Advanced bronchoscopic techniques in diagnosis and staging of lung cancer
Introduction
Bronchoscopy plays an important role in the diagnosis and staging of lung cancer. Despite the advances in surgical treatment and discovery of new medications, lung cancer is still the leading cause of death from malignant diseases worldwide (1-6). Fast and accurate detection and staging are important in order to make most adequate treatment plan for patients with lung cancer.
Bronchoscopic imaging techniques, such as autofluorescence imagining (AFI) or narrow band imaging (NBI), facilitate detection of premalignant lesions and early lung cancer. This might be of key importance for research in carcinogenesis. Natural course of premalignant lesions is uncertain. There are several therapeutic options for these lesions including bronchoscopic treatment; surgery or follow up, having in mind that large number of premalignant lesions will disappear spontaneously. Several clinical trials are aimed at evaluation of clinical course of premalignant lesions, trying to identify clinical characteristics of lesions progressing to invasive malignancy. However, detection rate of premalignant lesions under conventional bronchoscopic evaluation is low and most often accidental. Both AFI and NBI showed supremacy in detection of premalignant lesions over conventional white light videobronchoscopy. These bronchoscopic imaging techniques could be used in everyday practice for detection of synchronous tumors, for follow up after curative surgery, evaluation of vascularisation after lung transplantation or for evaluation of tumor margins (1-6).
Linear endobronchial ultrasound (EBUS) showed to be valuable in evaluation of mediastinal lymph nodes during staging of lung cancer. Lymph node stations 2, 4, 7, 10, and 11 are easily accessible for EBUS guided transbronchial needle aspiration (TBNA). EBUS-TBNA, as a minimally invasive procedure, proven to be complementary to mediastinoscopy in staging of lung cancer. However, in order to perform adequate staging of lymph nodes in lung cancer, at least three stations must be evaluated, which is often not the case. This issue must be accounted in all future investigations of EBUS-TBNA as diagnostic procedure in mediastinal staging. EBUS guided TBNA is also very good alternative in mediastinal restaging after mediastinoscopy and neoadjuvant chemo-radiotherapy. This technique decreases risks of mediastinoscopy in restaging, providing high diagnostic accuracy. Combination of EBUS-TBNA and rapid on site cytology (ROSE) can further increase diagnostic yield of the procedure. Radial EBUS with guiding sheath and miniature ultrasound probes provides high diagnostic yield in diagnosis of peripheral pulmonary lesions. Procedural risks of radial EBUS transbronchial biopsy are reasonably lower than that of transthoracic needle aspiration. Often, radial EBUS guided biopsy provides sufficient amount of tissue suitable for extensive genetic testing required nowadays in diagnostics of lung cancer. This technique could be used for assessment of invasive depth of malignant lesions in central airways. Such assessment could be beneficial for patients who are not candidates for surgery, but could be treated with interventional pulmonology techniques. Radial EBUS can be combined with other navigational bronchoscopic procedures such as electromagnetic navigation bronchoscopy (EMN) (i·Logic™ Lung Navigation System, COVIDIEN, Mansfield, MA, USA) or LungPoint Virtual Bronchoscopic Navigation system (VBN) (Bronchus Medical Inc., Mountain View, CA, USA). Published data suggest that diagnostic yield of both radial EBUS and navigational bronchoscopy, for small peripheral pulmonary lesions, could be improved if techniques are combined (7-11).
It is no longer questions are these new technologies usable in everyday practice. The question is: when, which and how will these techniques be used?
Many issues are still to be addressed: high initial price of the technology and its availability in selected high volume centers of excellence (or unavailability on large scale basis), training standards across respiratory endoscopy units, provision of sufficient data from multicentre randomized trials, reimbursement of costs, etc. (12).
However, in spite of many open issues, these technologies are more and more available and used in many bronchoscopic suites.
Autofluorescence bronchoscopy (AFB)
First systems for autofluorescence examination of bronchial mucosa were robust, impractical and hardly appropriate for everyday use in diagnostic bronchoscopy. In past decade these mainly research tools became a part of everyday equipment of bronchoscopy units. New systems for autofluorescence bronchoscopy, such as Autofluorescence Imaging-AFI (Olympus Co, Tokyo, Japan), SAFE-3000 (Pentax Co, Japan), D-Light AF system (Karl Storz, Tuttlingen, Germany) and Onco-LIFE (Xillix Technologies Corp. Richmond, Canada), produce clear, high quality images of bronchial mucosa. These systems represent new generation autofluorescence videobronchoscopy devices, mainly with video-chip based videobronchoscopes. Images generated by the new systems are clear, reproducible, and easy to interpret. In the mentioned systems autofluorescence and white light videobronchoscopy are incorporated into one bronchoscope. This allows easy and convenient transition from one mode to another during endoscopic examination of bronchial tree (13-17).
