MicroRNAs are novel non-invasive diagnostic biomarkers for pulmonary embolism: a meta-analysis
Introduction
Pulmonary embolism (PE), as a common type of life-threatening venous thromboembolism, is the third commonest cause of cardiovascular death after myocardial infarction and stroke with high morbidity and mortality (1,2). Because of its variable manifestation and poor sensitivity as well as specificity of signs and symptoms, rapid and accurate diagnosis of PE remains challenging (3). Recent diagnostic methods for PE mainly consist of biomarkers (such as D-dimer) and radiologic image examinations such as computed tomography angiography and ventilation-perfusion scintigraphy (3,4). However, as a widely applied biomarker for the diagnosis of PE, the D-dimer test exhibits high sensitivity, but low specificity for the diagnosis of PE (5). Therefore, a negative result of D-dimer test was commonly used for exclusion of PE (3,6). Moreover, the diagnostic accuracy of D-dimer test was greatly influenced by various pathophysiologic statuses such as age (7), pregnancy (8), and surgery (9). While for those radiologic image examinations, radiation exposure was the main concern for daily application (10). Therefore, novel non-invasive biomarker with improved diagnostic accuracy would significantly facilitate the diagnosis of PE.
MicroRNAs (miRNAs) are endogenous small (about 22 nucleotides in length) noncoding RNAs that plays important regulatory roles by targeting mRNAs for cleavage or translational repression (11). Recent studies have found that miRNAs play crucial roles in many cellular processes, such as development, proliferation, differentiation and apoptosis (11). Due to its high stability in plasma or serum and potential for highly sensitive measurement, circulating miRNAs have been intensively investigated as noninvasive biomarker for diseases (12), such as cancers (13,14), neurodegenerative diseases (15) and cardiovascular diseases (16). Recent studies have also explored the biomarker value of miRNAs in the diagnosis of PE. Xiao et al. (17) firstly explored the diagnostic value of elevated plasma miR-134 in PE, indicating that plasma miR-134 could be an important biomarker for the diagnosis of PE. Since then, other studies (18,19) also demonstrated the diagnostic biomarker role of variable miRNAs for PE diagnosis. However, all those studies were limited to a small sample size. Therefore, the actual diagnostic power of miRNAs in PE is still unclear. In our current study, we try to conduct a diagnostic meta-analysis to pool all those results of relevant studies exploring the diagnostic value of miRNAs in PE patients to establish the potential diagnostic power of miRNAs in PE. To our knowledge, our study is the first meta-analysis to explore the biomarker value of miRNAs in the diagnosis of PE.
Methods
Search strategies
We performed systematic computerized searches of the PubMed, Embase for reports dated up to July 22, 2016. We used the following search terms: “microRNA or miRNA or miR or RNA” AND “embolism”. All reference lists from the studies selected by electronic searching were scanned to identify other relevant studies.
Study selection
The following criteria were used for study inclusion: (I) studies explored the diagnostic value of miRNAs for PE diagnosis; (II) sufficient data could be obtained for calculating sensitivity and specificity of each study. The exclusion criteria were as follows: (I) papers without any relevant data that could be extracted for analysis; (II) papers that were not published in English; and (III) case reports, abstracts, conference reports, and reviews.
Data extraction and quality assessment
Two authors (Han-Yu Deng and Gang Li) extracted the data independently from each included study. To avoid bias, discrepancies were resolved by adjudication by a third author (Jun Luo). Data were carefully retrieved from full articles using a standardized data collection form. The following data were collected from each study: first author’s name, year of publication, number patients, specimen type, miRNAs type, miRNAs expression level, and cutoff values. The outcome variables including true-positive (TP), false-positive (FP), false-negative (FN), and true-negative (TN) results were extracted from each study. The quality assessment of each included study was conducted by the quality assessment of diagnostic accuracy studies-2 (QUADAS-2) (20), which consists of four domains: patient selection, index test, reference standard, and flow and timing. When a criterion was completed, an answer of Yes was given, No if a criterion was not fulfilled, and unclear if a criterion was unclear or not reported.
Statistical analysis
We used STATA software, version 12.0 (Stata Corporation, College Station, TX, USA), Meta-Disc statistical software version 1.4 and RevMan 5.3 (Cochrane Collaboration, Oxford, UK) to perform this meta-analysis. We analyzed diagnostic power of miRNAs in the diagnosis of PE by pooling sensitivity, specificity, positive likelihood ratio (PLR), negative likelihood ratio (NLR), diagnostic odds ratio (DOR) from each included study. Summary receiver operating characteristic (SROC) curves and the area under the curve (AUC) were also calculated. For each study, the between-study heterogeneity was assessed using χ2-based Q statistics and the I2 test. Random effects models were used because of the high heterogeneity of the studies (P<0.1 or I2>50%). Otherwise, fixed effects models were used. To detect threshold effects, the relationship between sensitivity and specificity was evaluated by the Spearman correlation coefficient (21). Subgroup and sensitivity analyses were conducted to assess the potential sources of between-study heterogeneity. The publication bias was evaluated by using Deeks’ funnel plot (22). Statistical significance was set at P<0.05.
