Role of circulating factors in cardiac aging
Introduction
Aging is a pivotal contributor to several diseases and a major risk factor for numerous chronic conditions affecting the whole body (1). The aging process is responsible itself for several structural and functional changes and for the increased incidence of several cardiovascular risk factors such as hypertension, dyslipidemia and diabetes (2,3). The concepts that circulating factors may regulate the aging process have shed the light on this issue contributing to the development of novel therapeutic strategies to promote healthy aging (4-6).
The aim of this review is to retrace the potential role of circulating and soluble factors to halt, reverse or even ameliorate the pathophysiological process of cardiac aging.
Aging, the magnitude of a problem
Aging may be considered a modern pandemic, associated with a serious social and economic impact. By 2050 more than two over nine billions of estimated people will be older than 60 years and yet in 2017 there will be more people over 65 than under 5 years old (7). In the European Union (EU), by 2060, approximately one third of the population will be aged 65 or over (Commission, 2012 #3816) whereas in the United States, 1 every 7 Americans is already older than 65 (8).
The progressive increase in life expectancy is associated with higher prevalence of chronic age-related disease (9). In this light, understanding the mechanisms underlying this process and the changes that physiologically occur with time, is pivotal to improve the quality of life of the elderly and reduce the burden of age-related diseases (10).
Aging is considered an invariable and progressive time-dependent decline in organism functions due to the constant gait of time (11) and is associated with deleterious changes leading to functional impairments typical of the elderly (12).
Aging is itself a major risk factor for several diseases, mostly chronic. Cardiovascular disease, cancer, degenerative disorders, immune-mediated diseases show indeed a higher prevalence in the elderly population, mainly because of the loss of the adaptive response to insults and an imbalance in tissue homeostasis (13). The aging process undeniably affects the cardiovascular system and the prevalence of cardiovascular diseases increases overtime. As early as in the 1970s, McKee and coll. identified in the Framingham study that older age was an independent risk factor for heart failure (HF) and aging was a predictor of worse prognosis in those patients (14). Aging indeed contributes significantly to the global burden of cardiovascular risk factors, HF, cardiomyopathies and ischemic heart disease (2). In particular, HF represents a major clinical and socio-economical problem characterized by significant morbidity and mortality and progressive increase in healthcare expenses, especially in those older than 65 years (15,16).
The aging process induces structural and functional changes such as vascular stiffening, myocyte hypertrophy and increased wall thickness, increased myocardial fibrosis and extracellular matrix (ECM) remodeling leading together to diastolic dysfunction characterized by reduced active filling of the left ventricle. These changes may explain the higher prevalence of heart failure with preserved ejection fraction (HFpEF) in the aging population (17-19). While aging may not represent the direct cause of HF, this process is associated with a lower threshold for clinical signs and symptoms of the disease (15,20).
Patients with HFpEF present normal ventricular volumes and ejection fraction, as opposed to heart failure with reduced ejection fraction (HFrEF), and a severe impairment in ventricular relaxation (21,22). Furthermore, those patients are often female and usually older with numerous comorbidities such as obesity, diabetes, and hypertension when compared to patients with HFrEF (23).
Despite several efforts, to date there are no specific treatments for this condition, therefore it appears crucial to develop novel strategies to understand the pathophysiological background and the possible therapeutic targets for patients with HFpEF (24).
Circulating factors in cardiac aging
A number of recent evidences indicate a pivotal role for circulating factors associated with aging in affecting the function of the cardiovascular system (Figure 1). While until recently the concept that circulating factors may affect cardiovascular health was limited mainly to lipids (25) and systemic factors mainly represented useful biomarkers for the diagnosis and for risk stratification of individuals at higher risk of cardiovascular disease (26-29), their role in promoting aging and pathological process has been recently highlighted (30-33).
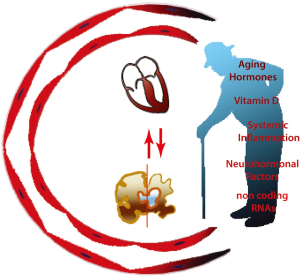
Blood, circulating factors and in vivo parabiosis
The concept that blood carries either beneficial or detrimental factors is not a novel finding. Hippocrates from Kos identified the four “evil humors” responsible for several disease and proposed the bloodletting, known as phlebotomy, as effective treatment for those conditions (34,35). In most recent times, bloodletting was considered still an available option for untreatable disease (36) and Sir William Osler recommended the phlebotomy as a therapeutic choice in his textbook “The Principles and Practice of Medicine” (37,38).
Blood carries circulating factors, like hormones, which can affect organs distant from their site of production. The diversified world of the hormones has not yet been completely characterized and a number of circulating factors with this role have yet to be identified (39). While circulating factors are important biomarkers in different conditions, growing evidences indicate their role as a therapeutic option for cardiovascular disease (40,41), liver disease (40), cancer (42), and neurological disease (43).
