Recent developments in the role of reactive oxygen species in allergic asthma
Introduction
Asthma is a characteristic of different phenotypes of chronic airway inflammation, including allergic asthma and non-allergic (intrinsic) asthma. Allergic asthma is a chronic airway inflammatory disease in which many immune regulatory cells within the immune system work together with epithelial cells to cause smooth muscle contraction, enhance airway hyper-reactivity (AHR), increase mucus secretion and stimulate bronchospasm. Non-allergic asthma often develops later in life and, per its definition, has neither immunoglobulin E (IgE) reactivity to allergens nor any obvious involvement of the adaptive immune system, such as type 2 helper T cells (Th2 cells) (1,2). Allergic asthma is more prevalent than non-allergic asthma. In our review, we focus on allergic asthma. In susceptible patients, the main clinical symptoms of allergic asthma are shortness of breath, chest tightness and wheezing (3). Allergic asthma is very common and afflicts 8.5% of the U.S. population and is estimated to cause >3,000 deaths and to cost more than $56 billion annually in lost work and medical expenses (4). Many patients use corticosteroids and a short- or long-acting β-adrenergic agonist to treat asthma; however, in 5–10% patients, therapy fails (5).
It is well known that reactive oxygen species (ROS), endogenous nitric oxide (NO) and NO derived reactive nitrogen species (RNS) have been reported to mediate oxidative stress and airway inflammation and pathogenesis. However, ROS are more common in allergic asthma development (6,7). Hence, in our review, we mainly focus on the relationship between ROS and allergic asthma. ROS, such as hydrogen peroxide, may be directly or indirectly involved in epithelial cell apoptosis and inactivation of antioxidant enzymes and contribute to allergic asthma (8-11). ROS are derived from endogenous or exogenous oxidants. Endogenous ROS are composed of mitochondria, NADPH, and the xanthine/xanthine oxidase (XO) system. Exogenous sources of ROS production have been linked to strong oxidizing gases, such as ozone (O3), sulfur dioxide (SO2), nitrogen dioxide (NO2) and particulate matter (PM), which are a major component of air pollution (12,13). The oxygen stress level in asthma is increased because of inflammatory cells in vivo, and cigarette smoke (CS) or PM produces ROS in vitro. For example, compared with normal subjects, the level of H2O2 and superoxide that are produced by eosinophils and neutrophils are significantly increased in asthma patients (14). Increased levels of ROS can cause harmful pathophysiological disorders of allergic asthma. Studies have reported that excessive ROS can damage DNA, carbohydrates, proteins, and lipids, ultimately leading to an increased inflammatory response in allergic asthma (15,16). In this review, we will discuss the updated pathophysiological and mechanisms of oxidative stress relating to allergic asthma.
Exogenous oxidants and allergic asthma
Patients’ exposure to the environmental atmosphere is the main way that exogenous oxidative stress is induced (17). CS is a complex mixture including more than 4,000 different compounds. It has been demonstrated that inhaled CS can increase ROS levels. Most of these compounds have ability to generate ROS during their metabolism. The mechanism of CS induced inflammation is incorporated with oxidative stress leading to cell death and oxidative DNA damage via apoptosis and/or necrosis (7). It was reported that antioxidants have the ability to protect cells from cigarette smoke extract (CSE) induced apoptosis (18). Isolated human airway smooth muscle (hASM) cells were used to determine Ca2+ responses and hASM proliferation, as well as ROS level and cytokine generation, by incubating these cells in the presence or absence of CSE. The results revealed that Ca2+ regulatory proteins alter airway remolding and function in smoking-related airway disease, especially allergic asthma (19). CSE can damage bronchial epithelial cells from asthmatic patients through regulation of apoptosis-inducing factors, which is implicated in DNA damage and ROS-mediated cell-death (18). Recently the nanoparticles (NPs) that particle diameter less than 100 nano including engineering NPs and natural formation of the NPs already exist in the air, such as photochemical smog, forest fires, car exhaust. The NPs could exacerbate the inflammatory responses in lungs. PM and is consistently involve in respiratory diseases, such as asthma, through oxidative stress pathways (20). PM is capable of generating free radicals, the metals on their surface takes part in redox cycling or depletion of anti-oxidant glutathione function and PM2.5 means the particle diameter less than or equal to 2.5 µm (21,22). Josephine was the first to estimate population-level health effects through ROS activity measurements and to use an epidemiologic approach to link PM2.5 ROS to asthma. The results showed that reducing water-soluble PM2.5 (WS-PM2.5) dithiothreitol (DTT) activity may significantly decreasing the incidence of asthma (23). A meta-analysis showed that particle components play a pro-oxidant role that thereby promote airway inflammation (24). It is well known that the aryl hydrocarbon receptor (AhR) is recognized as a receptor for environmental contaminants. AhR induction of increased mucin production is partially mediated by ROS generation, and antioxidant therapy can mitigate AhR-induced mucus hyper-secretory diseases (25). AhR ligands have been shown to induce mast cells to increase ROS production in asthma (26). Ozone could be an inflammatory mediator by scavenging ROS; for example, electron-rich olefins could be used to treat asthma (27). Additionally, asbestos, coal, and pollens also induce excessive ROS formation levels that are related to immune responses associated with allergic asthma (28,29).
