Serendipity and innovation: history and evolution of transthoracic echocardiography
“I once read a silly fairy tale, called The Three Princes of Serendip: as their highnesses travelled, they were always making discoveries, by accidents and sagacity, of things which they were not in quest of... now do you understand Serendipity?”—Horace Walpole (January 28th, 1754)
“Happy accident”, “fortunate happenstance”, “pleasant surprise”. In one word, Serendipity.
The history of echocardiography is sprinkled with many interesting episodes and anecdotes showing that devoting your life to the pursuit of one goal is praiseworthy, and that a little luck goes a long way. Transthoracic echocardiography (TTE) has led to dramatic improvements in cardiovascular medicine, and is now the most widely used diagnostic cardiac test after electrocardiography (ECG). Standard echocardiographic examination allows the physician to obtain crucial information on cardiac structures, function, and hemodynamics in a remarkably quick and, most of all, totally non-invasive way.
As the worldwide recognized “father of echocardiography”, Harvey Feigenbaum, wrote (1), the origins of this technology date back to Curie and Curie (2,3), who first discovered piezoelectricity in 1880 while observing that an electric potential would be produced when mechanical pressure was exerted on a quartz crystal such as Rochelle salt (sodium potassium tartrate tetrahydrate).
World War II saw rapid developments and refinements with regard to the application of ultrasounds in naval and military radars. Nevertheless, ultrasound waves would soon find more peaceful uses with most of the early experimental studies performed by non-physicians like Wild in the 1950s (4).
The first real approach to what can be considered a “clinical echo” is credited to Herz and Edler (5) who published their seminal paper “The use of ultrasonic reflectoscope for the continuous recording of the movements of heart walls” in 1954. Hertz was able to locate a commercial ultrasonic reflectoscope which he placed on his chest and attributed the moving signal to cardiac motion: it was the first example of amplitude mode (A-mode) display (6).
Anecdotes exist about the origin of the word “echocardiography”. The first term used to describe this technique was introduced by Edler, who called it “ultrasound cardiography” (UCG). At that time, the only other ultrasound examination that had gained popularity was “echoencephalography”, which was performed to detect an echo from the midline of the brain to see if it was deviated by an intra-cranial mass. Consequently, Feigenbaum put forth that, “if the ultrasonic examination of the brain was echoencephalography, then the examination of the heart should be echocardiography” (1).
Another interesting story regarding how Edler correctly identified signals originating from the mitral valve came from his 1960’s work on mitral stenosis. In particular, he was studying a signal which he attributed to the posterior wall of the left atrium. He performed ultrasound evaluations on dying patients and marked the exact location and direction of the ultrasound beam. Once the patient died, “he stuck an ice pick into the chest in the direction of the ultrasonic beam. At autopsy, he discovered that the beam transected the anterior leaflet of the mitral valve and not necessarily the back wall of the left atrium” (1).
Many great contributions to the development of echocardiography as we know it came during the 1960s. Interest in this new diagnostic method greatly increased during those years and manuscripts started to be published on the application and value of its use (the first American article on cardiac ultrasound imaging dates back in 1963, by Joyner and colleagues) (7). Great personalities like the above-mentioned Harvey Feigenbaum started to give their invaluable contribution to this new field of diagnostic medicine.
Serendipity is probably the best term to describe how fortuitous and critical the way the “father of echocardiography” stumbled upon cardiac ultrasounds was (1):
“In 1963, I was working in the cardiac catheterization laboratory […] measuring output, volumes, and pressures. I was frustrated with the tediousness and inaccuracies inherent in these techniques. As is my common practice, I usually eat lunch in my office and read “throw-away literature.” In one of these throw-away journals, I noticed an advertisement by a company making ultrasound instruments […] and I arranged to see it […]. It became apparent that […] [they had] absolutely no idea how their instrument could possibly measure cardiac volume. […] Instead of turning away in disgust, [...] I proceeded to take the ultrasonic transducer and place it on my chest. I was able to find a signal that I found fascinating. I asked what would happen if there were fluid. […] [The salesman] said that the fluid should be free of any echoes. […] I then found a patient with pericardial effusion and, as predicted, there was an echo-free space […] and therein lies the origin of my career in echocardiography.”