To study the possibility that a shared circulatory system may affect a specific condition, Paul Bert, in the mid-1800s, introduced the surgical technique of parabiosis in which, he hypothesized, surgically connected animals may develop a shared circulation (44). Animals joined in parabiosis develop a shared blood circulation, with rapid and continuous exchange of cells and soluble factors at physiological levels through their common circulatory system (45). This surgical technique has been underemployed until the 1970s when, Coleman and Coll., conjoined obese mice and diabetic mice in parabiosis with WT mice, paving the way to discover the role of leptin and leptin receptor in diabetes (46,47). More recently, in a revival of this interesting old-fashioned technique, heterochronic parabiosis, a specific experimental procedure whereby two animals of different ages are joined together, allowed to study the role of shared circulation in several conditions, such as muscular atrophy, neurodegenerative disorders, cardiovascular diseases, and to discover several soluble factors that can affect specific phenotypes in experimental animals (48-52).
Growth differentiation factor 11 (GDF11) was identified as the factor that recapitulates the effect of heterochronic parabiosis on cardiac muscle, reversing age-related cardiac hypertrophy (50). Further studies have extended the effect of GDF11 to restoration of skeletal muscle function and improved angiogenesis in the brains of aging mice (49,53).
Other studies have challenged these results, in part because of the homology with myostatin (GDF8), that render difficult to discriminate the effect of the specific proteins (54,55). Some of these discrepancies may be explained by the complexity and redundancy of the pathways and by the role of specific post-translational forms of GDF11, including specific antagonists (56,57). GDF11 has shown a dose-dependent effect on cardiac mass (58) and, although further studies are needed to clarify its specific role, the possibility of targeting specifically cardiomyocytes and other cells with aging hormones may represent a novel therapeutic option.
Circulating factors in aging: the intersection between brain and heart
Heart and brain have an intimate relationship, more evident in aging when the link between heart dysfunction and the brain activity become manifest (59).
Cardiovascular pathologies, cerebrovascular disorders and neurodegenerative diseases are prominent features of aging and dementia and cognitive impairment represent the main cause of disability in older people. The most common forms of dementia, Alzheimer’s disease (AD) and vascular dementia (VaD), cover about 80-90% of all dementias (59) and these pathologies are usually associated with risk factors for cardiovascular disorders and heart dysfunction.
Traditionally VaD, a pathological condition due to embolic stroke, cardiac dysfunction or age-related vascular stiffening responsible for chronic hypoperfusion, was formerly considered the entity promoted by diabetes mellitus, hypertension, hypercholesterolemia, obesity or closely related to cardiac pathologies. Recently, a large body of evidence shows that cardiovascular risk factors are also associated with AD, considered a purely neurodegenerative disorder (60).
In normal brain aging and in AD pathology, two neuropathological hallmarks characterize the brain tissue: extracellular deposits of amyloid beta (Aβ) protein in which misfoled Aβ fibrils are organized in senile plaques and intraneuronal aggregates of hyperphosphorylated and misfolded tau protein that become extraneuronal (“ghost” tangles) when tangle-bearing neurons die (61). Aβ protein spontaneously self-aggregates into other multiple physical forms than fibrils and one of them consists of oligomers. Most evidences support the notion that the early stages of Aβ oligomerization rather than the fibrils in senile plaques are responsible for the toxic effects of Aβ at synaptic level (62). During aging and in the progression of AD, synaptic plasticity, capacity of sprouting and neuronal integrity is compromised. Recent studies suggest that the direct abnormal accumulation of Aβ oligomers in the neuronal terminals might contribute to the synaptic damage and plasticity deficit, leading to cognitive impairment although the biological metastable nature of Aβ oligomers makes difficult their synaptic detection (62).
In addition to Aβ and Tau effects, multiple and complex factors have been identified in AD pathogenesis including oxidative stress, mitochondrial damage, inflammatory responses and changes in cellular communication. This complexity is the main reason by which there is still a lack of understanding of initiating disease mechanisms and the absence of effective treatment options (63). A large body of evidence shows that caloric restriction, exercise, mental activity and the control of cardiovascular risk factors can counteract the aging brain (52).
Recent findings have highlighted the importance of circulating factors in determining and reversing the course of brain aging and the profound intersection between heart and brain (49,51,52). The exposure of old animals to young blood in the later phase of their life is able to rejuvenate synaptic plasticity and to improve cognitive function therefore counterbalancing the aging process (52). Furthermore, in Xenopus oocytes, macrophage-derived soluble factors may directly activate N-methyl-d-aspartate (NMDA) receptor subtype NR1a/NR2B delaying the onset of AD (43). The exposure to young blood enhanced the complex interplay between vascular system and cognitive function. Indeed, circulating factors in young animals improved vascular function in the aging mouse brain, leading to increased blood flow and ultimately to increased neural activity and functioning (49).
Taken together these notions suggest that blood-borne factors are essential to ameliorate the brain aging phenotype (51,64).