Endogenous oxidants and allergic asthma
Intracellular ROS are mainly composed of mitochondria, NADPH oxidase, and the xanthine/XO system. The generation of superoxide anion is mediated by mitochondria and NADPH oxidase. It was reported that eosinophils and neutrophils could produce more superoxide anion from asthmatic patients compared with healthy subjects due to NADPH oxidase activation during asthma (30). The NADPH oxidase, dual oxidase 1 (DUOX1), critically contributes to airway epithelial secretion of interleukin-33 (IL-33), which is dependent on Src/EGFR pathway activation in asthma (31). Leukotriene C4 (LTC4) can elicit nuclear translocation of NADPH oxidase 4 (NOX4), ROS accumulation and oxidative DNA damage. LTC4 inhibitors, which are used clinically for asthma, were associated with endoplasmic reticulum (ER) stress activated NOX4 (32). It is interesting that NADPH oxidase 2 (Nox2) deficiency results in induced experimental asthma, which is caused by aggravate Th2 effector function in a T cell intrinsic manner; however, the underlying mechanism must be studied further (32). Superoxide anion can also be generated from mitochondrial respiration chain reactions. Mitochondria are double membrane organelles that possess a proteome and genome (33). Additionally, they are capable of synthesizing and catabolizing metabolites and generating ROS (34). Silencing of mitochondrial transcription factor A (TFAM) not only dramatically reduces bronchial smooth muscle (BSM) cell proliferation but also abolishes the difference between BSM cell proliferation in control subjects and asthmatic patients (35). A study focused on the relationship between mitochondrial ROS and NLRP3 inflammasome in asthma. NecroX-5, a novel mitochondrial ROS inhibitor can decrease protein levels of NLRP3, caspase-1 and IL-1β in tracheal epithelial cells (36). Xanthine oxidoreductase (XOR) is a highly conserved member of the molybdoenzyme family, which exists in two forms, xanthine dehydrogenase (XDH) and XO. The baseline expression of XOR is low; however, transcription can be up-regulated due to several factors, such as hypoxia, lipopolysaccharide (LPS), IFN-γ, IL-1, IL-6, tumor necrosis factor-α (TNF-α), and steroid stimulation. XOR activation is increased in the late allergic response phase (37).
Effect of oxidants on cells during allergic asthma
Epithelial cells
The barrier function of epithelial cells is often injured in allergic asthmatic patients. Epithelial cells are more easily affected by ROS derived from infiltrated inflammatory cells. In fact, IL-13 treatment with primary human tracheal epithelial can stimulate mucus formation and ROS production. However, the muc5ac secretion and ROS levels can be inhibited in epithelial cells that are depleted of the autophagy genes, autophagy related 5 or autophagy related 14, compared with non-depleted control cells. These findings indicate that autophagy is essential for IL-13 to modulate ROS and mucus formation in epithelial cells during asthma (38). A study suggested that proteinase-activated receptor 2 (PAR-2) is involved in airway inflammation regulation through oxidative stress, leading to increased DUOX-2/ROS pathway activation in airway epithelial cells (39). In human bronchial epithelial cells, lack of glutathione S-transferase Mu 1 (GSTM1) enhances ozone-induced IL-8 production because of ROS generation and subsequent NF-κB activation (40).
Airway smooth muscle and endothelial cells
Asthma can activate mitochondrial ATP-sensitive potassium channels (MitoKATP) in human airway smooth muscle cells (HASMCs) and promote HASMC proliferation and TGF-β1 expression (41). It was reported that even low CSE levels have a substantial impact on ASMC mitochondria and increased Drp1 expression, which subsequently affects mitochondrial morphology and energy production (42). ROS induce increased Ca2+, which results in contraction of airway smooth muscle cells that are treated with ovalbumin (OVA), resulting in airway hyper-responsiveness (43). AMP-activated protein kinase (AMPK) may be a possible therapeutic target for asthma because AMPK can ameliorate airway inflammatory responses by inhibiting ROS production and reducing vascular permeability via the HIF/VEGFA pathway (44). A murine model of asthma used to evaluate the effect of L-2-oxothiazolidine-4-carboxylic acid (OTC), a cysteine pro-drug that acts as an antioxidant, was shown to increase vascular permeability. When OTC was administered in the mouse model, it markedly reduced plasma extravasation and vascular endothelial growth factor (VEGF) levels in allergen-induced asthmatic lungs (45).
Inflammatory cells
Oxidants were reported to have various effects on inflammatory cells. Dendritic cell (DC) function is altered in the absence of a functional NADPH oxidase complex, providing a potential mechanism for the decreased allergic airway inflammation observed in an asthma model (46). Oxidant gasses can directly activate DCs. DCs play an important role in allergic asthma due to their potent ability to activate naive CD4+ T cells (47). If mast cells are exposed to oxidants, then they release significantly amounts of histamine and serotonin. Activated mast cells have increased intracellular ROS levels, and ROS enhance histamine release from mast cells (48). Exposure to AhR ligands results in an ROS-dependent increase of reversible oxidation, leading to enhanced mast cell function in OVA-stimulated mast cells (26). DJ-1 is an antioxidant protein. DJ-1-KO mice exhibited enhanced passive cutaneous anaphylaxis (PCA) and augmented ROS levels in sera and in bone marrow-derived mast cells (BMMCs) (49). During airway inflammation, peripheral blood eosinophils are increased by enhanced spontaneous ROS production and increased cytokines in allergic asthma patients (50,51).
Redox signaling mechanisms in allergic asthma
Hallmark Th2 disorders are the main cause of asthma. Antigen-presenting DCs that recognize and present allergens to T cells in the lymph nodes that activate CD4+ cells that produce IL-4 and IL-5 were found in the bronchoalveolar lavage fluid (BALF) and were correlated with the degree of airway eosinophilia (48). IL-4 induces immunoglobulin synthesis by B cells and release of IgE, which is a critical hallmark of TH2 immunity (52). IgE binds to its receptor, FcεRI, which is located on mast cells and regulates ROS. IL-5 is responsible for the generation and activation of eosinophils to mediate ROS generation. IL-13 is responsible for AHR and goblet cell metaplasia (53). It was reported that IL-4, IL-5 and IL-13 can regulate DUOX activity that is mediated by H2O2, which influences several important features of allergic asthma (54). Moreover, TNF-α and IL-1 can induce ROS formation. These critical mediators may activate mellatoproteinase-9 (MMP-9), intracellular adhesion molecule-1 (ICAM-1), and vascular cell adhesion molecule-1 (VCAM-1), which promotes ROS production and inflammation and exacerbates allergic asthma (55). ROS have been shown to increase smooth muscle contraction, enhance AHR and stimulate mucus secretion (Figure 1) (1,2).