Feigenbaum later introduced the monodimensional (M-mode) technique to measure left ventricular size in 1968 (8). The introduction of such a reliable and innovative non-invasive tool was critical in order to overcome the expected initial skepticism about echocardiography (1). A great tribute should be paid to him for his pivotal role in advancing standardization and education in this field, which literally let echocardiography survive and become an essential element in the training of cardiologists all over the world. He organized the first academic course solely dedicated to cardiac ultrasound in Indianapolis in 1968 and wrote the first book on echocardiography in 1972 (9). Feigenbaum also had the controversial intuition to separate the role of the sonographer (which could be performed by non-physicians) from the role of the interpreter (which, instead, has to be strictly performed by a physician), given sufficient standardization of the protocols of acquisition of the images. While criticism arose about the fact that in some cases medical knowledge could certainly improve the diagnostic value of the examination, it is now clear that cardiac sonographers are a precious, cost-effective resource (1,10). The next significant contribution that definitely changed the approach to the discipline was the introduction of two-dimensional (2D) echocardiography. Starting from M-mode tracings, Gramiak was able to obtain rudimental 2D images (11); real-time 2D echocardiography was later developed by Bom in the 1970s in Rotterdam (12).
As brief as it could be, no history of echocardiography would be complete without mentioning how troubled the early times of Doppler flow evaluation were.
The first description of its physical principles is attributed to Austrian mathematician Johann Christian Doppler. His initial description referred to changes in the wavelength of light as applied to astronomical events and was presented in 1842 in a paper entitled “On the Coloured Light of Double Stars and Some Other Heavenly Bodies” where he postulated that certain properties of light emitted from stars depend upon and vary strongly with the relative motion of the observer and the source of the wave.
Translating and adding this new insight into a straight-forward and reliable tool for the echocardiographic standard evaluation was not easy. As elegantly reviewed by Veyrat (13), the first one to introduce Doppler to cardiology was Satomura in 1956 (14). A huge credit should be given to the pioneering studies of Kalmanson, who introduced cardiac directional flow Doppler in 1969 (15,16). The possibility to use 2D images dramatically increased the applications of cardiac Doppler, although strong skepticism had to be overcome (in 1980 Kalmanson gave a presentation on cardiac Doppler at the French Society of Cardiology in front of a nearly empty room!) (13). Doppler examination was not considered easily accessible until Color Doppler, a much more user-friendly technique, was introduced by Bommer (17) and Namekawa (18). This new technology was able to provide information about direction, velocity, and turbulence of the blood flow (Figure 1A,B,C).
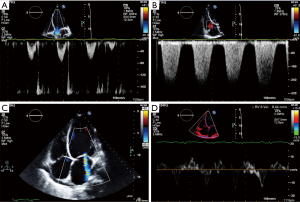
Doppler technology has undergone an impressive transformation over the last decades.
As observed by Otto, Doppler flow velocity measurements are based on backscatter of low amplitude, high velocity signals from moving blood cells. Conversely, Doppler tissue velocities are characterized by high amplitude, low velocity signals reflected from the myocardium (19). The separation of the two signals (blood vs. myocardium) by means of the application of filters able to reject echo signals originating from the blood is the basis for Tissue Doppler Imaging (TDI), a powerful tool introduced in the 1990s (20) by which systolic and diastolic function and even ventricular dyssynchrony can be assessed. Color-coded velocities superimposed on a 2D grey scale image let the user easily visualize myocardial motion in real time (21) (Figure 1D). Strain (a measure of the deformation defined as the difference between the final length and the original length divided by the original length) can also be estimated by TDI, further empowering its clinical uses.