Although these experiments have been performed in rodents, these results have sufficiently convinced the researchers to initiate a human study, testing the transfusion of young human plasma in patients with different types of dementia. The study (Plasma Study), started in September 2014 with final data collection in November 2016, enrolled 18 demented subjects and the preliminary data are not yet known (65) (http://clinicaltrials.gov/ct2/show/NCT02256306). More recently a pay-to-participate trial (Ambrosia’s trial) started in Monterey (CA) (66). Healthy volunteers and not necessarily elderly—the trial is open to anyone 35 and older—are enrolled in order to receive transfusion of plasma from donors under age 25 to test for more than 100 biomarkers that may vary with age. For the study characteristics, the trial is giving rise to huge ethical problems.
Systemic inflammation
Inflammation is the common pathway occurring within the vasculature and throughout the body in response to an injury (67). Systemic inflammation associated with aging, even in absence of a specific pathological process (68), has a key role in determining structural and functional changes in the aging myocardium (13), underlying the pathophysiological process of cardiac frailty and cardiovascular pathology (69). Even in the absence of chronic conditions, circulating inflammatory factors, such as interleukin (IL)-6, tumor necrosis factor (TNF)-α and soluble TNF receptor-1 (TNFR-1), and C-reactive protein (CRP) are usually two to four folds higher in the elderly compared to young subjects (70). The inflammatory response is tightly associated with increased production of reactive oxygen species (ROS), mitochondrial damage, and accelerated senesce (71). Furthermore, subtle inflammatory processes lead to arterial stiffening and endothelial dysfunction resulting in age-related inflammatory chronic disease, i.e., atherosclerosis and hypertension (69,72,73). Hosford-Donovan and Coll. demonstrated that higher level of CRP were independently associated with hypertensive phenotype in 65–70 years old women, postulating that chronic inflammation may influence blood pressure’s regulation in the elderly leading to increased vascular stiffening and accelerated atherosclerosis (74).
IL-6 levels are a marker of cardiovascular disease and serum concentration of this cytokine increases with age (13,75). Elevate levels of IL-6 are found in aged mice hearts and deletion of myocardial Insulin-like Growth Factor (IGF)-1 Receptor rescues the aging cardiac phenotype suggesting a possible link between these two factors (76). In humans, elevated level of IL-6 were associated with higher cardiovascular mortality over a period of 3 years of follow-up indicating that systemic inflammation may contribute to the cardiac aging process (77).
The measurement of serum concentration of CRP is a well-known useful marker for patients at higher risk of atherosclerotic disease and the prognostic value of this evaluation is comparable to blood cholesterol measurements (78,79). Furthermore, CRP concentration increases significantly with age, without gender differences, and provides a reliable measurement in assessing the risk of future cardiovascular events (80,81). Although CRP can be considered as a useful marker for cardiovascular disease, it is unlikely that specific treatments targeting this molecule may provide a net clinical benefit (82).
While several attempts to target this condition using therapeutic anti-inflammatory regimens to reduce cardiovascular mortality have failed (83), targeting the whole CRP/IL-6/IL-1 axis with monoclonal antibodies could open new therapeutic lines in cardiovascular pathology and offer novel insights for the aging process of the heart (82). An interesting finding indicates an inverse relationship between serum level of testosterone and CRP, warning regarding the increased risk of cardiovascular disease in aging men and linking the neurohormonal axis to the inflammatory process (84).
While targeting systemic inflammation may represent an interesting therapeutic option, so far little evidence are available indicating its employment in the near future (83).
Vitamin D
Vitamin D deficiency is a serious health issue worldwide, requiring special attention (85-87). Vitamin D is a fat-soluble vitamin responsible for calcium and phosphorus homeostasis (88,89). Vitamin D deficiency has been previously associated with poorer neuropsychological function, metabolic syndrome, arrhythmias and coronary artery disease, and shares a complex interplay with other cardiovascular risk factors (88-93). Numerous studies hypothesized that low levels of Vitamin D are inversely associated with cardiovascular disease and cardiac aging, however evidences and data from large clinical trials are limited (94-96). By contrast, higher levels of Vitamin D have been associated with longer leukocyte telomere length (LTL) suggesting a potential beneficial effect of this vitamin on the aging process (97). Therefore, besides the well-known effect of sun exposure on bone’s health, preservation of normal values of Vitamin D appears beneficial for cardiovascular health and healthy aging process (85,94).
Neurohormonal factors
Sympathetic nerve stimulation (SNS), Renin Angiotensin Aldosterone System (RAAS) and Natriuretic Peptides (NP), are part of the defense system adopted by the body to maintain fluid homeostasis and vascular resistance and provide proper perfusion to distant organs (98). Aging is associated to increased activation of the neurohormonal system, in part as a result of an imbalance between production and clearance of vasoactive molecules (99) and can in part explain the high incidence and prevalence of HF in the elderly (15). While the activation of these systems during the early phase of HF may normalize cardiac output and perfusion, chronic activation has a deleterious impact on the outcome of this condition, promoting structural and functional changes in the myocardium and the vasculature that eventually contribute to decompensated HF (100-103). Thus, in order to prevent the progression of HF and to improve morbidity and mortality, several treatments targeting this axis and modulate their activity have been developed (104,105). HF is also a risk factor for neurodegenerative disorders, possibly because impaired blood flow and neurohormonal activation favor accumulation of Aβ plaques and neurofibrillary tangles (106), indicating the strict link between aging and chronic disorders.