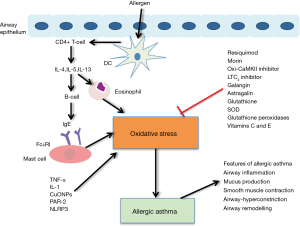
Current research has also provided more signaling molecules that are related with critical redox reactions during allergic asthma. The expression of copper oxide nanoparticles (CuONPs), which are metal oxide NPs, was increased in an OVA-induced asthma murine model. CuONPs markedly increase AHR, cytokines, and ROS (56,57). PAR-2 mediated DUOX-2 and ROS in airway epithelial cells in a cockroach allergen mice model. However, ENMD-1068, a small molecule antagonist of PAR-2, can inhibit DUOX-2 and ROS in allergic mice (39). NLRP3 inflammasome assembly is activated by mitochondrial ROS, which consequently induces IL-1β production and release (36). In OVA sensitized murine models, a decrease in cellular glutathione, which is an antioxidant, is involved in the resolution of airway inflammation and respiratory hypersensitivity during the sensitization stage, which has been associated with NOX inhibition and enhanced ROS generation (58). Moreover, decreased glutathione levels have been suggested to induce changes in TH1 to TH2 cell differentiation. Under these circumstances, the oxidant and antioxidant balance is lost and AHR is induce by the synthesis of S-nitrosoglutathione and a corresponding shift in NO metabolism (58,59). Another important redox mediator, glutathione S-transferase pi, which was found in lung epithelium, primarily facilitates the conjugation of reduced glutathione to mitigate the electrophilic properties of inhaled antigens and potentially reactive molecules (60). The multifunctional Ca2+ and calmodulin-dependent protein kinase II (CaMKII) can be activated by ROS and contribute to inflammation. Oxidative CaMKII was increased in airway epithelium biopsied from mildly asthmatic patients and OVA or aspergillus fumigatus challenged mouse models (61). A mouse model that evaluated airway epithelium with a specific deficiency in CaMKII expression showed clinical features that included inhibition of AHR airway epithelial proliferation and mucus secretion (62).
Redox therapeutics
The antioxidant system is well developed in allergic asthma. Generally, antioxidants can be divided into enzymatic [glutathione peroxidase and superoxide dismutase (SOD)] and non-enzymatic (vitamin E, vitamin C) subcategories, which play a critical role in the inhibition and elimination of oxidative damage. Recently, new treatments for ROS in allergic asthma were reported in several studies. Table 1 shows the antioxidant system.
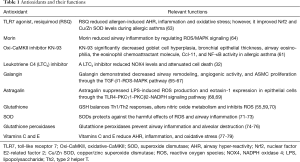
Full table
The toll-like receptor 7 (TLR7) agonist, resiquimod (RSQ)
TLRs play an important role in host defense against bacteria, fungi, viruses and allergies through the innate immune system. Accumulating evidence suggests that TLR-associated inflammation is involved in NOX-deriving ROS generation (39). It was shown that the TLR7 activation is protective in mouse models of asthma. Several mechanisms have been reported that contribute to the ability for TLR-7 to have a critical anti-inflammatory effect in asthma. In a study using OVA intraperitoneally to challenge mice that were intranasally treated with the TLR-7 agonist, RSQ, AHR, inflammation, NOX-4 and nuclear factor E2-related factor 2 (Nrf2) related signaling levels [NOS, nitrotyrosine, lipid peroxides and copper/zinc superoxide dismutase (Cu/Zn SOD)] were assessed. The results show that treatment with RSQ reduced AHR, inflammation and ROS; however, it improved Nrf2 and Cu/Zn SOD levels in an allergic asthma mouse model. These results from this study suggest that RSQ is beneficial and should be further developed as a therapeutic option in allergic asthma (63).
Morin
Morin (3,5,7,2’,4’-pentahydroxyflavone) exists in high concentrations in many herbs, such as Osage orange, Cudrania tricuspidata, and other moraceae family members. Morin has been shown to have strong anti-inflammatory and anti-tumor functions. Accumulating data have indicated that morin suppresses the growth of hepatocellular carcinoma (80) and decreases immune inflammatory responses in chronic colitis (81). However, the role of morin in the treatment of allergic asthma is still unknown. It was reported that in an OVA challenge allergic asthma model, inflammatory cell infiltration around the airways and vessels, goblet cell hyperplasia, collagen deposition/fibrosis and cytokine expression levels were dramatically attenuated following morin treatment. Western blotting and the 2’,7’-dichlorofluorescein assay revealed that the intracellular ROS and MAPK phosphorylation levels were abolished with morin treatment (64). A polyphenol flavonol derived morin treatment in an OVA-challenged allergic asthma model also reduced IL-4 and IL-13, lung tissue eosinophil and mastocyte infiltration levels (82). Both of these studies demonstrated novel profiles for morin as a potent asthma management agent.
Oxidative-CaMKII (Oxi-CaMKII) inhibitor
CaMKII is a ubiquitously expressed multimeric serine threonine kinase consisting of 12 subunits. Each subunit is composed of three conserved domains, including an amino terminal catalytic domain that provides the binding sites for ATP and substrates, a central auto-regulatory domain that has an inhibitory pseudosubstrate sequence and several sites for post-translational modifications and a carboxy-terminal associated domain for the oligomerization of subunits to form the holoenzyme and variable regions that are spliced to form various splice variants. CaMKII activity can also be enhanced by ROS, including NADPH oxidase. Mitochondria oxidizes its methionines at 281 and 282, leading to the development of autonomous enzyme activity, which is called Oxi-CaMKII (83). Elimination of ROS generation by blocking either of the pathways results in a significant decline in Oxi-CAMKII levels. Increased Oxi-CaMKII levels were also detected in OVA challenged allergic asthma mice, compared with vehicle treatment, without any significant increase in total CaMKII levels. Moreover, the Oxi-CaMKII levels were higher in severe asthmatics compared with mild asthmatics and healthy individuals, suggesting that Oxi-CaMKII is not only an indicator of asthma but also a marker of disease severity (61). The p47 gene encodes for NADPH oxidase, which is required for ROS generation (84). p47−/− OVA challenged asthma mice were found to have reduced ROS and Oxi-CaMKII levels compared with wild-type OVA challenged mice. Additionally, inhalation of the Oxi-CaMKII antagonist, KN-93, significantly decreased goblet cell hyperplasia, bronchial epithelial thickness, MUC5AC positive airway cells, Muc5ac mRNA expression, airway eosinophilia, eosinophil chemoattractant molecule Ccl-11, and NF-κB activity in allergic asthma (61).