In recent years, growing interest has characterized speckle-tracking strain imaging. This new non-Doppler technique is based on tracking the motion of natural acoustic markers (speckles, i.e., small bright spots in the myocardium) on the grey scale image as they move during the cardiac cycle (19). Speckle-tracking allows strain measurements to be taken independent of the angle, unlike the angle-dependent strain measurements using TDI. Assessment of regional wall motion abnormalities, as well as dyssynchrony, and atrial function are amongst the clinical applications for which it is most widely used.
Getting closer to, onto and into
Echocardiography is one of the most emblematic examples of the close relationship between progress in the medical field and advances of technology. The evolution of echocardiographic techniques has led to tremendous increase in the amount of information we can acquire to better define both cardiac anatomy and function. As with living beings, echocardiography follows a constant process of evolution, in that continuous changes characterize the development of diagnostic ultrasound to various situations. The need to obtain more detailed images by positioning the probe closer to, onto, or even into the heart led to the development of more invasive forms of examination: transesophageal echocardiography (TEE), epicardial echocardiography and, intracardiac echocardiography (ICE), respectively.
After the description of the first experimental transesophageal probes in the 1970s (22), TEE became popular only after European investigators proved it was safe and clinically useful (23). The first attempts to perform TEE date back to 1980, when a 2D transducer was positioned on a fiber-optic endoscope (24). Again, it is interesting to notice how the widespread use of TEE could have never been possible without the technological development of smaller, more flexible, higher frequency probes allowing better resolution and fewer artifact interferences.
The ability to perform TEE was the real breakthrough that brought echocardiography to a whole new level, i.e., “intraoperative echocardiography”. As observed by Gowda, the possibility to evaluate cardiac performance led to the early acceptance of TEE to monitor changes in ventricular function and hemodynamic measures during cardiac surgery (25). TEE is an essential tool both before and after cardiopulmonary bypass; not only does it allow confirmation of known structural and functional abnormalities that led the patient to the surgical table, it also permits additional evaluation which may change the surgical plan in the operative room (21). Its importance is crucial in patients undergoing valve surgery, excision of intra-cardiac tumors, myomectomy, as well as intra-cardiac shunt repairs and in patients with congenital heart defects.
The differing arenas of the operative room, cath lab, and electrophysiology (EP) lab allow even closer examination of structures needing interrogation.
The epicardial approach is unique in that it requires a collaborative effort with the cardiac surgeon to allow the echocardiographer to have direct access to the epicardial surface within the operative field (26). Epicardial echocardiography was first introduced in 1972; on that occasion, M-mode was used to evaluate the results following open mitral commissurotomy (27). The use of high frequency probes together with sterile sheaths makes epicardial echocardiography an extraordinary tool when TEE quality is suboptimal. Among all intraoperative imaging modalities, epicardial echocardiography can be the most practical one when TEE probe cannot be inserted or when probe placement is contraindicated, assisting cardiac surgeons, anesthesiologists, and cardiologists with clinical decision-making. In addition, it may offer better windows for imaging anterior cardiac structures (aorta, aortic valve, pulmonic valve, pulmonary arteries).
As observed by Bartel et al. (28), ICE not only complements, it has in part replaced TEE. Nevertheless, unlike TEE, ICE is a “purely intraprocedural guiding and imaging tool unsuitable for diagnostic purposes” which typically uses a catheter-like ultrasound probe inserted through a venous sheath as part of a cath or an EP lab procedure. High frequency probes are required along with great expertise for intracardiac manipulation of catheters (19). It is usually well-tolerated and does not require general anesthesia; moreover, in recent years, also 3D-ICE evaluation has become available, albeit not yet widely used. Although it is a potentially risky procedure, its combination with an already invasive procedure confers little additional risk. Its indications currently range from device closure of interatrial communications to EP procedures, as well as (but not limited to) trans-catheter aortic valve implantation (TAVI). Its potential use in assisting MitraClip implantation, left atrial appendage closure and paravalvular leaks closure is still matter of investigations; further studies comparing ICE vs. TEE and fluoroscopy are needed before it can be adopted as a primary imaging modality for those procedures (28).