Results
Description of the included studies
A flow chart of our analyses is shown in Figure 1. Three studies (17-19) covering a total of 254 participants were included in our analysis. The main data extracted from these included studies are listed in Table 1. Two studies (17,18) only focused on one type of miRNAs, while one study (19) explored three types of miRNAs, and therefore, five data sets of miRNAs were analyzed in our current meta-analysis totally. The standard method for the diagnosis of PE was pulmonary angiography, contrast-enhanced computed tomography pulmonary angiography (CTPA) or a ventilation-perfusion lung scan, alone or in combination in all studies. Two studies (17,18) used plasma as specimen, while one study (19) used serum, and all those specimens were collected on the first day after admission. The expression of miRNAs were measured by the quantitative real-time reverse transcription PCR (qRT-PCR) method in all those included studies . All those miRNAs in our current analysis were up-regulated in PE patients compared with control individuals. The outcome variables including TP, FP, FN, and TN results extracted from each study were also listed in Table 1.
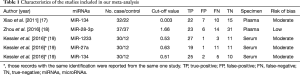
Full table
Quality assessment
We used the QUADAS-2 quality assessment to assess the quality of our included studies, the quality of which turned out to be generally good. However, potential risk of bias was observed in our meta-analysis (Figure 2).
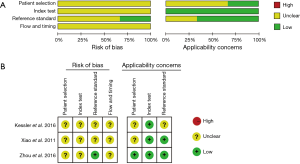
Diagnostic accuracy analysis
The forest plot of sensitivity and specificity for miRNAs in the diagnosis of PE was shown in Figure 3. Those pooled results of the diagnostic power of miRNAs in PE diagnosis with their corresponding 95% confidence intervals (CIs) are as follows: sensitivity, 0.83 (95% CI: 0.67–0.92); specificity, 0.85 (95% CI: 0.72–0.92); PLR, 5.40 (95% CI: 2.7–10.9); NLR, 0.20 (95%CI: 0.10–0.44); and DOR, 26.00 (95%CI: 7.00–101.00) (Table 2). Moreover, the SROC curve was showed in Figure 4, and the AUC was estimated to be 0.90 (95% CI: 0.87–0.92).
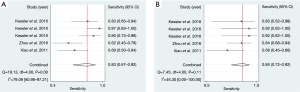
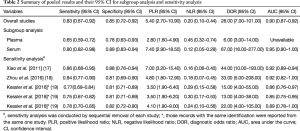
Full table
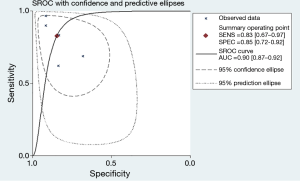
Subgroup analysis and sensitivity analysis
Our meta-analysis showed that the Chi-squared and I2 tests values of sensitivity and specificity were 19.12 (P<0.01), and 79.09%, 7.45 (P=0.11), and 46.3%, respectively, indicating significant heterogeneity among the included studies in the analysis of sensitivity. As we know, the threshold effect can be a major cause of heterogeneity in diagnostic meta-analysis. However, in this meta-analysis, we did not find the evidence that the heterogeneity was caused by the threshold effect, because the Spearman correlation coefficient was –0.67 with a P value of 0.22 (P>0.05). Due to the limited sample size, we did not conduct meta-regression analysis; we conducted subgroup analysis only based on the specimen type (plasma or serum) instead. The serum-based specimen seemed to show higher diagnostic accuracy than the plasma-based specimen, with sensitivity decreased from 0.90 to 0.65, specificity decreased from 0.89 to 0.78 (Table 2).
To evaluate the stability of our overall results, we performed sensitivity analysis by sequential removal of each study to explore the influence of the individual data set to overall results. Sensitivity analysis showed that our overall results were not obviously influenced by single study (Table 2).
Publication bias
We used Deeks’s funnel plot asymmetry test to conduct publication bias analysis, which showed a symmetric funnel plot (Figure 5), indicating that there was no significant publication bias among the studies included in our meta-analysis(Deeks’ test: P=0.13).
Discussion
PE is a frequent and life-threatening disease with significant morbidity and mortality (23). However, a timely and accurate diagnosis of PE remains challenging in our clinical practice (5). Although the diagnosis of PE was improved with the advent of D-dimer test and CT scan, there is still need for simple, reliable and non-invasive biomarker for accurate and rapid diagnosis of PE (17). Recent researches have focused more on miRNAs as non-invasive diagnostic biomarkers of various diseases, including PE. However, due to the limited number of studies and sample size available, the actual diagnostic power of miRNAs in the diagnosis of PE still remains unclear. Therefore, in this meta-analysis, we tried to pool the results of all those available studies to explore and establish the actual diagnostic power of miRNAs for PE.