Activation of RASS, which is pivotal for fluid and blood pressure homeostasis (98), leads to increased level of Ang-II, which has a pro-hypertrophic and pro-fibrotic effect. High levels of Ang-II are tightly associated with cardiomyocyte hypertrophy, ECM remodeling and increased collagen deposition and ultimately to cardiac fibrosis (13,107,108). These changes at the tissue level contribute to clinical manifestations of cardiac aging, such as diastolic dysfunction, impaired relaxation and reduced compliance of the ventricle, and promote the progression to heart failure with ejection fraction either preserved (HFpEF) or heart failure with ejection fraction either reduced (HFrEF), both in humans and in animals (109). Recent studies in hypertensive and aging animals have shown how hyperactivation of the RAAS system reduces ventricular compliance through increased titin-based myocardial stiffness, ultimately contributing to diastolic dysfunction and development of HFpEF, underlining the pleiotropic negative effects of the persistent increased activation of RAAS (110,111). Persistent activation of RAAS also stimulates β-amiloid production (112), an important link between cardiac and neuronal aging (113). Ang-II has important pro-inflammatory effects, leading to increased secretion of TNFα, IL-1β, and IL-6 (114). With aging, increased levels of Ang-II stimulate NADPH oxidase 4 (NOX4) on the mitochondrial membrane enhancing the oxidative damage, a cornerstone of the aging process (115-117). Ang-II enhances cardiac fibroblast proliferation through NOX4/ROS-dependent IL-18 induction, MMP9 and p38 MAPK activation, promoting cardiac remodeling and diastolic dysfunction (118,119), while blockade of the RAAS delays the fibrotic response of the myocardium (120).
In humans, proteins of the RAAS system can be found in the urine of healthy aged individuals (121) suggesting an increased activity of this system, possibly explaining the progressive cardiac and kidney failure (122-124), the increased incidence of hypertension, due endothelial dysfunction and arterial stiffness (125), the high incidence of metabolic syndrome and diabetes (126), and the aging phenotype (127,128).
RAAS blockade with angiotensin converting enzyme inhibitors (ACE-I) or angiotensin receptor blockers (ARBs) is able to reduce cardiovascular mortality independently from blood pressure lowering, either in humans or in animals (129-131), ameliorates cardiac hypertrophy and myocardial fibrosis (132), endothelial dysfunction and arterial stiffness (133) and improves animal survival (134). Furthermore, treatment with ACE-I and ARBs represent a valid therapeutic option for aged people (105,135,136), improving cerebral blood flow and reducing the 1-year risk of fall in elderly people (137), even though in hypertensive patients it is associated with worse gait performance (138) and appears to have no effect on walking distance or on age-related decline of muscle strength (139).
Aging is also associated with elevated levels of endothelin-1 (ET-1), in part stimulated by Ang-II (140), promoting the development of the an aging phenotype characterized by ECM remodeling, increased collagen deposition and endothelial dysfunction contributing to a progressive impairment of the diastolic function and eventually to HFpEF (141,142). ET-1 stimulates collagen deposition through both endothelin receptor type A (ETA) and type B (ETB), and administration of ETA receptor antagonists is able to reduce either cardiac or renal fibrosis (141,143,144). Furthermore, antidepressant treatment with venlafaxine, commonly used in elderly population, is able to modulate TGF-β expression and to reduce brain damage in a rat model of ET-1 induced stroke, confirming the key role of ET-1 in the progression of vascular damage (145).
NP are a family of different peptides acting directly on cardiovascular and renal systems, balancing fluid homeostasis (146) and promoting vasorelaxation, natriuresis and diuresis (147). Plasma levels of NPs are crucial in the evaluation of patients with HF (146,148). Elevated serum levels of brain natriuretic peptide (BNP) are independently associated with poorer outcomes (149,150) and predict higher in-hospital mortality in very elderly patients admitted for HF (151). BNP levels are elevated in the aging population compared to the younger counterparts even in the absence of a clear diagnosis of HF (152,153). BNP levels are persistently elevated in the elderly independently from blood pressure changes, renal function, atrial volumes, myocardial mass or other age-related changes (154,155) suggesting the importance of these circulating factors in normal aging. NPs increase the production of cGMP (156) and are degraded mainly through the enzyme Neprilysin (NEP), a zinc-dependent enzyme widely expressed throughout the body (157).
In animals, increased levels of NPs or chronic inhibition of NEP are able to prevent the progression of cardiac aging (158,159). Similarly, in humans, simultaneous inhibition of NEP and Ang-II type-1 receptor (AT1R) with LCZ-696 (which is composed by Valsartan and Sacubitril) has shown to reduce significantly mortality and hospitalization in patients with HF (160). It is estimated that this compound, recommended in the most recent guidelines on treatment of HF (135,136), may add 1 to 2 years to life expectancy in these patients (161).
Organism homeostasis and the correct balancing of circulation factors are essential for physiological and healthy aging. Imbalance of these axis significantly affects the aging phenotype, thus therapeutic strategies acting on these targets may ameliorate lifespan and healthspan of the aging population.
