Surgical technique: establishing a pre-clinical large animal model to test aortic valve leaflet substitute
Introduction
Substantial progress has been achieved in treating congenital cardiovascular diseases due to better diagnostics, advance surgical techniques and improvement in postoperative care. Nevertheless, the ultimate goal is to achieve a physiologic and durable repair that has the potential to grow, which has not been attained yet due to the limitations of all the tissue substitutes used. Therefore, there remains the need for surgical re-intervention through the patient’s lifetime.
Aortic valve patients suffering from symptomatic aortic stenosis or insufficiency have limited life expectancy (1). Degenerative valve disease has become the most frequent disease leading to aortic valve replacement (AVR) in adults, which has been established as a standard treatment but implies prosthesis-related complications. Generally, the replacement of diseased valves is done by either mechanical prosthesis or bioprosthetic tissue valves. Mechanical valves need anticoagulation, which is contraindicated in the young frequently (relatively), either for planned pregnancy or because their lifestyle is very active. All biological valves are at risk for calcification and structural valve degeneration (SVD), leading to reoperation. The age of the patient at the time of valve implantation was shown to be the most important determinant of SVD (2); besides, it has been demonstrated that neonates and infants undergoing AVR are a high-risk group for mortality (3). All the valve prostheses used have the disadvantage in that they are unable to grow, repair or remodel, and are thrombogenic and pose an increased risk for endocarditis. Techniques like the Ross principle or homograft implantation have been introduced, especially in children and adolescents. Unfortunately, the rate of SVD for homograft—around 30% after 10 years and 59% after 15 years—is similar to bioprosthetic valves (2). Both the Ross principle and homograft do not have the ability to grow, as is expected of an ideal valve substitute, alongside hemodynamic function as the original valve, non-thrombogenic, no antigenicity and no structural degeneration. In contrast to older patients suffering from degenerative valve disease, young adults and children face the risk of either multiple reoperations or the side effect of lifelong anticoagulation. The lack of an ideal valve substitute has raised interest in alternative techniques, such as repairing the diseased valve rather than replacing it. In the past several years, the percentage of valve repair as compared to AVR has increased (1). For many of the aortic valve repair techniques, a patch is needed as substitute, partial or complete replacement or leaflet extension. So far various cusp tissue substitutes have been tested, but failed to fulfill the requirements for an optimal patch material.
Currently used patch material for valve repair
An optimal patch material should be pliable, hemostatic and resistant to tearing, should not shrink or calcify, and should possibly not induce an inflammatory cascade. Since the late 1960s, attempts have been made to use biologic materials like fascia lata, dura mater and bovine pericardium with poor outcomes (4-6). An easily available source for valve repair is the autologous pericardium, which is widely used for partial or total cusp replacement or leaflet extension (7-10). Some groups propagate pretreatment with glutaraldehyde, which prevents secondary shrinking and calcification (9,10). On the other hand, untreated fresh autologous pericardium might possess true growth, but distensibility as well. Despite good short- and mid-term results, it is associated with a high degeneration rate in the long term (11-14) and is associated with bacterial endocarditis (8,10,15). Bovine pericardium—used as leaflet extension—has been shown to be less effective as compared to autologous pericardium (16,17), and autologous pericardium has greater resistance to retraction. Since polytetrafluoroethylene (PTFE) was successfully used for right ventricular outflow graft reconstruction or in mitral leaflet position with good long-term results (18-20), it was applied as leaflet extension in the aortic valve position. Nosál' and coworkers reported 13 congenitally affected patients, with the youngest being 22 months old, in whom PTFE leaflet extensions were done with a follow-up till 30 months (21). However, there still remains a higher risk of endocarditis, and concerns have been raised about leaflet thickening and decreased mobility (18,22). Technical and scientific achievements in the field of tissue engineering (TE) may offer new perspectives with the potential of an off-the-shelf starter matrix for guided tissue regeneration.