Our meta-analysis found that miRNAs showed relatively high diagnostic performances with an AUC of 0.90, and that the sensitivity and specificity were 0.83 and 0.85, respectively. Moreover, the pooled DOR in our meta-analysis was 26. Therefore, all those above good results suggested that miRNAs are powerful diagnostic biomarkers for PE diagnosis. Even though the pooled results for PLR and NLR were not so encouraging, we still believe that miRNAs remains relatively high accuracy in the diagnosis of PE in terms of the pooled values of AUC and DOR. In our subgroup analysis, we found that the diagnostic power of miRNAs in the serum seemed to be higher than that of miRNAs in the plasma. Therefore, our meta-analysis proved that miRNAs could be potential diagnostic biomarker for PE, especially for the miRNAs in the serum. To our knowledge, this is the first meta-analysis to summarize the diagnostic power of miRNAs for PE diagnosis.
Currently, the diagnosis of PE was mainly based on the combination of blood tests and image examinations. D-dimer test was the most commonly used blood test for the diagnosis of PE. However, D-dimer test has a high sensitivity but low specificity (5), and therefore, a negative D-dimer test is widely considered to be good for “ruling out” PE (3). However, in some cases, such as patients in pregnancy (8) or undergoing arthroplasty (9), a negative D-dimer test seemed not to rule out PE safely in these patients. Image examinations such as CTPA, also have some limitations, for example radiation exposure, potential allergy to contrast material, and the risk of contrast-induced nephropathy (10,24). Therefore, there is a great need for simple and reliable methods for rapid and accurate diagnosis of PE. Our current meta-analysis showed the evidence for miRNAs as non-invasive diagnostic biomarkers for PE. Recent researches (25-27) have explored the detailed mechanisms between miRNAs and thrombosis, indicating that miRNAs play important roles in the pathological process of thrombosis. Therefore, with more and more evidence showing the relations between miRNAs and thrombosis, we believe that miRNAs could not only serve as a diagnostic biomarker but also a therapeutic target of PE.
Our meta-analysis has several limitations. First, our meta-analysis was conducted based on a limited sample size, which could influence our analytical power. Second, obvious heterogeneity was observed in the analysis of sensitivity. Even though we tried to explore possible factors causing the heterogeneity through meta-regression analysis and subgroup analysis, due to the limited number of the studies and sample size, we had no chance to perform meta-regression analysis and only conducted subgroup analysis based on the specimen type. However, we think the heterogeneity could be caused by the innate difference among various kinds of miRNAs as well as the cut-off value and sample size of each study. Third, due to the limited number of studies exploring the diagnostic value of miRNAs, all those included studies focused on different miRNAs, therefore we could not pool the diagnostic value of one specific miRNA across all studies and instead, we only pooled those various miRNAs together to generate a preliminary conclusion about the diagnostic value of miRNAs. Fourth, potential risk of bias was observed in our study. Finally, the diagnostic biomarker of miRNAs is still in the preliminary research stage, how these results could be applied in clinical practice and how to improve the overall diagnostic power of miRNAs (for example, the combination of miRNAs and D-dimer test for the diagnosis of PE) as well as how to measure miRNAs in serum or plasma more efficiently and easily would be problems for future studies. Therefore, more researches are needed to confirm and update and our conclusions about the diagnostic as well as therapeutic roles of miRNAs in PE.
Conclusions
MiRNAs could serve as novel non-invasive diagnostic biomarkers of PE with a relatively high diagnostic power. More researches, however, are needed to explore the diagnostic and therapeutic values of miRNAs for PE.
Acknowledgements
Funding: This work was supported by National Natural Science Foundation of China [No. 81672291; No. 31071210] (to Yi-Dan Lin).
Footnote
Conflicts of Interest: The authors have no conflicts of interest to declare.