MicroRNAs (miRNAs) and long non coding RNAs (lncRNA)
Circulating miRNAs are single-stranded and non-coding RNA molecules of approximately 22 nucleotides regulating several biological activities (162). miRNAs emerged as important biomarkers and therapeutic target for several conditions, including HF (163). Numerous miRNAs, such as miR-146, miR-155, miR-21, miR-126 appear to be involved in the aging process (164,165), in cardiac remodeling observed with aging (166,167) and are associated with prognosis and response to therapy in HF (163).
The miR-34 family, which includes miR-34a, miR-34b and miR-34c and is important in cancer formation, metastasis, and cell viability (168), is tightly associated with the aging process (169). Indeed, elevated levels of miR-34 are found in the heart of old mice (170) and the inhibition of its downstream target, the protein phosphatase-1 regulatory subunit-10 (PNUTS) is associated with increased cardiomyocyte apoptosis, telomere attrition and cardiac contractile impairment, typical hallmarks of cardiac aging (171).
Like miRNA, lncRNA, which are the vast majority of non-coding RNAs and are longer than 200 nucleotides, are also important modulator of the aging process (172,173). lncRNAs are key regulator of the aging process and play and important role in the onset and progression of cardiac aging (174,175). Senescence-associated lncRNAs (SAL-RNAs) may indeed influence the aging phenotype, regulating cardiac function and myocardial fibrosis (176). In particular, SAL-RNA1 appears to delay cardiac aging (176) while SAL-RNA2 and SAL-RNA3 promote the survival of the senescent fibroblasts through the increased expression of p53 (174). Furthermore, the lncRNA H19 is an important modulator of the aging process, negatively affecting cell proliferation and promoting cellular senescence (177,178).
miRNAs and lncRNAs, interesting and useful biomarkers, represent also a potential therapeutic target to modulate the aging process. Recently, Kaneko and Coll. demonstrated that treatment with ARBs reduces the serum level of miR-146a, miR-149, miR-150, and miR-342-3p, improving survival rate and ameliorating congestion of the animals treated, suggesting possible crossroads between these two pathways of cardiac aging (179). Further studies are needed to elucidate the mechanism underlying this process and to develop tailored therapy to prevent the progression of the aging phenotype.
Conclusions
The aging epidemic that we are observing will benefit from our understanding of the molecular mechanisms that regulate cardiovascular aging with clear repercussions on other interconnected systems. The recent notions that circulating factors may contribute to control aging and the chronic illnesses that are strictly connected to this phenotype can be seen as an intriguing finding but also as a new therapeutic opportunity. A number of therapeutic options that interferes with circulating mediators of cardiac aging have shown a clear role in ameliorating the burden of chronic cardiovascular conditions. Unraveling the complex systemic mechanisms that regulate cardiac aging will provide novel pharmacologic strategies with a clear impact on quality of life in a progressively aging world.
Acknowledgements
We would like to thank Fondazione Generali, Fondazione Casali, Fondazione Cassa di Risparmio di Trieste and Fondazione Cassa di Risparmio Gorizia (supports assegno di ricerca for FSL) for the scientific support and research contracts.
Funding: This work was supported by the ICGEB General Fund.
Footnote
Conflicts of Interest: The authors have no conflicts of interest to declare.
References
- Larson MG. Assessment of cardiovascular risk factors in the elderly: the Framingham Heart Study. Stat Med 1995;14:1745-56. [Crossref] [PubMed]
- Mozaffarian D, Benjamin EJ, Go AS, et al. Heart Disease and Stroke Statistics-2016 Update: A Report From the American Heart Association. Circulation 2016;133:e38-e360. [Crossref] [PubMed]
- Hertel J, Friedrich N, Wittfeld K, et al. Measuring Biological Age via Metabonomics: The Metabolic Age Score. J Proteome Res 2016;15:400-10. [Crossref] [PubMed]
- Conboy IM, Conboy MJ, Rebo J. Systemic Problems: A perspective on stem cell aging and rejuvenation. Aging (Albany NY) 2015;7:754-65. [Crossref] [PubMed]
- Wyss-Coray T. Ageing, neurodegeneration and brain rejuvenation. Nature 2016;539:180-6. [Crossref] [PubMed]
- Cannata A, Camparini L, Sinagra G, et al. Pathways for salvage and protection of the heart under stress: novel routes for cardiac rejuvenation. Cardiovasc Res 2016;111:142-53. [Crossref] [PubMed]
- International UaH. Ageing in the Twenty-First Century A Celebration and A Challenge: UNFPA and HelpAge International; 2012. Available online: http://www.unfpa.org/publications/ageing-twenty-first-century
- (AoA) USDoHaHS-AoA. Aging Statistics 2016. Available online: https://aoa.acl.gov/Aging_Statistics/
- De Luca d'Alessandro E, Bonacci S, Giraldi G. Aging populations: the health and quality of life of the elderly. Clin Ter 2011;162:e13-8. [PubMed]
- Townsend N, Nichols M, Scarborough P, et al. Cardiovascular disease in Europe — epidemiological update 2015. 2015:2696-705.