LTC4 inhibitors
LTC4 production is triggered in ER stress through oxidative stress. The role of LTC4 in allergic asthma has been extensively evaluated (85). LTC4 is produced in mast cells by assembly of a biosynthetic complex at the nuclear envelope, consisting of cytosolic phospholipase A2 (cPLA2), 5-lipoxygenase (5-LO), 5-LO activating protein (FLAP) and LTC4 synthase (LTC4S). Dvash reported that microsomal glutathione-S-transferase 2 (MGST2)-LTC4 can be elicited by the ER stress. This pathway is the major mediator of ER stress triggered oxidative stress and oxidative DNA damage, which associates with NOX4 and participates in ER stress-triggered cell death. However, the level of NOX4 under extensive ER stress is greatly reduced using LTC4 antagonists. LTC4 receptor antagonists also attenuated cell death and mouse morbidity (32). Now, LTC4 inhibitors are widely used in the clinical treatment of asthma.
Galangin
Galangin (3,5,7-trihydroxyflavone) is a plant that has been used as a herbal medicine for a variety of ailments and exists in high concentrations in honey and alpinia officinarum (65,86). A study showed that galangin exhibited anti-inflammatory and antioxidant activity in vitro due to having an affinity towards the COX-2 active site, which was further explored with selective COX-2 inhibitors (66). Galangin also attenuates mast cell function, including decreasing histamine and cytokines release. Furthermore, galangin inhibited IgE-mediated PCA in the inflamed tissue. Galangin inhibited pro-inflammatory cytokine expression, including TNF-α, IL-6, IL-1β, and IL-8, by regulating c-Jun N-terminal kinases and p38 mitogen-activated protein kinase, nuclear factor-κB, and caspase-1 expression (67). Another study revealed that galangin could markedly attenuate the extent of chronic inflammation and airway remodeling in OVA challenged asthma mice, including attenuating inflammatory cell infiltration into the BALF and decreasing the level of OVA-specific IgE in the serum. Furthermore, TGF-β1 and VEGF levels were also reduced following galangin treatment. Additionally, galangin inhibited TGF-β1-induced ASMC proliferation in vitro, which involved the ROS level attenuation and ERK, JNK and Akt phosphorylation inhibition. This was the first article to report the potential role of galangin on airway remodeling through TGF-β1-ROS-MAPK signaling, which may provide a promising therapeutic treatment for asthma patients (65).
Astragalin
Astragalin, which is a kaempferol-3-O-glucoside found in persimmon leaves and green tea seeds, possesses anti-inflammatory activity (87,88). It was reported that astragalin inhibited eosinophil infiltration in an OVA-induced asthma model. IL-4, IL-5 and IL-13 were decreased after astragalin treatment. Histological studies demonstrated that astragalin substantially inhibited OVA-induced eosinophilia in lung tissue. All of these anti-inflammatory roles may occur through suppression of cytokine signaling (SOCS)-3 and enhancement of SOCS-5 expression in an asthma model (68). Another study investigated the potential of astragalin and found that it can antagonize oxidative stress-associated airway eosinophilia and epithelial apoptosis. Astragalin suppresses LPS-induced ROS production and eotaxin-1 expression in epithelial cells. The LPS induction of eotaxin-1 was linked to ROS through the TLR4-signaling pathway and PKCγ1-PKCβ2-NADPH oxidases were disturbed by astragalin. Additionally, astragalin endotoxin-instigated epithelial apoptosis was attenuated through manipulating oxidative stress-elicited MAPK signaling in airway epithelial cells. Therefore, astragalin may serve as a modulator against asthma (69).
Glutathione
Glutathione has an SH residue and reacts with oxygen radicals. Glutathione plays an important role in several respiratory diseases and can act against oxidative inflammation along with other enzymatic/non-enzymatic antioxidants. Glutathione can also affect cellular signaling through regulation of redox sensitivity, transcription factors and phosphatases (89,90). Furthermore, glutathione levels can be decreased due to several environment pollutants that have been linked to increased asthma prevalence worldwide (70,91). Glutathione attenuated AHR and inflammation could occur through several mechanisms: (I) the Th1/Th2 balance (70); (II) alteration of NO metabolism through the formation of S-nitrosoglutathione, which was reported to be associated with regulation of airway responses (59); and (III) altering the balance between ROS inhibition and antioxidant reaction (55). Buthionine sulfoximine (BSO) was used for depletion or repletion of glutathione levels during sensitization and challenge phases, respectively, followed by assessment of AHR, inflammation and oxidant-antioxidant balance in an allergy mouse model. A study found that glutathione depletion with BSO induced AHR and airway inflammation and caused a greater oxidant-antioxidant imbalance, as reflected by increased NADPH oxidase expression/ROS generation and decreased total antioxidant capacity. This study indicates that ROS generation in allergic asthma mice was aggravated due to oxidized glutathione and decreased airway responses (58).
SODs
SODs are known as protective antioxidants against the harmful effects of ROS. All forms of SODs act through a common mechanism: dismutation of the superoxide anion to the less potent hydrogen peroxide. Several forms of SODs exist, including Cu/Zn SOD, MnSOD, and extracellular SOD (EC-SOD) (92). Cu/Zn SOD can suppress AHR indicating that the generation of superoxide anion is associated with AHR formation (71). SOD3 is an important isoform of SOD. Several studies have focused on the antioxidant role of SOD3. A study reported for the first time that SOD3 specifically inhibits DC maturation. Subsequently, SOD3 controls T cell activation and proliferation, Th2 and Th17 cell differentiation and inhibits OVA-induced airway remodeling. Interestingly, SOD3 administration in mice alleviated OVA-induced allergic asthma (72,73). Despite the advantages as an anti-inflammatory and antioxidant reagent, it is difficult to obtain large quantities of active recombinant human SOD3 (rhSOD3). A new study found that incorporating metal ions into rhSOD3 after purification maximized the enzymatic activity of rhSOD3. Additionally, albumin or polyethylene glycol prevented rapid inactivation or degradation of rhSOD3 during preparative procedures and long-term storage (72).