The role of AFB was majorly investigated in detection of premalignant lesions of bronchial mucosa. Most of the results confirmed higher sensitivity for detection of precancerous bronchial lesions, when compared to white light bronchoscopy (WLB) alone. However, it is known that specificity of AFB for detection of premalignant lesions remains low. Reported specificity varies in different trials, one of the causes of variation could be in different systems used, but other could lay in different prevalence of lesions in investigated populations (13-17).
Specificity of AFB in follow up of surgical margins after curative surgery, detection of synchronous lesions or in evaluation of lung cancer extension specificity of AFB is significantly higher. One of the open issues on AFB is question of its usability in bronchoscopic lung cancer screening. Results of published series did not support the general use of AFB as a screening tool for lung cancer. These results suggest that AFB could be used in groups of patients at high risk for lung cancer. Some authors, however, do not recommend its use not even in high risk populations. However, AFB is more than efficient in evaluation of bronchial mucosa in certain risk groups. Patients who can benefit are: long term chronic obstructive pulmonary disease (COPD) patients, patients with prolonged cough, heavy smokers, and patients with known head and neck malignancy. In these groups indication for AFB should be made along with existing indication for bronchoscopy. In recent years the indications for AFB have widened. This technique might find its place in routine bronchoscopy. It can be used for evaluation of tumor extension for follow up after surgical resection or for follow up after photodynamic therapy of centrally located early lung cancer. Sharp learning curve and clear distinction between normal and pathologically altered mucosa; make this technology acceptable for all bronchoscopists. New hardware and software improvements such as addition of backscattered light analysis, ultraviolet spectra, fluorescence-reflectance or dual digital systems could improve diagnostic yield of this technology (13-17).
Technology overview
The cellular basis for the fluorescence phenomenon is in the substances called fluorophores. Fluorophores are variously concentrated within tissues, showing organ typical patterns. Some of the fluorophores are tryptophane, collagen, elastine and porphyrine. Intensity of autofluorescence emission generated by the fluorophores depends on their concentration, their maximal absorption and remission and of course on the characteristics of the light source. It is believed that the concentration of the fluorophores in the pathologically altered bronchial mucosa is different than in normal bronchial mucosa (13,14). On the AFI examination normal mucosa appears green, while pathologically altered mucosa is magenta or red-brownish (Figures 1,2,3). Classification of endobronchial findings during autofluorescence bronchoscopy is given in Table 1.
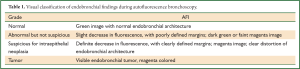
Full Table
Modern day AFB systems are based on videobronchoscopy and charged couple device (CCD) chips. One of the systems authors have more experience with is AFI by Olympus Co. (Tokyo, Japan). This system is comprised of AF videobronchoscope (BF F260), video processor unit EVIS LUCERA SPECTRUM (CV-260SL), and xenon light source CLV-260SL. The AF videobronchoscopy image could be presented on a 19 inch LCD monitor OEV-191, but it can also be produced on any other (HD) monitor. The EVIS LUCERA SPECTRUM video processor unit is based on black and white CCD chip technology, in which color separation is achieved through the use of an RGB color filter equipped with the light source unit. The AFI system utilizes high resolution imaging of the CCD chip at the distal end of the EVIS LUCERA SPECTRUM BF-F260 videobronchoscope. In addition to the blue excitation light (wavelength 390-440 nm) that causes autofluorescence, the AFI system uses green light (540-550 nm) that is mainly absorbed by hemoglobin. These two kinds of light, captured by the CCD at the tip of the bronchoscope are converted into electrical signals. The barrier filter in front of the CCD decreases excessive blue light in order to detect the weak autofluorescence signal (13,14).
Clinical data on AFB
Two important meta-analyses on AFB were published in last few years. Chen and associates performed a meta-analysis in order to re-examine the diagnostic efficiency of AFB compared with WLB (18). Included studies had to have definitive histology as diagnostic standard, and provided sufficient data to construct 2×2 table for assessing diagnostic yield of AFB for detection of lung cancer and premalignant lesions. Fifteen sets of data, derived from 14 studies were found to be suitable for analysis. Pooled sensitivity, specificity, positive likelihood ratio and negative likelihood ratio for AFB were 0.90 (95% CI: 0.84-0.93), 0.56 (95% CI: 0.45-0.66), 2.0 (range, 1.7-2.5) and 0.18 (range, 0.13-0.26), respectively. Pooled diagnostic odds ratio was found to be 11. Pooled sensitivity, specificity, positive likelihood ratio and negative likelihood ratio for WLB were respectively: 0.66 (95% CI: 0.58-0.73), 0.69 (95% CI: 0.57-0.79), 2.1 (1.6-2.9) and 0.50 (0.43-0.58), while diagnostic odds ratio was 4. In this meta-analysis contribution of differences in excitation light source, histological criteria and biopsy strategy was not counted as a covariate. The authors concluded that AFB was superior to conventional WLB in detecting lung cancer and preneoplastic lesions. In Sun’s meta-analysis (19) the main objective was comparison between accuracy of AFB combined with WLB versus WLB alone in the diagnosis of lung cancer. The authors searched several databases in time period from January 1990 to October 2010. Twenty one studies involving 3,266 patients were ultimately analyzed in Sun’s meta-analysis. The pooled relative sensitivity on a per lesion basis to detect premalignant lesions and invasive cancer was for AFB+WLB 2.04 (95% CI: 1.72-2.42) and for WLB alone 1.15 (95% CI: 1.05-1.26), and it was statistically significant (P=0.003). The pooled sensitivity on a per-lesion basis of AFB + WLB versus WLB to detect invasive cancer was 94.71% and 88.53%, respectively, while the pooled specificity on a per-lesion basis of AFB+WLB versus WLB alone was 60.94% and 79.7%, respectively. The corresponding values of relative specificity on per-lesion basis of AFB+WLB versus WLB alone was 0.65 and was statistically significant (P<0.00001). The main results of this meta-analysis showed that combination of AFB + WLB significantly improves sensitivity in detection of premalignant lesions, even though this advantage seemed much less in detection of invasive lung cancer.