Study rationale
The ultimate goal is to develop an aortic valve leaflet substitute which is physiological, durable and has the potential for growth, and test it in a chronic animal model. Our research group developed an off-the-shelf decellularized TE patch (TEP) as an aortic valve leaflet substitute (23,24). In a step-by-step approach, we want to establish an animal model as proof for feasibility, and, in a further step, we plan to use the chronic animal model as proof of concept.
For decades, different animal models have been a central critical component in the pre-clinical safety evaluation of cardiovascular devices developed for use in humans (25).
Here, we describe our technique of implantation of this decellularized TEP as an aortic valve leaflet substitute in a pre-clinical large animal model in orthotropic position.
Operative technique
We operated on adult Swiss White Mountain sheep (59–65 kg). All the surgical procedures and postoperative care were carried out in accordance with the approved protocol by the Ethics Committee (approval no. 19/2013) of the Canton (state) of Zurich. During the course of the animal experiments, all the animals received humane care in accordance with the Guide for Care and Use of Laboratory Animals (Publication no. 85-23. Bethesda, MD: National Institutes of Health, 1985) as well as with the “Principles of Laboratory Animal-Care”.
After general anesthesia and preoperative preparation (invasive blood pressure lines femoral artery and upper body half (ear), venous lines, bladder catheter,) either median sternotomy (n: 6) or right lateral thoracotomy (RLT; n: 4) using 4th intercostal space, without removing a rib, was carried out. The pericardium was opened by protecting the phrenic nerve and stay sutures were placed. For cardiopulmonary bypass (CPB), 10,000 IU of Heparin was given and the activated clotting time (ACT) levels above 300 seconds were maintained throughout the CPB. Arterial cannulation was done via the aortic arch (n: 3) or truncus brachiocephalicus (n: 4) using a 21-F cannula, which allows adequate arterial inflow. Venous cannulation via the right atrium using a single stage 36-F cannula was carried out. A pulmonary VENT catheter was placed with continuous suction (150 mL/minute). Temperature monitoring was done with a rectal probe and mild hypothermia (32 degree Celsius) was sought for the intervention. Calafiore blood cardioplegia was given over the aortic root, followed by aortotomy. The aortic valve was exposed by using three stay sutures just above the upper edge of the commissures. The non-coronary leaflet was completely explanted (see Figure 1) and used as a pattern for patch tailoring. The TEP was textured to a mild triangular shape of the explanted leaflet by adding 3mm of extra patch material, as compared to the explanted leaflet (see Figure 2). The substitute was implanted using six mattress 6-0 polypropylene sutures following the origin of the explant line (see Figure 3). The last sutures on each side ended with the upper edge of the commissures. The aim was equal distribution on each side of the created base of the patch to achieve good geometry (see Figure 4).
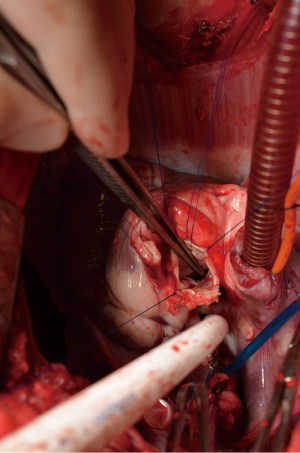
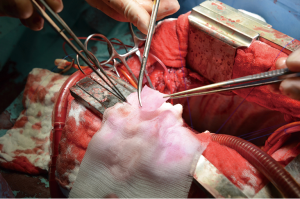
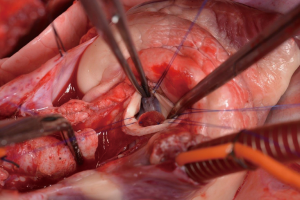
After testing the valve function and successful weaning from CPB, one-third of the full Protamine dose was administered. After chest closure, echocardiography was done followed by computer tomography (CT). Finally, the sheep were sacrificed, and the whole aortic root was explanted (see Figure 5) and the TEP was analyzed.