- Ho AD, Wagner W, Mahlknecht U. Stem cells and ageing. The potential of stem cells to overcome age-related deteriorations of the body in regenerative medicine. EMBO Rep 200;6 Spec No:S35-8.
- Rae MJ, Butler RN, Campisi J, et al. The demographic and biomedical case for late-life interventions in aging. Sci Transl Med 2010;2:40cm21. [Crossref] [PubMed]
- North BJ, Sinclair DA. The intersection between aging and cardiovascular disease. Circ Res 2012;110:1097-108. [Crossref] [PubMed]
- McKee PA, Castelli WP, McNamara PM, et al. The natural history of congestive heart failure: the Framingham study. N Engl J Med 1971;285:1441-6. [Crossref] [PubMed]
- Roger VL. Epidemiology of heart failure. Circ Res 2013;113:646-59. [Crossref] [PubMed]
- Mahmood SS, Wang TJ. The epidemiology of congestive heart failure: the Framingham Heart Study perspective. Glob Heart 2013;8:77-82. [Crossref] [PubMed]
- Chen CH, Nakayama M, Nevo E, et al. Coupled systolic-ventricular and vascular stiffening with age: implications for pressure regulation and cardiac reserve in the elderly. J Am Coll Cardiol 199832:1221-7. [PubMed]
- Lakatta EG, Levy D. Arterial and cardiac aging: major shareholders in cardiovascular disease enterprises: Part II: the aging heart in health: links to heart disease. Circulation 2003;107:346-54. [Crossref] [PubMed]
- Redfield MM, Jacobsen SJ, Borlaug BA, et al. Age- and gender-related ventricular-vascular stiffening: a community-based study. Circulation 2005;112:2254-62. [Crossref] [PubMed]
- Strait JB, Lakatta EG. Aging-associated cardiovascular changes and their relationship to heart failure. Heart Fail Clin 2012;8:143-64. [Crossref] [PubMed]
- Desai A, Fang JC. Heart failure with preserved ejection fraction: hypertension, diabetes, obesity/sleep apnea, and hypertrophic and infiltrative cardiomyopathy. Heart Fail Clin 2008;4:87-97. [Crossref] [PubMed]
- Loffredo FS, Nikolova AP, Pancoast JR, et al. Heart failure with preserved ejection fraction: molecular pathways of the aging myocardium. Circ Res 2014;115:97-107. [Crossref] [PubMed]
- Owan TE, Redfield MM. Epidemiology of diastolic heart failure. Prog Cardiovasc Dis 2005;47:320-32. [Crossref] [PubMed]
- Sharma K, Kass DA. Heart failure with preserved ejection fraction: mechanisms, clinical features, and therapies. Circ Res 2014;115:79-96. [Crossref] [PubMed]
- Stone NJ, Robinson JG, Lichtenstein AH, et al. 2013 ACC/AHA guideline on the treatment of blood cholesterol to reduce atherosclerotic cardiovascular risk in adults: a report of the American College of Cardiology/American Heart Association Task Force on Practice Guidelines. Circulation 2014;129:S1-45. [Crossref] [PubMed]
- Vasan RS. Biomarkers of cardiovascular disease: molecular basis and practical considerations. Circulation 2006;113:2335-62. [Crossref] [PubMed]
- O'Neill S, Bohl M, Gregersen S, et al. Blood-Based Biomarkers for Metabolic Syndrome. Trends Endocrinol Metab 2016;27:363-74. [Crossref] [PubMed]
- van der Hoeven NW, Hollander MR, Yildirim C, et al. The emerging role of galectins in cardiovascular disease. Vascul Pharmacol 2016;81:31-41. [Crossref] [PubMed]
- Ahlin F, Arfvidsson J, Vargas KG, et al. MicroRNAs as circulating biomarkers in acute coronary syndromes: A review. Vascul Pharmacol 2016;81:15-21. [Crossref] [PubMed]
- Gregersen I, Holm S, Dahl TB, et al. A focus on inflammation as a major risk factor for atherosclerotic cardiovascular diseases. Expert Rev Cardiovasc Ther 2016;14:391-403. [Crossref] [PubMed]
- Willerson JT, Ridker PM. Inflammation as a cardiovascular risk factor. Circulation 2004;109:II2-10. [Crossref] [PubMed]
- Krintus M, Kozinski M, Kubica J, et al. Critical appraisal of inflammatory markers in cardiovascular risk stratification. Crit Rev Clin Lab Sci 2014;51:263-79. [Crossref] [PubMed]
- Fox CS, Golden SH, Anderson C, et al. Update on Prevention of Cardiovascular Disease in Adults With Type 2 Diabetes Mellitus in Light of Recent Evidence: A Scientific Statement From the American Heart Association and the American Diabetes Association. Circulation 2015;132:691-718. [Crossref] [PubMed]
- Garcia-Valdecasas F. The theory of the four humors (or how the old medicine healed). Med Hist (Barc) 1991.1-20. [PubMed]
- Hippocrates' Four Humors in 1893 at Chicago's Post-Graduate School of Anaesthesia. Anesthesiology 2016;125:6. [Crossref] [PubMed]
- Parapia LA. History of bloodletting by phlebotomy. Br J Haematol 2008;143:490-5. [PubMed]
- Golden RL. The centenary of Osler's "The Principles and Practice of Medicine.". Osler Libr Newsl 1992;69:1-3. [PubMed]
- Girard DE. The history of Osler's Principles and Practice of Medicine. Med Times 1981;109:1s-4s, 13s.