Diagnostic yield of AFB in detection of pre-malignant lesions could be highly dependent on several factors such as the type of AFB system, the grade of the lesion, incidence of lesions in investigated population and bronchoscopists experience. Sensitivity and specificity of AFB in detection of premalignant lesions in some of the largest trials is given in Table 2.
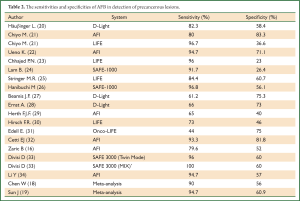
Full Table
The question of low specificity remains one of the major issues of AFB. It is believed that software enhancements such as spectral analysis can improve the specificity of AFB. Several other optical enhancements were tested in clinical trial in order to reevaluate diagnostic yield of AFB. Combination of AFB with other optical bronchoscopic imaging techniques could improve diagnostic performance of AFB (35-37)
Narrow Band Imaging (NBI)
NBI is bronchoscopic optical enhancement technology which enables thorough and detailed examination of submucosal microcapillary grid. Combination of magnification videobronchoscopy and NBI showed great potential in detection of precancerous and cancerous lesions of the bronchial mucosa. This technology enables endoscopists to obtain more detailed information on the pathologically altered mucosal and submucosal blood vessels of endoscopically accessible organs such as oro-pharynx, oesophagus, stomach, duodenal ampulla, lung, colon, bronchi and bladder. Pathological patterns of submucosal blood vessels in bronchial mucosa are known as Shibuya’s descriptors (dotted, tortuous and abrupt ending blood vessels) (Figures 4-6). Most often these patterns appear together, however it is possible to determine predomination of one pattern what could be related to specific histology of lung cancer. Abnormal vascular patterns which are not pathological are known as the “background noise”. These “background noise” patterns are shown on Figures 7-9. NBI could be more efficient in the detection of precancerous lesions, especially angiogenic squamous dysplasia (ASD), than the white light videobronchoscopy alone. However, this technology could be successfully used for evaluation of tumor margins, follow up after curative surgery or detection of synchronous tumors. The learning curve for NBI videobronchoscopy is sharp. This makes the NBI useful even when it is used by an inexperienced bronchoscopist. It is believed that the combination of NBI with autofluorescence videobronchoscopy will give better results in lung cancer detection (38-40).
NBI creates clear and sharp images of the blood vessels in the surface layers of bronchial mucosa. This technique could reveal minute patterns on mucosal membranes when the tissue is irradiated with the two narrow wave bands of light. The NBI system uses a blue narrow band, with the wave length ranging from 390-445 nm, for imaging the capillaries of the surface mucosal layer. A green narrow band (of the wave length from 530-550 nm) is used to visualize the thick blood vessels inside the membranes. This optical enhancement enables creation of clear image of the target tissue and provides a better contrast on the mucosal surface, reduces examination time, and eliminates futile biopsies (38-40).
Technology overview
One of the most commonly used videoendoscopy systems for NBI videobronchoscopy is based on the color CCD chip that has several tiny color filters in each pixel (EVIS EXERA II, Olympus Inc, Tokyo, Japan). However, EVIS LUCERA SPECTRUM videoendoscopy system (Olympus Inc, Tokyo, Japan) based on the black and white CCD chip, also supports NBI technology. There are brand new systems emerging on the market, such as EXERA III which also support this technique (38,39).
The EXERA II system is equipped with a digital zoom at 1.2 and 1.5 times magnification. However, the high definition endoscope (HD) possesses a physical zoom that allows the tip of the bronchoscope to be advanced up to 2 mm away from the mucosal surface without decreasing the resolution. This combination allows 50-fold magnification. It must be underlined that these numbers are limited in reality by numerous factors affecting magnification, from the tissue characteristics, to the quality of the monitor. The combination of the high definition technology (HDTV) and NBI provides the top quality performance and the clearest images in observing the mucosal surface (39).