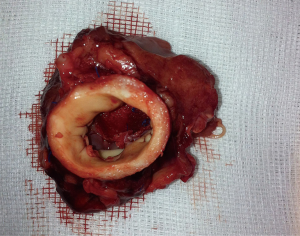
Using this technique, the patch was implanted in nine sheep. The operation was completed successfully in all the nine attempts. The average aortic cross-clamp time was 96 minutes, with the average bypass time over 140 minutes. Postoperative echocardiographic and CT studies (see Figure 6) revealed good co-adaptation of the leaflets (see Figure 7) without signs of relevant aortic valve stenosis or regurgitation. After post-operative diagnostic (echocardiography and CT), the animals were sacrificed and the TEP was analyzed. The analyses showed no tears within the patch due to systemic blood pressure. Histologic analyses showed infiltration of blood cells into the TEP (see Figure 8).
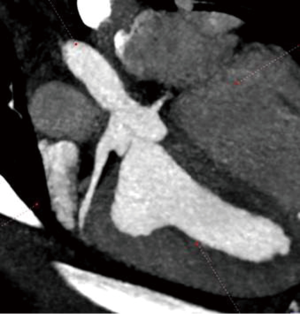
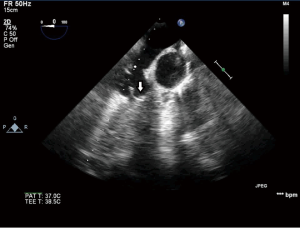
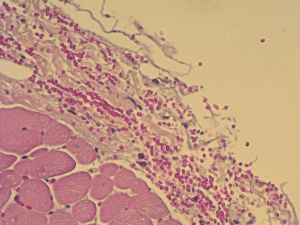
Only the last sheep of the series was kept alive for 24 hours to test feasibility for translation to chronic phase trial.
Comments
There are increasing numbers of scientific publications on the use of animal models in cardiovascular disease research. The advantages of sheep are that they have similarities with humans in the molecular basis of cardiac contraction and in coronary anatomy. Likewise, the physiology is comparable to humans; especially when testing a TEP in the high pressure system, the mean arterial blood pressure in sheep seems to be of approximately 100 mmHg (26). Finally, calcification as one of the main outcomes might occur very early, as one year in a human is equivalent to seven years in a sheep. From our experience and set-up, we would like to share some crucial issues:
(I) Surgical access: we started with median sternotomy, with the idea that this approach provides the best exposure to the heart; however, the potential benefits of a thoracotomy outweighed those of sternotomy, especially when considering a chronic study. We believe that a thoracotomy leads to decreased morbidity, especially postoperative pain, as compared to that associated with sternotomy. In terms of early extubation and postoperative breathing (as the animals lay on the sternum), thoracotomy is beneficial in comparison to sternotomy. According to our experience, an RLT using the fourth intercostal space offered good exposure to the aorta and truncus brachiocephalicus as well as good access to the aortic valve. Arterial cannulation is easy to achieve using this surgical access. If one chooses to use a left lateral thoracotomy (LLT), which is possible, one has to dissect the main pulmonary artery to get sufficient access to the ascending aorta and the aortic valve.
(II) CPB and cardioplegia: there might be several possibilities to perform arterial cannulation. While we tried to avoid femoral cannulation (lines for arterial blood pressure measurement and venous lines; higher infection risk), one of the shortcomings of right-sided thoracotomy is that one will not have good access to the descending aorta, which is a good option to cannulate when using an LLT. In contrast to humans, where the ascending aorta is the primary target, this part of the aorta in sheep is short, and we tried to preserve the whole ascending aorta for application of cardioplegia (via root) and the cross clamp as well as left enough material for the closure of the ascending aorta. Alternatively, we chose the truncus brachiocephalicus, which is easy to access from RLT, for arterial cannulation.