Glutathione peroxidases
Glutathione peroxidases are a family of selenium-dependent and selenium-independent antioxidant enzymes. Glutathione peroxidase may decrease airway inflammation and AHR in asthmatic patients because selenium-related antioxidants are powerful scavengers of peroxynitrite (93). An interesting study found that in a susceptible subgroup of mother-child pairs in a population, namely, pregnant women with suboptimal selenium status and offspring with the homozygous minor variant of glutathione peroxidases 4, the incidence of childhood asthma was decreased (74). A study showed the glutathione peroxidase levels were lower in an allergic asthma group compared with a healthy group (75). The expression of human glutathione peroxidase-1 (GPx-1) in transgenic mice significantly increased protein phosphatase-2A (PP2A) and protein tyrosine phosphatase-1B (PTP1B) activities and prevented chronic CS-induced airway inflammation. However, the reverse was observed in GPx-1 knockout mice, including an exaggerated emphysema phenotype and airway inflammation (76).
Vitamins C and E
Dietary intake of vitamin C can reduce the risk of asthma and was associated with increased glutathione peroxidase activity (94). Excessive ROS production decreases enzymatic and non-enzymatic antioxidant levels, such as glutathione, vitamins C and E, β-carotene and glutathione peroxidases, leading to an imbalance of oxidants-antioxidants in airways. It was reported the combination of vitamin C, choline chloride, and selenium reduces AHR, airway inflammation, and ROS levels, probably by inducing IL-10 production by FOXP3+ cells, implicating that vitamin C might be a potential therapeutic against allergic airway disease (77). Vitamin E has antioxidant properties and is comprised of α, β, γ, and δ isoforms for both tocopherols and tocotrienols (95). It is well known that tocopherol is regarded as the isoform that includes the anti-oxidative and anti-inflammatory effects of vitamin E, and some evidence has pointed to γ-tocotrienol as the superior bioactive isoform of vitamin E (95,96). A study demonstrated that oral γ-tocotrienol markedly abated house dust mite (HDM)-induced increases in airway inflammatory, serum IgE levels, cytokine and chemokine levels, mucus production, and oxidative damage biomarkers. The protective mechanisms of γ-tocotrienol are likely mediated by blocking nuclear NF-κB translocation and augmenting Nrf2 nuclear levels, as shown in an allergic asthma model (78). Harada showed that γ-tocotrienol could contribute to the therapeutic airway remodeling benefits in asthma by inhibiting HASMC proliferation and migration (79).
Significance and future directions
In this review, we have discussed the relationships between ROS and inflammation in asthma. The effect of oxidants on cells in asthma was also discussed. Allergic asthma is associated with acute increases in ROS, AHR and mucus secretion levels in response to allergen exposure, leading to oxidant and antioxidant imbalances, initiation of inflammatory signaling pathways, and oxidative stress. Through the use of animal studies and clinical trials, ROS have been found to play a central role in the pathogenesis of allergic asthma. Several new antioxidant methods have been tested in animal studies; however, they have not been evaluated in asthma patients. In the future, human and animal studies should bridge the transition from the bench to the clinic. Moreover, a standard scale should be created to assess the degree of disease progression and severity with regard to the identity and quantity of oxidative stress byproducts. Further research should be focused on the identification of new therapy methods with regard to ROS in allergic asthma.
Acknowledgements
Funding: This study was supported in part by grants from the Central South University Innovation Foundation for Postgraduates (2015zzts114), Scientific Research Foundation of Health and Family Planning Commission of Hunan Province (132015-012) and the National Key Scientific & Technology Support Program: Collaborative Innovation of Clinical Research for Chronic Obstructive Pulmonary Disease and Lung Cancer (No. 2013BAI09B09).
Footnote
Conflicts of Interest: The authors have no conflicts of interest to declare.
References
- Cho YS, Moon HB. The role of oxidative stress in the pathogenesis of asthma. Allergy Asthma Immunol Res 2010;2:183-7. [Crossref]
- Hung CY, Huang FL, Shi LS, et al. The Ethanol Extract of Osmanthus fragrans Flowers Reduces Oxidative Stress and Allergic Airway Inflammation in an Animal Model. Evid Based Complement Alternat Med 2013;2013:304290.
- Löwhagen O. Diagnosis of asthma - a new approach. Allergy 2012;67:713-7. [Crossref]
- Barnett SB, Nurmagambetov TA. Costs of asthma in the United States: 2002-2007. J Allergy Clin Immunol 2011;127:145-52. [Crossref]
- Szefler SJ. Advancing asthma care: the glass is only half full! J Allergy Clin Immunol 2011;128:485-94. [Crossref]
- Jiang L, Diaz PT, Best TM, et al. Molecular characterization of redox mechanisms in allergic asthma. Ann Allergy Asthma Immunol 2014;113:137-42. [Crossref]
- Zuo L, Koozechian MS, Chen LL. Characterization of reactive nitrogen species in allergic asthma. Ann Allergy Asthma Immunol 2014;112:18-22. [Crossref]
- Comhair SA, Erzurum SC. Redox control of asthma: molecular mechanisms and therapeutic opportunities. Antioxid Redox Signal 2010;12:93-124. [Crossref]
- Nadeem A, Siddiqui N, Alharbi NO, et al. Airway and systemic oxidant-antioxidant dysregulation in asthma: a possible scenario of oxidants spill over from lung into blood. Pulm Pharmacol Ther 2014;29:31-40. [Crossref]
- Nadeem A, Masood A, Siddiqui N. Oxidant--antioxidant imbalance in asthma: scientific evidence, epidemiological data and possible therapeutic options. Ther Adv Respir Dis 2008;2:215-35. [Crossref]
- Saleh D, Ernst P, Lim S, et al. Increased formation of the potent oxidant peroxynitrite in the airways of asthmatic patients is associated with induction of nitric oxide synthase: effect of inhaled glucocorticoid. FASEB J 1998;12:929-37.