EVIS EXERA II system is equipped with double band filter (415 and 540 nm) which is removed from the optical axis under the normal observation. Under the NBI, the filter splits the light into two bands (415 and 540 nm) and the split light bands illuminate the mucosa. To create the color image on the monitor, the light must be outputted to R, G and B channels. In order to accomplish a high visibility of the blood vessels, the superficial capillary grid must be reproduced in the black and white pattern, and relatively thicker vessels in the deeper part of the mucosa must be highlighted with a different color pattern. The colors must be allocated according to the human visual perception. That is why the 415 nm wavelength band is allocated to B and G channels and the superficial capillary grid is visualized in the brownish color. The 540 nm band is allocated to the R channel and the deeper layer capillaries are visualized as greenish-blue or more often cyan. Bleeding is shown in the black, tar-like color, because the light rays of 415 nm and 540 nm are mainly absorbed by hemoglobin, and there is no reflected light from that surface (39,40).
Clinical data on NBI
The study that introduced magnification bronchoscopy and NBI in respiratory medicine investigated the subepithelial patterns in bronchial dysplasia using a high magnification videobronchoscope. Sibuya et al. (41) developed a method of high magnification videobronchoscopy that enabled thorough visualization of subepithelial vascular patterns of the bronchial mucosa. The authors included 31 patients with sputum cytology suspected or positive for malignancy. The study protocol included the examination with conventional white light bronchoscopy, followed by autofluorescence bronchoscopy (LIFE system). Having identified the sites with pathological fluorescence, high magnification videobronchoscopy was used to examine the microvascular network at the sites of normal and abnormal fluorescence and the images were compared to the biopsy sample established pathological diagnosis. The results showed that the regular vascular patterns seen in 20/22 abnormal sites (on fluorescence bronchoscopy) were associated with bronchitis. On the other hand, the vascular networks with increased vessel growth and complex networks of tortuous capillaries observed in 15 of 21 fluorescently abnormal sites correlated to bronchial dysplasia. There was a significant difference (P<0.0001) between bronchitis and dysplasia. The study concluded that magnification videobronchoscopy enabled differentiation between bronchitis and dysplasia.
In 2003, Shibuya et al. published the study that combined magnification videobronchoscopy and narrow band imaging (42). The aim of that study was to investigate the usefulness of magnification videobronchoscopy and NBI in examining ASD. The study population included heavy smokers with a high risk of lung cancer. There were 48 patients with the sputum cytology suspected or positive for a malignancy included in the study. The bronchoscopic exploration started with the white light bronchoscopy, followed by autofluorescence bronchoscopy. This study found significant correlation between the frequencies of the dotted vessels visualized by NBI and the tissues pathologically confirmed as ASD.
One of the most valuable publications on NBI videobronchoscopy was published by Herth et al. (43). The authors evaluated the diagnostic yields of NBI individually and in combination with WLB and AFI. The primary aim was to determine the value of NBI as compared to AFI and WLB, not to examine the value of bronchoscopy in the early lung cancer detection. The relative sensitivities of AFI and NBI when compared to WLB were 3.7 and 3.0 respectively, both were statistically significant. NBI showed significantly higher specificity when compared to AFI, but without significance for sensitivity. Combining the findings of WLB to either AFI or NBI produced no change of the relative sensitivity of any of the procedures. The relative sensitivity of the combined AFI and NBI was 4.0, but without significance when compared to AFI or NBI alone. In another words, the combination of AFI and NBI induced no significant increase of the diagnostic yield. The sequence of the techniques had no impact on the findings obtained. In the conclusion, the authors stated that detection of angiogenic squamous dysplasia by NBI technology might be advantageous over AFI screening in detection of early stage lung cancer. It was confirmed that NBI could increase specificity without compromising the sensitivity.
In several studies published by our group we investigated diagnostic efficiency of NBI in detection of tumor margins and intrabronchial extension of invasive lung cancer. In these trials NBI was not used for diagnosis of gross tumorous masses, it was used solely for investigations of tumor margins. In pilot trial we found the sensitivity, specificity, PPV and NPV of NBI for the visualization of the tumor margins to be 97.8%, 85%, 93.7% and 94.4%, respectively. The sensitivity, specificity, PPV and NPV of the white light videobronchoscopy was significantly lower, 92.3%, 60%, 69.6% and 88.9% respectively. Larger confirmatory trial followed. In the final study, the specificity, sensitivity, PPV and NPV of 85.6%, 95%, 84% and 95.6%, respectively were obtained (38,39). In one of the latest trials we investigated relationship between vascular pattern and lung cancer histology. Dotted blood vessels were significantly (P<0.000) associated with adenocarcinoma, identified in 68.4% adenocarcinoma and 31.6% squamous cell cancers (SCC). Tortuous blood vessels were identified in 72% SCC, 8% adenocarcinoma, 12% small cell lung cancer (SCLC) and 8% of large cell lung cancer (LC). Tortuous blood vessels were significantly (P<0.000) associated with SCC. Abrupt ending vessels were identified in 81% SCC, 14.3% SCLC and 4.8% adenocarcinoma, and were significantly associated (P<0.000) with SCC. This study concluded that dotted visual pattern of blood vessels identified during NBI videobronchoscopy is highly suggest adenocarcinoma histology of lung cancer. Tortuous and abrupt ending blood vessels visualized under NBI videobronchoscopy suggest squamous cell histology of lung cancer (44).