The mainstays of cardio-protection during cardiac surgery are cardioplegia, hypothermia, and prevention of cardiac distention. We always use antegrade cardioplegia with cannulation of the aortic root as this (still) might be the best way of cardiac protection through the strenuous CPB process. We did not actively cool the animals, but allowed a passive cooling down to 32 degree Celsius. To avoid cardiac distention, a good venous drainage for CPB is essential; and also the placement of the VENT catheter in the main pulmonary artery, which is easy to access and has low bleeding complications after removal might help.
(III) Technique of patch augmentation and implantation: the advantages of using the non-coronary leaflet seem to be obvious, as the risk of complications involving the coronary arteries are much less. We believe that in order to prove the hypothesis of cell immigration into the patch and transformation of the TEP into native leaflet tissue, one should cut out the whole leaflet. The explanted leaflet might give an idea about how big the substitute should be; nevertheless, we decided to add some additional space to the TEP to have a sufficient rim for sutures and a sufficient coaptation height. On the other hand, one has to be careful against adding too much patch material, as this might interfere with the function of the two remaining leaflets. The use of mattress suture allows a more clear view and better placement of the sutures as compared to a running suture; the thorax of sheep is deep (see Figures 1,2,3,4)!
Our experiences and comments may help other groups using a sheep model in cardiovascular disease research to further increase animal welfare by improving their rates of survival.
Acknowledgements
None.
Footnote
Conflicts of Interest: The authors have no conflicts of interest to declare.
References
- Vahanian A, Alfieri O, Andreotti F, et al. Guidelines on the management of valvular heart disease (version 2012): the Joint Task Force on the Management of Valvular Heart Disease of the European Society of Cardiology (ESC) and the European Association for Cardio-Thoracic Surgery (EACTS). Eur J Cardiothorac Surg 2012;42:S1-44. [Crossref] [PubMed]
- Rahimtoola SH. Choice of prosthetic heart valve in adults an update. J Am Coll Cardiol 2010;55:2413-26. [Crossref] [PubMed]
- Woods RK, Pasquali SK, Jacobs ML, et al. Aortic valve replacement in neonates and infants: an analysis of the Society of Thoracic Surgeons Congenital Heart Surgery Database. J Thorac Cardiovasc Surg 2012;144:1084-89. [Crossref] [PubMed]
- Senning A. Aortic valve replacement by fascia lata. Verh Dtsch Ges Kreislaufforsch 1970;36:33-8. [Crossref] [PubMed]
- Stolf NA, Puig LB, Zerbini EJ. Late results of replacement of cardiac valves by dura-mater allografts. Int J Artif Organs 1980;3:104-7. [PubMed]
- Al-Halees Z, Gometza B, Duran CM. Aortic valve repair with bovine pericardium. Ann Thorac Surg 1998;65:601-2. [PubMed]
- Lausberg HF, Aicher D, Langer F, et al. Aortic valve repair with autologous pericardial patch. Eur J Cardiothorac Surg 2006;30:244-9. [Crossref] [PubMed]
- Al Halees Z, Al Shahid M, Al Sanei A, et al. Up to 16 years follow-up of aortic valve reconstruction with pericardium: a stentless readily available cheap valve? Eur J Cardiothorac Surg 2005;28:200-5; discussion 205. [Crossref] [PubMed]
- El Khoury G, Glineur D, Rubay J, et al. Functional classification of aortic root/valve abnormalities and their correlation with etiologies and surgical procedures. Curr Opin Cardiol 2005;20:115-21. [Crossref] [PubMed]