- Zuo L, Clanton TL. Reactive oxygen species formation in the transition to hypoxia in skeletal muscle. Am J Physiol Cell Physiol 2005;289:C207-16. [Crossref]
- Zuo L, Nogueira L, Hogan MC. Reactive oxygen species formation during tetanic contractions in single isolated Xenopus myofibers. J Appl Physiol (1985) 2011;111:898-904.
- Loukides S, Bouros D, Papatheodorou G, et al. The relationships among hydrogen peroxide in expired breath condensate, airway inflammation, and asthma severity. Chest 2002;121:338-46. [Crossref]
- Henricks PA, Nijkamp FP. Reactive oxygen species as mediators in asthma. Pulm Pharmacol Ther 2001;14:409-20. [Crossref]
- Suski JM, Lebiedzinska M, Bonora M, et al. Relation between mitochondrial membrane potential and ROS formation. Methods Mol Biol 2012;810:183-205. [Crossref]
- To T, Daly C, Feldman R, et al. Results from a community-based program evaluating the effect of changing smoking status on asthma symptom control. BMC Public Health 2012;12:293. [Crossref]
- Bucchieri F, Marino Gammazza A, Pitruzzella A, et al. Cigarette smoke causes caspase-independent apoptosis of bronchial epithelial cells from asthmatic donors. PLoS One 2015;10:e0120510. [Crossref]
- Wylam ME, Sathish V, VanOosten SK, et al. Mechanisms of Cigarette Smoke Effects on Human Airway Smooth Muscle. PLoS One 2015;10:e0128778. [Crossref]
- Saravia J, Lee GI, Lomnicki S, et al. Particulate matter containing environmentally persistent free radicals and adverse infant respiratory health effects: a review. J Biochem Mol Toxicol 2013;27:56-68. [Crossref]
- Stohs SJ, Bagchi D, Hassoun E, et al. Oxidative mechanisms in the toxicity of chromium and cadmium ions. J Environ Pathol Toxicol Oncol 2001;20:77-88. [Crossref]
- Valko M, Morris H, Cronin MT. Metals, toxicity and oxidative stress. Curr Med Chem 2005;12:1161-208. [Crossref]
- Bates JT, Weber RJ, Abrams J, et al. Reactive Oxygen Species Generation Linked to Sources of Atmospheric Particulate Matter and Cardiorespiratory Effects. Environ Sci Technol 2015;49:13605-12. [Crossref]
- Delfino RJ, Staimer N, Tjoa T, et al. Airway inflammation and oxidative potential of air pollutant particles in a pediatric asthma panel. J Expo Sci Environ Epidemiol 2013;23:466-73. [Crossref]
- Chiba T, Uchi H, Tsuji G, et al. Arylhydrocarbon receptor (AhR) activation in airway epithelial cells induces MUC5AC via reactive oxygen species (ROS) production. Pulm Pharmacol Ther 2011;24:133-40. [Crossref]
- Zhou Y, Tung HY, Tsai YM, et al. Aryl hydrocarbon receptor controls murine mast cell homeostasis. Blood 2013;121:3195-204. [Crossref]
- Bibi H, Reany O, Waisman D, et al. Prophylactic treatment of asthma by an ozone scavenger in a mouse model. Bioorg Med Chem Lett 2015;25:342-6. [Crossref]
- Andreau K, Leroux M, Bouharrour A. Health and cellular impacts of air pollutants: from cytoprotection to cytotoxicity. Biochem Res Int 2012;2012:493894.
- Boldogh I, Bacsi A, Choudhury BK, et al. ROS generated by pollen NADPH oxidase provide a signal that augments antigen-induced allergic airway inflammation. J Clin Invest 2005;115:2169-79. [Crossref]
- Polonikov AV, Ivanov VP, Bogomazov AD, et al. Genetic and biochemical mechanisms of involvement of antioxidant defense enzymes in the development of bronchial asthma. Biomed Khim 2015;61:427-39. [Crossref]
- Hristova M, Habibovic A, Veith C, et al. Airway epithelial dual oxidase 1 mediates allergen-induced IL-33 secretion and activation of type 2 immune responses. J Allergy Clin Immunol 2016;137:1545-1556.e11. [Crossref]
- Dvash E, Har-Tal M, Barak S, et al. Leukotriene C4 is the major trigger of stress-induced oxidative DNA damage. Nat Commun 2015;6:10112. [Crossref]
- Emelyanov VV. Mitochondrial connection to the origin of the eukaryotic cell. Eur J Biochem 2003;270:1599-618. [Crossref]
- Cloonan SM, Choi AM. Mitochondria: commanders of innate immunity and disease? Curr Opin Immunol 2012;24:32-40. [Crossref]
- Trian T, Allard B, Ozier A, et al. Selective dysfunction of p53 for mitochondrial biogenesis induces cellular proliferation in bronchial smooth muscle from asthmatic patients. J Allergy Clin Immunol 2016;137:1717-1726.e13. [Crossref]
- Kim SR, Kim DI, Kim SH, et al. NLRP3 inflammasome activation by mitochondrial ROS in bronchial epithelial cells is required for allergic inflammation. Cell Death Dis 2014;5:e1498. [Crossref]
- Sugiura H, Liu X, Kobayashi T, et al. Reactive nitrogen species augment fibroblast-mediated collagen gel contraction, mediator production, and chemotaxis. Am J Respir Cell Mol Biol 2006;34:592-9. [Crossref]
- Dickinson JD, Alevy Y, Malvin NP, et al. IL13 activates autophagy to regulate secretion in airway epithelial cells. Autophagy 2016;12:397-409. [Crossref]
- Nadeem A, Alharbi NO, Vliagoftis H, et al. Proteinase activated receptor-2-mediated dual oxidase-2 up-regulation is involved in enhanced airway reactivity and inflammation in a mouse model of allergic asthma. Immunology 2015;145:391-403. [Crossref]
- Wu W, Doreswamy V, Diaz-Sanchez D, et al. GSTM1 modulation of IL-8 expression in human bronchial epithelial cells exposed to ozone. Free Radic Biol Med 2011;51:522-9. [Crossref]
- Chen C, Wang R, Zhou S, et al. Effects of mitochondrial ATP-sensitive potassium channels on the proliferation and secretion of human airway smooth muscle cells. Iran J Allergy Asthma Immunol 2014;13:420-7.