The place of NBI in the assessment of premalignant and malignant lesions has been defined: this technology is efficient, specific and sensitive enough in the detection of early lung cancer. The NBI is sensitive and specific enough to identify dysplasia, carcinoma in situ and invasive lung cancer. It is a technology with a potential to contribute to the investigations and understanding of neo-angiogenesis as a crucial part of carcinogenesis of lung tumors. The role of NBI in the investigations of vascular anomalies in COPD and bronchial asthma is a challenging field for pulmonologists and bronchologists. It would be wise to attempt at investigating the blood vessel patterns in patients with COPD and correlating the findings to the responsiveness to therapy and rate of exacerbations. One of the emerging indications for the NBI in respiratory endoscopy is the quantitative assessment of the airway vascularity after lung transplantation. This will be one of the major advantages NBI can offer in relation to other imaging techniques (40-45).
Endobronchial ultrasound (EBUS)
EBUS has broadened the diagnostic potential of bronchoscopy. EBUS exists in two forms: radial and linear EBUS. Both types use a transducer that produce and receive the sound waves. A processor integrates the sound waves reflected by tissues to generate the two-dimensional ultrasound image. Radial EBUS probes facilitate not only guided TBNA and guided biopsies of peripheral lung lesions but also evaluation of airway wall structure. Linear EBUS, on the other hand, facilitates TBNA of mediastinal, hilar, and intrapulmonary lymph nodes under real-time ultrasound control with a high diagnostic yield (46-50). Linear EBUS consists of dedicated bronchoscope with US probe at the distal tip and ultrasonic center. This EBUS bronchoscope incorporates color and power Doppler, with corresponding high resolution flow modes, to allow better detection of vascular involvement before lymph node sampling. Ultrasound and the conventional bronchoscopic images can be viewed simultaneously. TBNA is performed with a specially designed needle within a catheter device, which is passed through the working channel of the bronchoscope. Figures 10 and 11 show the transbronchial needle inside the lymph node. One of the major advantages of this technique is real-time aspiration of the mediastinal lymph nodes. Depending on the diameter of sampling needle, cytology or histology samples can be obtained and used for diagnosis. In recent years new sampling instruments are under development and evaluation. One of the potentially most useful will be TBNA forceps, developed by Herth et al., which will allow better quality of histology samples. It has been shown that EBUS TBNA might be complementary to mediastinoscopy in staging of lung cancer. After localization of the peripheral lung lesion with the radial EBUS probe, the probe must be pulled out of the working channel of the bronchoscope. Sampling tool (forceps) could then be introduced and placed in the lesion in order to take samples. Fluoroscopy is often used to verify the position of the forceps. Development of new smaller probes with guiding catheters solves the problem of navigation. The probe is placed into the catheter and slided through working channel of the bronchoscope. Once the place of tumor was visualized by radial EBUS probe, the probe is being removed while the catheter remains in place, sampling tool is than inserted through the catheter (Figures 12,13) (51,52) (Table 3).
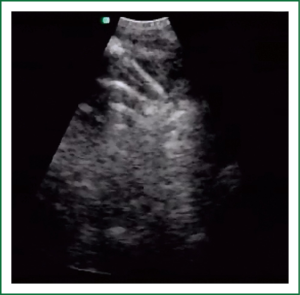
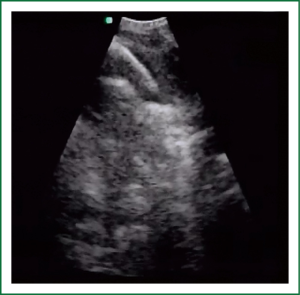
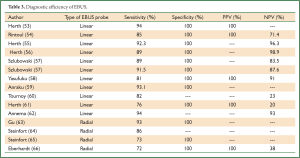
Full Table
Conclusions
Advanced bronchoscopic techniques are helpful in everyday diagnostic workup of lung cancer. Imaging technologies provided more accurate visualization of pathological alterations in bronchial mucosa, enabling more adequate assessment of endobronchial processes. On the other hand EBUS technology provides minimally invasive highly accurate evaluation of the mediastinum which might be complementary to mediastinoscopy. Radial EBUS probes are beneficial in diagnosis in peripheral lung lesions, together with rapid on site cytology, radial EBUS shows high diagnostic yield. In combination with navigational technologies that yield can be further increased.
Acknowledgements
Disclosure: The authors declare no conflict of interest.