- Tata JR. One hundred years of hormones. EMBO Rep 2005;6:490-6. [Crossref] [PubMed]
- Targher G, Byrne CD. Circulating Markers of Liver Function and Cardiovascular Disease Risk. Arterioscler Thromb Vasc Biol 2015;35:2290-6. [Crossref] [PubMed]
- Poggesi A, Pasi M, Pescini F, et al. Circulating biologic markers of endothelial dysfunction in cerebral small vessel disease: A review. J Cereb Blood Flow Metab 2016;36:72-94. [Crossref] [PubMed]
- Qi P, Zhou XY, Du X. Circulating long non-coding RNAs in cancer: current status and future perspectives. Mol Cancer 2016;15:39. [Crossref] [PubMed]
- Xiong H, McCabe L, Costello J, et al. Activation of NR1a/NR2B receptors by soluble factors from APP-stimulated monocyte-derived macrophages: implications for the pathogenesis of Alzheimer's disease. Neurobiol Aging 2004;25:905-11. [Crossref] [PubMed]
- Conboy MJ, Conboy IM, Rando TA. Heterochronic parabiosis: historical perspective and methodological considerations for studies of aging and longevity. Aging Cell 2013;12:525-30. [Crossref] [PubMed]
- Wright DE, Wagers AJ, Gulati AP, et al. Physiological migration of hematopoietic stem and progenitor cells. Science 2001;294:1933-6. [Crossref] [PubMed]
- Coleman DL. Effects of parabiosis of obese with diabetes and normal mice. Diabetologia 1973;9:294-8. [Crossref] [PubMed]
- Coleman DL. A historical perspective on leptin. Nat Med 2010;16:1097-9. [Crossref] [PubMed]
- Conboy IM, Conboy MJ, Wagers AJ, et al. Rejuvenation of aged progenitor cells by exposure to a young systemic environment. Nature 2005;433:760-4. [Crossref] [PubMed]
- Katsimpardi L, Litterman NK, Schein PA, et al. Vascular and neurogenic rejuvenation of the aging mouse brain by young systemic factors. Science 2014;344:630-4. [Crossref] [PubMed]
- Loffredo FS, Steinhauser ML, Jay SM, et al. Growth Differentiation Factor 11 Is a Circulating Factor that Reverses Age-Related Cardiac Hypertrophy. Cell 2013;153:828-39. [Crossref] [PubMed]
- Villeda SA, Luo J, Mosher KI, et al. The ageing systemic milieu negatively regulates neurogenesis and cognitive function. Nature 2011;477:90-4. [Crossref] [PubMed]
- Villeda SA, Plambeck KE, Middeldorp J, et al. Young blood reverses age-related impairments in cognitive function and synaptic plasticity in mice. Nat Med 2014;20:659-63. [Crossref] [PubMed]
- Sinha M, Jang YC, Oh J, et al. Restoring systemic GDF11 levels reverses age-related dysfunction in mouse skeletal muscle. Science 2014;344:649-52. [Crossref] [PubMed]
- Egerman MA, Cadena SM, Gilbert JA, et al. GDF11 Increases with Age and Inhibits Skeletal Muscle Regeneration. Cell metabolism 2015;22:164-74. [Crossref] [PubMed]
- Smith SC, Zhang X, Zhang X, et al. GDF11 Does Not Rescue Aging-Related Pathological Hypertrophy. Circ Res 2015;117:926-32. [Crossref] [PubMed]
- Schafer MJ, Atkinson EJ, Vanderboom PM, et al. Quantification of GDF11 and Myostatin in Human Aging and Cardiovascular Disease. Cell metabolism 2016;23:1207-15. [Crossref] [PubMed]
- Walker RG, Poggioli T, Katsimpardi L, et al. Biochemistry and Biology of GDF11 and Myostatin: Similarities, Differences, and Questions for Future Investigation. Circ Res 2016;118:1125-41; discussion 42. [Crossref] [PubMed]
- Poggioli T, Vujic A, Yang P, et al. Circulating Growth Differentiation Factor 11 / 8 Levels Decline with Age. 2015.