- Aravamudan B, Kiel A, Freeman M, et al. Cigarette smoke-induced mitochondrial fragmentation and dysfunction in human airway smooth muscle. Am J Physiol Lung Cell Mol Physiol 2014;306:L840-54. [Crossref]
- Tuo QR, Ma YF, Chen W, et al. Reactive oxygen species induce a Ca(2+)-spark increase in sensitized murine airway smooth muscle cells. Biochem Biophys Res Commun 2013;434:498-502. [Crossref]
- Park SJ, Lee KS, Kim SR, et al. AMPK activation reduces vascular permeability and airway inflammation by regulating HIF/VEGFA pathway in a murine model of toluene diisocyanate-induced asthma. Inflamm Res 2012;61:1069-83. [Crossref]
- Lee KS, Park HS, Park SJ, et al. A prodrug of cysteine, L-2-oxothiazolidine-4-carboxylic acid, regulates vascular permeability by reducing vascular endothelial growth factor expression in asthma. Mol Pharmacol 2005;68:1281-90. [Crossref]
- van der Vliet A. Nox enzymes in allergic airway inflammation. Biochim Biophys Acta 2011;1810:1035-44.
- Ckless K, Hodgkins SR, Ather JL, et al. Epithelial, dendritic, and CD4(+) T cell regulation of and by reactive oxygen and nitrogen species in allergic sensitization. Biochim Biophys Acta 2011;1810:1025-34.
- Lambrecht BN, Hammad H. Lung dendritic cells in respiratory viral infection and asthma: from protection to immunopathology. Annu Rev Immunol 2012;30:243-70. [Crossref]
- Kim DK, Kim HS, Kim AR, et al. DJ-1 regulates mast cell activation and IgE-mediated allergic responses. J Allergy Clin Immunol 2013;131:1653-62. [Crossref]
- Lavinskiene S, Malakauskas K, Jeroch J, et al. Functional activity of peripheral blood eosinophils in allergen-induced late-phase airway inflammation in asthma patients. J Inflamm (Lond) 2015;12:25. [Crossref]
- Gaurav R, Bewtra AK, Agrawal DK. Chloride Channel 3 Channels in the Activation and Migration of Human Blood Eosinophils in Allergic Asthma. Am J Respir Cell Mol Biol 2015;53:235-45. [Crossref]
- Cheng D, Xue Z, Yi L, et al. Epithelial interleukin-25 is a key mediator in Th2-high, corticosteroid-responsive asthma. Am J Respir Crit Care Med 2014;190:639-48. [Crossref]
- Zaiss DM, Gause WC, Osborne LC, et al. Emerging functions of amphiregulin in orchestrating immunity, inflammation, and tissue repair. Immunity 2015;42:216-26. [Crossref]
- Chang S, Linderholm A, Franzi L, et al. Dual oxidase regulates neutrophil recruitment in allergic airways. Free Radic Biol Med 2013;65:38-46. [Crossref]
- Lee IT, Yang CM. Role of NADPH oxidase/ROS in pro-inflammatory mediators-induced airway and pulmonary diseases. Biochem Pharmacol 2012;84:581-90. [Crossref]
- Park JW, Lee IC, Shin NR, et al. Copper oxide nanoparticles aggravate airway inflammation and mucus production in asthmatic mice via MAPK signaling. Nanotoxicology 2016;10:445-52. [Crossref]
- Cho WS, Duffin R, Poland CA, et al. Differential pro-inflammatory effects of metal oxide nanoparticles and their soluble ions in vitro and in vivo; zinc and copper nanoparticles, but not their ions, recruit eosinophils to the lungs. Nanotoxicology 2012;6:22-35. [Crossref]
- Nadeem A, Siddiqui N, Alharbi NO, et al. Glutathione modulation during sensitization as well as challenge phase regulates airway reactivity and inflammation in mouse model of allergic asthma. Biochimie 2014;103:61-70. [Crossref]
- Ferrini ME, Simons BJ, Bassett DJ, et al. S-nitrosoglutathione reductase inhibition regulates allergen-induced lung inflammation and airway hyperreactivity. PLoS One 2013;8:e70351. [Crossref]
- Schroer KT, Gibson AM, Sivaprasad U, et al. Downregulation of glutathione S-transferase pi in asthma contributes to enhanced oxidative stress. J Allergy Clin Immunol 2011;128:539-48. [Crossref]
- Sanders PN, Koval OM, Jaffer OA, et al. CaMKII is essential for the proasthmatic effects of oxidation. Sci Transl Med 2013;5:195ra97. [Crossref]
- Zhang R, Khoo MS, Wu Y, et al. Calmodulin kinase II inhibition protects against structural heart disease. Nat Med 2005;11:409-17. [Crossref]
- Nadeem A, Siddiqui N, Al-Harbi NO, et al. TLR-7 agonist attenuates airway reactivity and inflammation through Nrf2-mediated antioxidant protection in a murine model of allergic asthma. Int J Biochem Cell Biol 2016;73:53-62. [Crossref]
- Ma Y, Ge A, Zhu W, et al. Morin Attenuates Ovalbumin-Induced Airway Inflammation by Modulating Oxidative Stress-Responsive MAPK Signaling. Oxid Med Cell Longev 2016;2016:5843672.
- Liu YN, Zha WJ, Ma Y, et al. Galangin attenuates airway remodelling by inhibiting TGF-beta1-mediated ROS generation and MAPK/Akt phosphorylation in asthma. Sci Rep 2015;5:11758. [Crossref]
- Honmore VS, Kandhare AD, Kadam PP, et al. Isolates of Alpinia officinarum Hance as COX-2 inhibitors: Evidence from anti-inflammatory, antioxidant and molecular docking studies. Int Immunopharmacol 2016;33:8-17. [Crossref]
- Kim HH, Bae Y, Kim SH. Galangin attenuates mast cell-mediated allergic inflammation. Food Chem Toxicol 2013;57:209-16. [Crossref]
- Liu J, Cheng Y, Zhang X, et al. Astragalin Attenuates Allergic Inflammation in a Murine Asthma Model. Inflammation 2015;38:2007-16. [Crossref]
- Cho IH, Gong JH, Kang MK, et al. Astragalin inhibits airway eotaxin-1 induction and epithelial apoptosis through modulating oxidative stress-responsive MAPK signaling. BMC Pulm Med 2014;14:122. [Crossref]
- Crinnion WJ. Do environmental toxicants contribute to allergy and asthma? Altern Med Rev 2012;17:6-18.