References
- McErlean A, Ginsberg MS. Epidemiology of lung cancer. Semin Roentgenol 2011;46:173-7. [PubMed]
- Wahidi MM, Herth FJ, Ernst A. State of the art: interventional pulmonology. Chest 2007;131:261-74. [PubMed]
- Sutedja G. New techniques for early detection of lung cancer. Eur Respir J Suppl 2003;39:57s-66s. [PubMed]
- Colt HG, Murgu SD. Interventional bronchoscopy from bench to bedside: new techniques for early lung cancer detection. Clin Chest Med 2010;31:29-37. [PubMed]
- Perin B, Zarić B, Becker HD, et al. Interventional pulmonology. In: Jeremic B. eds. Medical Radiology, 2011, Advances in Radiation Oncology in Lung Cancer. Heidelberg: Springer Verlag, 2011:45-52.
- Yarmus L, Feller-Kopman D. Bronchoscopes of the twenty-first century. Clin Chest Med 2010;31:19-27. [PubMed]
- Yasufuku K. Early diagnosis of lung cancer. Clin Chest Med 2010;31:39-47. [PubMed]
- Wang KP, Browning R. Transbronchial needle aspiration with or without endobronchial ultrasound. Thoracic Cancer 2010;1:87-93.
- Cetinkaya E, Gunluoglu G, Ozgul A, et al. Value of real-time endobronchial ultrasound-guided transbronchial needle aspiration. Ann Thorac Med 2011;6:77-81. [PubMed]
- Parmaksız ET, Caglayan B, Salepci B, et al. The utility of endobronchial ultrasound-guided transbronchial needle aspiration in mediastinal or hilar lymph node evaluation in extrathoracic malignancy: Benign or malignant? Ann Thorac Med 2012;7:210-4. [PubMed]
- Lim E., Shah PL. Role of endobronchial ultrasound-guided transbronchial needle aspiration for mediastinal lymph node staging of lung cancer. Thoracic Cancer 2010;1:2-3.
- Bolliger CT, Mathur PN, Beamis JF, et al. ERS/ATS statement on interventional pulmonology. European Respiratory Society/American Thoracic Society. Eur Respir J 2002;19:356-73. [PubMed]
- Zaric B, Perin B, Becker HD, et al. Autofluorescence imaging videobronchoscopy in the detection of lung cancer: from research tool to everyday procedure. Expert Rev Med Devices 2011;8:167-72. [PubMed]
- Zaric B, Perin B, Carapic V, et al. Diagnostic value of autofluorescence bronchoscopy in lung cancer. Thorac Cancer 2013;4:1-8.
- Zaric B, Canak V, Stojanovic G, et al. Autofluorescence videobronchoscopy (AFI) for the assessment of tumor extension in lung cancer. Technol Cancer Res Treat 2009;8:79-84. [PubMed]
- Zaric B, Perin B, Becker HD, et al. Combination of narrow band imaging (NBI) and autofluorescence imaging (AFI) videobronchoscopy in endoscopic assessment of lung cancer extension. Med Oncol 2012;29:1638-42. [PubMed]
- Zaric B, Becker HD, Perin B, et al. Autofluorescence imaging videobronchoscopy improves assessment of tumor margins and affects therapeutic strategy in central lung cancer. Jpn J Clin Oncol 2010;40:139-45. [PubMed]
- Chen W, Gao X, Tian Q, et al. A comparison of autofluorescence bronchoscopy and white light bronchoscopy in detection of lung cancer and preneoplastic lesions: a meta-analysis. Lung Cancer 2011;73:183-8. [PubMed]
- Sun J, Garfield DH, Lam B, et al. The value of autofluorescence bronchoscopy combined with white light bronchoscopy compared with white light alone in the diagnosis of intraepithelial neoplasia and invasive lung cancer: a meta-analysis. J Thorac Oncol 2011;6:1336-44. [PubMed]
- Häussinger K, Becker H, Stanzel F, et al. Autofluorescence bronchoscopy with white light bronchoscopy compared with white light bronchoscopy alone for the detection of precancerous lesions: a European randomized controlled multicenter trial. Thorax 2005;60:496-503. [PubMed]
- Chiyo M, Shibuya K, Hoshino H, et al. Effective detection of bronchial preinvasive lesions by a new autofluorescence imaging bronchovideoscope system. Lung Cancer 2005;48:307-13. [PubMed]
- Ueno K, Kusunoki Y, Imamura F, et al. Clinical experience with autofluorescence imaging system in patients with lung cancers and precancerous lesions. Respiration 2007;74:304-8. [PubMed]
- Chhajed PN, Shibuya K, Hoshino H, et al. A comparison of video and autofluorescence bronchoscopy in patients at high risk of lung cancer. Eur Respir J 2005;25:951-5. [PubMed]
- Lam B, Wong MP, Fung SL, et al. The clinical value of autofluorescence bronchoscopy for the diagnosis of lung cancer. Eur Respir J 2006;28:915-9. [PubMed]
- Stringer MR, Moghissi K, Dixon K. Autofluorescence bronchoscopy in volunteer asymptomatic smokers. Photodiagnosis Photodyn Ther 2008;5:148-52. [PubMed]
- Hanibuchi M, Yano S, Nishioka Y, et al. Autofluorescence bronchoscopy, a novel modality for the early detection of bronchial premalignant and malignant lesions. J Med Invest 2007;54:261-6. [PubMed]
- Beamis JF Jr, Ernst A, Simoff M, et al. A multicenter study comparing autofluorescence bronchoscopy to white light bronchoscopy using a non-laser light stimulation system. Chest 2004;125:148S-9S. [PubMed]
- Ernst A, Simoff MJ, Mathur PN, et al. D-light autofluorescence in the detection of premalignant airway changes: a muticenter trial. J Bronchol 2005;12:133-8.