The never-ending quest for better imaging
The need to acquire better, more detailed images not only led to the development of new technologies allowing to get closer to (TEE), onto (epicardial echocardiography) and, into (ICE) cardiac chambers. Following M-mode (Figure 2A) and 2D-mode (Figure 2B), three-dimensional (3D)-echocardiography (Figure 2C) was later introduced. Nevertheless, to consider this as a sort of “evolutionary scale” in which the most recent modality fully replaces the previous one, would be highly misleading. All the above-mentioned modalities are currently used to make the echocardiographic evaluation as comprehensive as possible, by obtaining different and complementary information from each.
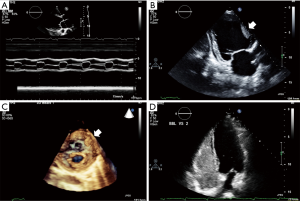
The term 3D-echocardiography, in particular, is very broad. It refers to “several approaches aiming at the acquisition and display of cardiac ultrasound images” (19). Three-dimensional echocardiography has a long history with the first 3D-reconstruction of 2D images in 1974 (29) by means of equipment that was far from being considered practical or of any real clinical use. Following the efforts of biomedical engineering, smaller equipment was developed together with complex transducers, able to generate high quality images. Initially, acquisition of a series of 2D images to be subsequently consolidated into a unique 3D image was needed. The need to “usher in the third dimension” (30) has led to strong improvements in both hardware and software, allowing 3D echocardiography to mitigate many of the limitations faced by the 2D technique for the assessment of left ventricular volume and function. The entire left ventricle can be sampled by means of pyramidal datasets which can be acquired over a single beat (real-time) or more beats (near real-time), using the ECG signal as the trigger. These slices are then “stitched” together to generate a 3D image of the entire structure (30). Moreover, the extreme accuracy and feasibility of 3D echocardiography in studying valvular and congenital lesions has been largely demonstrated in the operating room, where 3D TEE has been proved to be feasible, able to accurately predict valve morphology, and to provide additional and complementary morphologic information compared with conventional 2D TEE (31).
A certain degree of serendipity also characterizes the introduction of contrast echocardiography in 1969 at the University of Rochester (1). While Gramiak and Shah were performing an ultrasound examination on a patient undergoing cardiac catheterization to evaluate cardiac output, they noticed that the injection of indocyanine green dye produced a cloud of echoes within the heart (32) (a phenomenon very similar to that observed earlier with saline by Joyner, who never reported it). Gramiak and Shah later gave a presentation describing this new approach that fascinated Feigenbaum, who started investigating possible new clinical implications of contrast echocardiography. This discovery enabled examination of the characteristic of flow patterns in the presence of normal and abnormal cardiac hemodynamics (33). The potentially huge benefits of contrast echocardiography in detecting intra-cardiac shunts were subsequently examined in depth by researchers from the Mayo Clinic (34). Today contrast echocardiography is a useful tool to overcome some of the limitations of standard TTE, as in the case of obese patients or patients with lung disease, for whom image quality could be suboptimal (Figure 2D).
Getting smaller, getting smarter
Smaller echo machines have been marketed in the last years, from a “laptop” format size to the most recent “pocket-size” hand-held format. These brand-new devices portend the potential to dramatically increase portability and expand diagnostic ability of the physician, although it has to be noticed that point-of-care assessment is not meant to substitute a comprehensive echocardiographic exam. A summary of the indications for pocket-size devices has been provided by the European Association of Echocardiography (35) which recommends that they should be regarded as a “complement” to a physical examination (particularly in the setting of coronary and intensive care units) or as a tool for a fast, initial screening in an emergency setting (including first cardiac evaluation in ambulances); moreover, their use could be extended to screening programs, triaging candidates for a complete echocardiographic examination, and as teaching tool in medical schools. If correctly used, pocket-size devices portend the potential not only to increase diagnostic accuracy, but also to save health-care resources, reduce waiting lists for inpatients and improve teaching. In any case, “with the exception of cardiologists who are certified for TTE according to national legislation, specific training and certification is recommended for all users”, subject to the fact that this certification “should be limited to the clinical questions that can potentially be answered by pocket-size devices” (35).