- Grinda JM, Latremouille C, Berrebi AJ, et al. Aortic cusp extension valvuloplasty for rheumatic aortic valve disease: midterm results. Ann Thorac Surg 2002;74:438-43. [Crossref] [PubMed]
- McMullan DM, Oppido G, Davies B, et al. Surgical strategy for the bicuspid aortic valve: tricuspidization with cusp extension versus pulmonary autograft. J Thorac Cardiovasc Surg 2007;134:90-8. [Crossref] [PubMed]
- Alsoufi B, Karamlou T, Bradley T, et al. Short and midterm results of aortic valve cusp extension in the treatment of children with congenital aortic valve disease. Ann Thorac Surg 2006;82:1292-9; discussion 1300. [Crossref] [PubMed]
- De La Zerda DJ, Cohen O, et al. Aortic valve-sparing repair with autologous pericardial leaflet extension has a greater early re-operation rate in congenital versus acquired valve disease. Eur J Cardiothorac Surg 2007;31:256-60. [Crossref] [PubMed]
- Odim J, Laks H, Allada V, et al. Results of aortic valve-sparing and restoration with autologous pericardial leaflet extensions in congenital heart disease. Ann Thorac Surg 2005;80:647-53; discussion 653-4. [Crossref] [PubMed]
- Jeong DS, Kim KH, Ahn H. Long-term results of the leaflet extension technique in aortic regurgitation: thirteen years of experience in a single center. Ann Thorac Surg 2009;88:83-9. [Crossref] [PubMed]
- Duran CM, Gallo R, Kumar N. Aortic valve replacement with autologous pericardium: surgical technique. J Card Surg 1995;10:1-9. [Crossref] [PubMed]
- Chauvaud S, Jebara V, Chachques JC, et al. Valve extension with glutaraldehyde-preserved autologous pericardium. Results in mitral valve repair. J Thorac Cardiovasc Surg 1991;102:171-7; discussion 177-8. [PubMed]
- Ito T, Maekawa A, Yamana K, et al. Use of an expanded polytetrafluoroethylene patch as an artificial leaflet in mitral valve plasty: an early experience. Ann Thorac Surg 2010;89:1620-4. [Crossref] [PubMed]
- Brown JW, Ruzmetov M, Vijay P, et al. Right ventricular outflow tract reconstruction with a polytetrafluoroethylene monocusp valve: a twelve-year experience. J Thorac Cardiovasc Surg 2007;133:1336-43. [Crossref] [PubMed]
- Ando M, Takahashi Y. Ten-year experience with handmade trileaflet polytetrafluoroethylene valved conduit used for pulmonary reconstruction. J Thorac Cardiovasc Surg 2009;137:124-31. [Crossref] [PubMed]
- Nosál' M, Poruban R, Valentík P, et al. Initial experience with polytetrafluoroethylene leaflet extensions for aortic valve repair. Eur J Cardiothorac Surg 2012;41:1255-7; discussion 1258. [Crossref] [PubMed]
- Quintessenza JA, Jacobs JP, Morell VO, et al. Initial experience with a bicuspid polytetrafluoroethylene pulmonary valve in 41 children and adults: a new option for right ventricular outflow tract reconstruction. Ann Thorac Surg 2005;79:924-31. [Crossref] [PubMed]
- Weber B, Dijkman PE, Scherman J, et al. Off-the-shelf human decellularized tissue-engineered heart valves in a non-human primate model. Biomaterials 2013;34:7269-80. [Crossref] [PubMed]
- Hoerstrup SP, Cummings Mrcs I, Lachat M, et al. Functional growth in tissue-engineered living, vascular grafts: follow-up at 100 weeks in a large animal model. Circulation 2006;114:I159-66. [Crossref] [PubMed]
- Gallegos RP, Nockel PJ, Rivard AL, et al. The current state of in-vivo pre-clinical animal models for heart valve evaluation. J Heart Valve Dis 2005;14:423-32. [PubMed]
- DiVincenti L Jr, Westcott R, Lee C. Sheep (Ovis aries) as a model for cardiovascular surgery and management before, during, and after cardiopulmonary bypass. J Am Assoc Lab Anim Sci 2014;53:439-48. [PubMed]