- Herth FJ, Eberhardt R, Anantham D, et al. Narrow-band imaging bronchoscopy increases the specificity of bronchoscopic early lung cancer detection. J Thorac Oncol 2009;4:1060-5. [PubMed]
- Hirsch FR, Prindiville SA, Miller YE, et al. Fluorescence versus white-light bronchoscopy for detection of preneoplastic lesions: a randomized study. J Natl Cancer Inst 2001;93:1385-91. [PubMed]
- Edell E, Lam S, Pass H, et al. Detection and localization of intraepithelial neoplasia and invasive carcinoma using fluorescence-reflectance bronchoscopy: an international, multicenet clinical trial. J Thorac Oncol 2009;4:49-54. [PubMed]
- Cetti EJ, Nicholson AG, Singh S, et al. An evaluation of a videobronchoscopy-based autofluorescence system in lung cancer. Eur Respir J 2010;35:1185-7. [PubMed]
- Divisi D, Di Tommaso S, De Vico A, et al. Early diagnosis of lung cancer using a SAFE-3000 autofluorescence bronchoscopy. Interact Cardiovasc Thorac Surg 2010;11:740-4. [PubMed]
- Li Y, Li X, Sui XZ, et al. Comparison of the autofluorescence bronchoscope and the white light bronchoscope in airway examination. Chin J Cancer 2010;29:1018-22. [PubMed]
- Hüttenberger D, Gabrecht T, Wagnières G, et al. Autofluorescence detection of tumors in the human lung--spectroscopical measurements in situ, in an in vivo model and in vitro. Photodiagnosis Photodyn Ther 2008;5:139-47. [PubMed]
- Gabrecht T, Glanzmann T, Freitag L, et al. Optimized autofluorescence bronchoscopy using additional backscattered red light. J Biomed Opt 2007;12:064016. [PubMed]
- Gabrecht T, Radu A, Grosjean P, et al. Improvement of the specificity of cancer detection by autofluorescence imaging in the tracheo-bronchial tree using backscattered violet light. Photodiagnosis Photodyn Ther 2008;5:2-9. [PubMed]
- Bojan Z, Branislav P, Aleksandra J, et al. Influence of narrow band imaging (NBI) videobronchoscopy on the assessment of central lung cancer extension and therapeutic decision. Cancer Invest 2009;27:918-23. [PubMed]
- Zaric B, Becker HD, Perin B, et al. Narrow band imaging videobronchoscopy improves assessment of lung cancer extension and influences therapeutic strategy. Jpn J Clin Oncol 2009;39:657-63. [PubMed]
- Zaric B, Perin B. Use of narrow-band imaging bronchoscopy in detection of lung cancer. Expert Rev Med Devices 2010;7:395-406. [PubMed]
- Shibuya K, Hoshino H, Chiyo M, et al. Subepithelial vascular patterns in bronchial dysplasias using a high magnification bronchovideoscope. Thorax 2002;57:902-7. [PubMed]
- Shibuya K, Hoshino H, Chiyo M, et al. High magnification bronchovideoscopy combined with narrow band imaging could detect capillary loops of angiogenic squamous dysplasia in heavy smokers at high risk for lung cancer. Thorax 2003;58:989-95. [PubMed]
- Herth FJ, Eberhardt R, Anantham D, et al. Narrow-band imaging bronchoscopy increases the specificity of bronchoscopic early lung cancer detection. J Thorac Oncol 2009;4:1060-5. [PubMed]
- Zaric B, Perin B, Stojsic V, et al. Relation between vascular patterns visualized by Narrow Band Imaging (NBI) videobronchoscopy and histological type of lung cancer. Med Oncol 2013;30:374. [PubMed]
- Vincent BD, Fraig M, Silvestri GA. A pilot study of narrow-band imaging compared to white light bronchoscopy for evaluation of normal airways and premalignant and malignant airways disease. Chest 2007;131:1794-9. [PubMed]
- Dincer HE. Linear EBUS in staging non-small cell lung cancer and diagnosing benign diseases. J Bronchology Interv Pulmonol 2013;20:66-76. [PubMed]
- Groth SS, Whitson BA, D’Cunha J, et al. Endobronchial ultrasound-guided fine-needle aspiration of mediastinal lymph nodes: a single institution’s early learning curve. Ann Thorac Surg 2008;86:1104-9; discussion 1109-10. [PubMed]
- Garcia-Olive I, Sanz-Santos J, Andreo F, et al. Application of real time endobronchial ultrasound-guided transbronchial needle aspiration for lung cancer staging. Zhongguo Fei Ai Za Zhi 2010;13:406-9. [PubMed]
- Fielding D, Bashirzadeh F, Nguyen P, et al. Review of the role of EBUS-TBNA for the pulmonologist, including lung cancer staging. Zhongguo Fei Ai Za Zhi 2010;13:410-7. [PubMed]
- Dango S, Guenter J, Passlick B. Endobronchial ultrasound-guided transbronchial needle aspiration and its role in non-small cell lung cancer: diagnostic impact and limitations. Thoracic Cancer 2010;1:70-6.