Briefly, only focused evaluations can be currently performed, even when used by expert sonographers; nevertheless, the potential benefits that this new technology could offer in the next future are multiple (36).
Conclusions
Echocardiography is an amazingly versatile technology, able to provide critical information both non-invasively and invasively. If properly indicated and correctly performed, its cost-effectiveness can be tremendously high, avoiding the need for more expensive and potentially riskier diagnostic tests.
Since its first days as a simple tool to assess pericardial effusion and mitral valve abnormalities, echocardiography has come a long way, achieving incredible advancements, widespread use, and an increasing level of complexity, which makes education and training “imperative to provide high-quality examinations and proper interpretation” (1). Credit should be given to the pioneers that believed in this innovative technology despite initial skepticism.
The promise of further developments, the continuing efforts of both physicians and non-physicians, together with the hope for possible future “happy accidents”, will keep this powerful diagnostic tool a major asset in the field of diagnostic medicine.
Acknowledgements
None.
Footnote
Conflicts of Interest: The authors have no conflicts of interest to declare.
References
- Feigenbaum H. Evolution of echocardiography. Circulation 1996;93:1321-7. [Crossref] [PubMed]
- Curie P, Curie J. Developpement, par pression de l’electricite polaire dans les cristaux hemiedres a faces inclinees. Comptes Rendus 1880;31:291-5.
- Curie P, Curie J. Lois du degagement de l’electricite par pression, dans la tourmaline. Comptes Rendus 1881;92:186.
- Wild JJ, Crawford HD, Reid JM. Visualization of the excised human heart by means of reflected ultrasound of echography;preliminary report. Am Heart J 1957;54:903-6. [Crossref] [PubMed]
- Edler I, Hertz CH. The use of ultrasonic reflectoscope for the continuous recording of the movements of heart walls. 1954. Clin Physiol Funct Imaging 2004;24:118-36. [Crossref] [PubMed]
- Mayer P. At the Heart of the Matter An Overview of Adult Echocardiography for the Non-Cardiac Sonographer. J Diagn Med Sonogr 2015;31:221-32. [Crossref]
- Joyner CR, Reid JM, Bond JP. Reflected ultrasound in the assessment of mitral valve disease. Circulation 1963;27:503-11. [Crossref] [PubMed]
- Feigenbaum H, Popp RL, Wolfe SB, et al. Ultrasound measurements of the left ventricle. A correlative study with angiocardiography. Arch Intern Med 1972;129:461-7. [Crossref] [PubMed]
- Feigenbaum H. Echocardiography. Philadelphia: Lea & Febiger, 1972.
- Nicastro I, Barletta V, Conte L, et al. Professional education, training and role of the cardiac sonographer in different countries [Internet]. [cited 2016 Nov 30]. Available online: http://www.jcecho.org/article.asp?issn=2211-4122;year=2013;volume=23;issue=1;spage=18;epage=23;aulast=Nicastro
- Gramiak R, Waag RC, Simon W. Ciné ultrasound cardiography. Radiology 1973;107:175-80. [Crossref] [PubMed]
- Bom N, Lancée CT, Honkoop J, et al. Ultrasonic viewer for cross-sectional analyses of moving cardiac structures. Biomed Eng 1971;6:500-3, 5.
- Veyrat C. The glorious years leading Doppler flow to the heart, the odyssey of the pioneers! Acta Cardiol 2014;69:351-65. [PubMed]
- Satomura S. Study on examining the heart with the ultrasonic Doppler method. Jpn Circ J 1956;20:227-8.
