prospective studies. JAMA 1998;279:1477-82. [Crossref] [PubMed]
- Coller BS. Leukocytosis and ischemic vascular disease morbidity and mortality: is it time to intervene? Arterioscler Thromb Vasc Biol 2005;25:658-70. [Crossref] [PubMed]
- Wheeler JG, Mussolino ME, Gillum RF, et al. Associations between differential leucocyte count and incident coronary heart disease: 1764 incident cases from seven prospective studies of 30,374 individuals. Eur Heart J 2004;25:1287-92. [Crossref] [PubMed]
- Chapman CM, Beilby JP, McQuillan BM, et al. Monocyte count, but not C-reactive protein or interleukin-6, is an independent risk marker for subclinical carotid atherosclerosis. Stroke 2004;35:1619-24. [Crossref] [PubMed]
- Johnsen SH, Fosse E, Joakimsen O, et al. Monocyte count is a predictor of novel plaque formation: a 7-year follow-up study of 2610 persons without carotid plaque at baseline the Tromso Study. Stroke 2005;36:715-9. [Crossref] [PubMed]
- Nasir K, Guallar E, Navas-Acien A, et al. Relationship of monocyte count and peripheral arterial disease: results from the National Health and Nutrition Examination Survey 1999-2002. Arterioscler Thromb Vasc Biol 2005;25:1966-71. [Crossref] [PubMed]
- Friedman GD, Klatsky AL, Siegelaub AB. The leukocyte count as a predictor of myocardial infarction. N Engl J Med 1974;290:1275-8. [Crossref] [PubMed]
- Olivares R, Ducimetiere P, Claude JR. Monocyte count: a risk factor for coronary heart disease. Am J Epidemiol 1993;137:49-53. [Crossref] [PubMed]
- Ismahil MA, Hamid T, Bansal SS, et al. Remodeling of the mononuclear phagocyte network underlies chronic inflammation and disease progression in heart failure: critical importance of the cardiosplenic axis. Circ Res 2014;114:266-82. [Crossref] [PubMed]