References
- Lapner ST, Kearon C. Diagnosis and management of pulmonary embolism. BMJ 2013;346:f757. [Crossref] [PubMed]
- Monreal M, Mahé I, Bura-Riviere A, et al. Pulmonary embolism: Epidemiology and registries. Presse Med 2015;44:e377-83. [Crossref] [PubMed]
- Righini M, Robert-Ebadi H, Le Gal G. Diagnosis of pulmonary embolism. Presse Med 2015;44:e385-91. [Crossref] [PubMed]
- Kearon C. Diagnosis of pulmonary embolism. CMAJ 2003;168:183-94. [PubMed]
- Di Nisio M, van Es N, Buller HR. Deep vein thrombosis and pulmonary embolism. Lancet 2017;388:3060-3073. [Crossref] [PubMed]
- Stein PD, Hull RD, Patel KC, et al. D-dimer for the exclusion of acute venous thrombosis and pulmonary embolism: a systematic review. Ann Intern Med 2004;140:589-602. [Crossref] [PubMed]
- Woller SC, Stevens SM, Adams DM, et al. Assessment of the safety and efficiency of using an age-adjusted D-dimer threshold to exclude suspected pulmonary embolism. Chest 2014;146:1444-51. [Crossref] [PubMed]
- Rhead S, Ferguson C. Towards evidence based emergency medicine: Best BETs from the Manchester Royal Infirmary. BET 2: Current evidence does support the use of a negative D-dimer to rule out suspected pulmonary embolism in pregnancy. Emerg Med J 2014;31:946-7. [Crossref] [PubMed]
- Sugimoto E, Kuroda T, Fujita Y, et al. D-dimer testing cannot rule out thromboembolism after major lower extremity arthroplasties and thromboprophylaxis treatment. J Anesth 2015;29:686-9. [Crossref] [PubMed]
- Schembri GP, Miller AE, Smart R. Radiation dosimetry and safety issues in the investigation of pulmonary embolism. Semin Nucl Med 2010;40:442-54. [Crossref] [PubMed]
- Bartel DP. MicroRNAs: genomics, biogenesis, mechanism, and function. Cell 2004;116:281-97. [Crossref] [PubMed]
- Pritchard CC, Cheng HH, Tewari M. MicroRNA profiling: approaches and considerations. Nat Rev Genet 2012;13:358-69. [Crossref] [PubMed]
- Inamura K, Ishikawa Y. MicroRNA In Lung Cancer: Novel Biomarkers and Potential Tools for Treatment. J Clin Med 2016;5:E36. [Crossref] [PubMed]
- Harada K, Baba Y, Ishimoto T, et al. The role of microRNA in esophageal squamous cell carcinoma. J Gastroenterol 2016;51:520-30. [Crossref] [PubMed]
- Basak I, Patil KS, Alves G, et al. microRNAs as neuroregulators, biomarkers and therapeutic agents in neurodegenerative diseases. Cell Mol Life Sci 2016;73:811-27. [Crossref] [PubMed]
- Li M, Zhang J. Circulating MicroRNAs: Potential and Emerging Biomarkers for Diagnosis of Cardiovascular and Cerebrovascular Diseases. Biomed Res Int 2015;2015:730535.
- Xiao J, Jing ZC, Ellinor PT, et al. MicroRNA-134 as a potential plasma biomarker for the diagnosis of acute pulmonary embolism. J Transl Med 2011;9:159. [Crossref] [PubMed]
- Zhou X, Wen W, Shan X, et al. MiR-28-3p as a potential plasma marker in diagnosis of pulmonary embolism. Thromb Res 2016;138:91-5. [Crossref] [PubMed]
- Kessler T, Erdmann J, Vilne B, et al. Serum microRNA-1233 is a specific biomarker for diagnosing acute pulmonary embolism. J Transl Med 2016;14:120. [Crossref] [PubMed]
- Whiting PF, Rutjes AW, Westwood ME, et al. QUADAS-2: a revised tool for the quality assessment of diagnostic accuracy studies. Ann Intern Med 2011;155:529-36. [Crossref] [PubMed]
- Zamora J, Abraira V, Muriel A, et al. Meta-DiSc: a software for meta-analysis of test accuracy data. BMC Med Res Methodol 2006;6:31. [Crossref] [PubMed]
- Deeks JJ, Macaskill P, Irwig L. The performance of tests of publication bias and other sample size effects in systematic reviews of diagnostic test accuracy was assessed. J Clin Epidemiol 2005;58:882-93. [Crossref] [PubMed]
- Bertoletti L, Humbert M. Pulmonary embolism: An update. Presse Med 2015;44:e373-6. [Crossref] [PubMed]
- Remy-Jardin M, Pistolesi M, Goodman LR, et al. Management of suspected acute pulmonary embolism in the era of CT angiography: a statement from the Fleischner Society. Radiology 2007;245:315-29. [Crossref] [PubMed]
- Meng Q, Wang W, Yu X, et al. Upregulation of MicroRNA-126 Contributes to Endothelial Progenitor Cell Function in Deep Vein Thrombosis via Its Target PIK3R2. J Cell Biochem 2015;116:1613-23. [Crossref] [PubMed]
- Chen L, Wang J, Wang B, et al. MiR-126 inhibits vascular endothelial cell apoptosis through targeting PI3K/Akt signaling. Ann Hematol 2016;95:365-74. [Crossref] [PubMed]
- Gareri C, De Rosa S, Indolfi C. MicroRNAs for Restenosis and Thrombosis After Vascular Injury. Circ Res 2016;118:1170-84. [Crossref] [PubMed]