- Herth FJ. Endobronchial ultrasound-guided biopsy of coin lesions. Future Oncol 2007;3:273-5. [PubMed]
- Kokkonouzis I, Strimpakos AS, Lampaditis I, et al. The role of endobronchial ultrasound in lung cancer diagnosis and staging: a comprehensive review. Clin Lung Cancer 2012;13:408-15. [PubMed]
- Herth FJ, Eberhardt R, Vilmann P, et al. Real-time endobronchial ultrasound guided transbronchial needle aspiration for sampling mediastinal lymph nodes. Thorax 2006;61:795-8. [PubMed]
- Rintoul RC, Skwarski KM, Murchison JT, et al. Endobronchial and endoscopic ultrasound-guided real-time fine-needle aspiration for mediastinal staging. Eur Respir J 2005;25:416-21. [PubMed]
- Herth FJ, Ernst A, Eberhardt R, et al. Endobronchial ultrasound-guided transbronchial needle aspiration of lymph nodes in the radiologically normal mediastinum. Eur Respir J 2006;28:910-4. [PubMed]
- Herth FJ, Eberhardt R, Krasnik M, et al. Endobronchial ultrasound-guided transbronchial needle aspiration of lymph nodes in the radiologically and positron emission tomography-normal mediastinum in patients with lung cancer. Chest 2008;133:887-91. [PubMed]
- Szlubowski A, Kuzdzał J, Kołodziej M, et al. Endobronchial ultrasound-guided needle aspiration in the non-small cell lung cancer staging. Eur J Cardiothorac Surg 2009;35:332-5; discussion 335-6. [PubMed]
- Yasufuku K, Pierre A, Darling G, et al. A prospective controlled trial of endobronchial ultrasound-guided transbronchial needle aspiration compared with mediastinoscopy for mediastinal lymph node staging of lung cancer. J Thorac Cardiovasc Surg 2011;142:1393-400.e1.
- Anraku M, Pierre AF, Nakajima T, et al. Endobronchial ultrasound-guided transbronchial needle aspiration in the management of previously treated lung cancer. Ann Thorac Surg 2011;92:251-5; discussion 255. [PubMed]
- Tournoy KG, Rintoul RC, van Meerbeeck JP, et al. EBUS-TBNA for the diagnosis of central parenchymal lung lesions not visible at routine bronchoscopy. Lung Cancer 2009;63:45-9. [PubMed]
- Herth FJ, Annema JT, Eberhardt R, et al. Endobronchial ultrasound with transbronchial needle aspiration for restaging the mediastinum in lung cancer. J Clin Oncol 2008;26:3346-50. [PubMed]
- Annema JT, van Meerbeeck JP, Rintoul RC, et al. Mediastinoscopy vs endosonography for mediastinal nodal staging of lung cancer: a randomized trial. JAMA 2010;304:2245-52. [PubMed]
- Gu P, Zhao YZ, Jiang LY, et al. Endobronchial ultrasound-guided transbronchial needle aspiration for staging of lung cancer: a systematic review and meta-analysis. Eur J Cancer 2009;45:1389-96. [PubMed]
- Steinfort DP, Vincent J, Heinze S, et al. Comparative effectiveness of radial probe endobronchial ultrasound versus CT-guided needle biopsy for evaluation of peripheral pulmonary lesions: a randomized pragmatic trial. Respir Med 2011;105:1704-11. [PubMed]
- Steinfort DP, Khor YH, Manser RL, et al. Radial probe endobronchial ultrasound for the diagnosis of peripheral lung cancer: systematic review and meta-analysis. Eur Respir J 2011;37:902-10. [PubMed]
- Eberhardt R, Ernst A, Herth FJ. Ultrasound-guided transbronchial biopsy of solitary pulmonary nodules less than 20 mm. Eur Respir J 2009;34:1284-7. [PubMed]