- Kalmanson D, Novikoff N, Chiche P, et al. Study and physiologic interpretation of right intracardiac blood velocity curves by directional untrasonic probes with Doppler effect. Comptes Rendus 1969;269:1097-100. [PubMed]
- Kalmanson D, Toutain G, Novikoff N, et al. Velocimetric catheterization of the heart and large vessels by directional ultrasonic probe with Doppler effect. Preliminary report. Ann Med Interne (Paris) 1969;120:685-700. [PubMed]
- Bommer W, Miller L. Real-time two-dimensional color-flow Doppler:enhanced Doppler flow imaging in the diagnosis of cardiovascular disease. Am J Cardiol 1982;46:944. [Crossref]
- Namekawa K, Kasai C, Koyano A. Imaging of blood flow using autocorrelation. Ultrasound Med Biol 1982;8:138.
- Otto C. Textbook of Clinical Echocardiography. 5th ed. Philadelphia: Elsevier, 2013.
- McDicken WN, Sutherland GR, Moran CM, et al. Colour Doppler velocity imaging of the myocardium. Ultrasound Med Biol 1992;18:651-4. [Crossref] [PubMed]
- Maleki M, Esmaeilzadeh M. The evolutionary development of echocardiography. Iran J Med Sci 2012;37:222-32. [PubMed]
- Frazin L, Talano JV, Stephanides L, et al. Esophageal echocardiography. Circulation 1976;54:102-8. [Crossref] [PubMed]
- Schluter M, Henrath P. Transesophageal echocardiography:potential advantages and initial clinical results. Pract Cardiol 1983;9:149.
- Hisanaga K, Hisanaga A, Nagata K, et al. Transesophageal cross-sectional echocardiography. Am Heart J 1980;100:605-9. [Crossref] [PubMed]
- Gowda RM, Khan IA, Vasavada BC, et al. History of the evolution of echocardiography. Int J Cardiol 2004;97:1-6. [Crossref] [PubMed]
- Reeves ST, Glas KE, Eltzschig H, et al. Guidelines for performing a comprehensive epicardial echocardiography examination:recommendations of the American Society of Echocardiography and the Society of Cardiovascular Anesthesiologists. J Am Soc Echocardiogr 2007;20:427-37. [Crossref] [PubMed]
- Johnson ML, Holmes JH, Spangler RD, et al. Usefulness of echocardiography in patients undergoing mitral valve surgery. J Thorac Cardiovasc Surg 1972;64:922-34. [PubMed]
- Bartel T, Müller S, Biviano A, et al. Why is intracardiac echocardiography helpful? Benefits, costs, and how to learn. Eur Heart J 2014;35:69-76. [Crossref] [PubMed]
- Dekker DL, Piziali RL, Dong E. A system for ultrasonically imaging the human heart in three dimensions. Comput Biomed Res 1974;7:544-53. [Crossref] [PubMed]
- Skubas NJ, Mahajan A. Intraoperative evaluation of left ventricular volume and function:it is time to usher in the third dimension. Anesth Analg 2014;118:698-700. [Crossref] [PubMed]
- Abraham TP, Warner JG, Kon ND, et al. Feasibility, accuracy, and incremental value of intraoperative three-dimensional transesophageal echocardiography in valve surgery. Am J Cardiol 1997;80:1577-82. [Crossref] [PubMed]
- Gramiak R, Shah PM, Kramer DH. Ultrasound cardiography:contrast studies in anatomy and function. Radiology 1969;92:939-48. [Crossref] [PubMed]
- Feigenbaum H, Stone JM, Lee DA, et al. Identification of ultrasound echoes from the left ventricle by use of intracardiac injections of indocyanine green. Circulation 1970;41:615-21. [Crossref] [PubMed]
- Seward JB, Tajik AJ, Spangler JG, et al. Echocardiographic contrast studies:initial experience. Mayo Clin Proc 1975;50:163-92. [PubMed]
- Sicari R, Galderisi M, Voigt JU, et al. The use of pocket-size imaging devices:a position statement of the European Association of Echocardiography. Eur J Echocardiogr 2011;12:85-7. [Crossref] [PubMed]
- Johri AM. Generation iUltrasound:Bedside diagnostic imaging with Hand-Held Cardiac Ultrasound. Cardiol J 2014;21:98-9. [Crossref] [PubMed]