- Barnes PJ, Karin M. Nuclear factor-kappaB: a pivotal transcription factor in chronic inflammatory diseases. N Engl J Med 1997;336:1066-71. [Crossref]
- Kim S, Kim HY, Kim JH, et al. Enhancement of potency and stability of human extracellular superoxide dismutase. BMB Rep 2015;48:91-6. [Crossref]
- Kwon MJ, Jeon YJ, Lee KY, et al. Superoxide dismutase 3 controls adaptive immune responses and contributes to the inhibition of ovalbumin-induced allergic airway inflammation in mice. Antioxid Redox Signal 2012;17:1376-92. [Crossref]
- Shaheen SO, Rutterford CM, Lewis SJ, et al. Maternal selenium status in pregnancy, offspring glutathione peroxidase 4 genotype, and childhood asthma. J Allergy Clin Immunol 2015;135:1083-5.e3. [Crossref]
- Ling Y, Yin-Shi G, Hong-Wei S, et al. Oxidative stress status in umbilical cord blood from neonates born to mothers with atopic asthma. J Matern Fetal Neonatal Med 2014;27:192-6. [Crossref]
- Geraghty P, Hardigan AA, Wallace AM, et al. The glutathione peroxidase 1-protein tyrosine phosphatase 1B-protein phosphatase 2A axis. A key determinant of airway inflammation and alveolar destruction. Am J Respir Cell Mol Biol 2013;49:721-30. [Crossref]
- Bansal P, Saw S, Govindaraj D, et al. Intranasal administration of a combination of choline chloride, vitamin C, and selenium attenuates the allergic effect in a mouse model of airway disease. Free Radic Biol Med 2014;73:358-65. [Crossref]
- Peh HY, Ho WE, Cheng C, et al. Vitamin E Isoform gamma-Tocotrienol Downregulates House Dust Mite-Induced Asthma. J Immunol 2015;195:437-44. [Crossref]
- Harada T, Yamasaki A, Chikumi H, et al. gamma-Tocotrienol reduces human airway smooth muscle cell proliferation and migration. Pulm Pharmacol Ther 2015;32:45-52. [Crossref]
- Sivaramakrishnan V, Niranjali Devaraj S. Morin regulates the expression of NF-kappaB-p65, COX-2 and matrix metalloproteinases in diethylnitrosamine induced rat hepatocellular carcinoma. Chem Biol Interact 2009;180:353-9. [Crossref]
- Kapoor R, Kakkar P. Protective role of morin, a flavonoid, against high glucose induced oxidative stress mediated apoptosis in primary rat hepatocytes. PLoS One 2012;7:e41663. [Crossref]
- Franova S, Kazimierova I, Pappova L, et al. Bronchodilatory, antitussive and anti-inflammatory effect of morin in the setting of experimentally induced allergic asthma. J Pharm Pharmacol 2016;68:1064-72. [Crossref]
- Luczak ED, Anderson ME. CaMKII oxidative activation and the pathogenesis of cardiac disease. J Mol Cell Cardiol 2014;73:112-6. [Crossref]
- Freund-Michel V, Guibert C, Dubois M, et al. Reactive oxygen species as therapeutic targets in pulmonary hypertension. Ther Adv Respir Dis 2013;7:175-200. [Crossref]
- Di Gennaro A, Haeggstrom JZ. The leukotrienes: immune-modulating lipid mediators of disease. Adv Immunol 2012;116:51-92. [Crossref]
- Kirtikar KR, Basu BD. Indian medicinal plants. Deraduhun: International Book Distributors, 1996:2445.
- Li F, Liang D, Yang Z, et al. Astragalin suppresses inflammatory responses via down-regulation of NF-kappaB signaling pathway in lipopolysaccharide-induced mastitis in a murine model. Int Immunopharmacol 2013;17:478-82. [Crossref]
- Soromou LW, Chen N, Jiang L, et al. Astragalin attenuates lipopolysaccharide-induced inflammatory responses by down-regulating NF-kappaB signaling pathway. Biochem Biophys Res Commun 2012;419:256-61. [Crossref]
- Sahiner UM, Birben E, Erzurum S, et al. Oxidative stress in asthma. World Allergy Organ J 2011;4:151-8. [Crossref]
- Ghezzi P. Role of glutathione in immunity and inflammation in the lung. Int J Gen Med 2011;4:105-13. [Crossref]
- Kato T, Tada-Oikawa S, Takahashi K, et al. Endocrine disruptors that deplete glutathione levels in APC promote Th2 polarization in mice leading to the exacerbation of airway inflammation. Eur J Immunol 2006;36:1199-209. [Crossref]
- Rahman I, Biswas SK, Kode A. Oxidant and antioxidant balance in the airways and airway diseases. Eur J Pharmacol 2006;533:222-39. [Crossref]
- Sies H, Sharov VS, Klotz LO, et al. Glutathione peroxidase protects against peroxynitrite-mediated oxidations. A new function for selenoproteins as peroxynitrite reductase. J Biol Chem 1997;272:27812-7. [Crossref]
- Jeong DW, Yoo MH, Kim TS, et al. Protection of mice from allergen-induced asthma by selenite: prevention of eosinophil infiltration by inhibition of NF-kappa B activation. J Biol Chem 2002;277:17871-6. [Crossref]
- Sen CK, Khanna S, Roy S. Tocotrienols in health and disease: the other half of the natural vitamin E family. Mol Aspects Med 2007;28:692-728. [Crossref]
- Cook-Mills JM, Abdala-Valencia H, Hartert T. Two faces of vitamin E in the lung. Am J Respir Crit Care Med 2013;188:279-84. [Crossref]