- Kerola T, Kettunen R, Nieminen T. The complex interplay of cardiovascular system and cognition: how to predict dementia in the elderly? Int J Cardiol 2011;150:123-9. [Crossref] [PubMed]
- Kalaria RN. Neuropathological diagnosis of vascular cognitive impairment and vascular dementia with implications for Alzheimer's disease. Acta neuropathologica 2016;131:659-85. [Crossref] [PubMed]
- Hyman BT, Phelps CH, Beach TG, et al. National Institute on Aging-Alzheimer's Association guidelines for the neuropathologic assessment of Alzheimer's disease. Alzheimers Dement 2012;8:1-13. [Crossref] [PubMed]
- Tu S, Okamoto S, Lipton SA, et al. Oligomeric Abeta-induced synaptic dysfunction in Alzheimer's disease. Mol Neurodegener 2014;9:48. [Crossref] [PubMed]
- Hickman RA, Faustin A, Wisniewski T. Alzheimer Disease and Its Growing Epidemic: Risk Factors, Biomarkers, and the Urgent Need for Therapeutics. Neurol Clin 2016;34:941-53. [Crossref] [PubMed]
- Ruckh JM, Zhao JW, Shadrach JL, et al. Rejuvenation of regeneration in the aging central nervous system. Cell Stem Cell 2012;10:96-103. [Crossref] [PubMed]
- Sharon Sha PI, Stanford University. The PLasma for Alzheimer SymptoM Amelioration (PLASMA) Study (PLASMA). Available online: https://clinicaltrials.gov/ct2/show/NCT02256306
- LLC A. Young Plasma Clinical Trial. Available online: https://.http://www.ambrosiaplasma.com/
- Medzhitov R. Origin and physiological roles of inflammation. Nature 2008;454:428-35. [Crossref] [PubMed]
- Franceschi C, Bonafe M, Valensin S, et al. Inflamm-aging. An evolutionary perspective on immunosenescence. Ann N Y Acad Sci 2000;908:244-54. [Crossref] [PubMed]
- Wu J, Xia S, Kalionis B, et al. The role of oxidative stress and inflammation in cardiovascular aging. Biomed Res Int 2014;2014:615312.
- Bruunsgaard H, Pedersen BK. Age-related inflammatory cytokines and disease. Immunol Allergy Clin North Am 2003;23:15-39. [Crossref] [PubMed]
- Jenny NS. Inflammation in aging: cause, effect, or both? Discov Med 2012;13:451-60. [PubMed]
- Linton PJ, Gurney M, Sengstock D, et al. This old heart: Cardiac aging and autophagy. J Mol Cell Cardiol 2015;83:44-54. [Crossref] [PubMed]
- De la Fuente M, Miquel J. An update of the oxidation-inflammation theory of aging: the involvement of the immune system in oxi-inflamm-aging. Curr Pharm Des 2009;15:3003-26. [Crossref] [PubMed]
- Hosford-Donovan A, Nilsson A, Wahlin-Larsson B, et al. Observational and mechanistic links between C-reactive protein and blood pressure in elderly women. Maturitas 2016;89:52-7. [Crossref] [PubMed]
- Maggio M, Guralnik JM, Longo DL, et al. Interleukin-6 in aging and chronic disease: a magnificent pathway. J Gerontol A Biol Sci Med Sci 2006;61:575-84. [Crossref] [PubMed]
- Ock S, Lee WS, Ahn J, et al. Deletion of IGF-1 Receptors in Cardiomyocytes Attenuates Cardiac Aging in Male Mice. Endocrinology 2016;157:336-45. [Crossref] [PubMed]
- Volpato S, Guralnik JM, Ferrucci L, et al. Cardiovascular disease, interleukin-6, and risk of mortality in older women: the women's health and aging study. Circulation 2001;103:947-53. [Crossref] [PubMed]
- Ridker PM. A Test in Context: High-Sensitivity C-Reactive Protein. J Am Coll Cardiol 2016;67:712-23. [Crossref] [PubMed]
- Ogita M, Miyauchi K. C-reactive protein and cardiovascular disease. J Cardiol 2016;68:179. [Crossref] [PubMed]
- Herbeth B, Siest G, Henny J. High sensitivity C-reactive protein (CRP) reference intervals in the elderly. Clin Chem Lab Med 2001;39:1169-70.
- Assuncao LG, Eloi-Santos SM, Peixoto SV. High sensitivity C-reactive protein distribution in the elderly: the Bambui Cohort Study, Brazil. Braz J Med Biol Res 2012;45:1284-6. [Crossref] [PubMed]
- Ridker PM. From C-Reactive Protein to Interleukin-6 to Interleukin-1: Moving Upstream To Identify Novel Targets for Atheroprotection. Circ Res 2016;118:145-56. [Crossref] [PubMed]
- Passacquale G, Di Giosia P, Ferro A. The role of inflammatory biomarkers in developing targeted cardiovascular therapies: lessons from the cardiovascular inflammation reduction trials. Cardiovasc Res 2016;109:9-23. [Crossref] [PubMed]
- Kaplan SA, Johnson-Levonas AO, Lin J, et al. Elevated high sensitivity C-reactive protein levels in aging men with low testosterone. Aging Male 2010;13:108-12. [Crossref] [PubMed]
- Holick MF. Vitamin D: a d-lightful solution for health. J Investig Med 2011;59:872-80. [Crossref] [PubMed]
- Wang TJ, Pencina MJ, Booth SL, et al. Vitamin D deficiency and risk of cardiovascular disease. Circulation 2008;117:503-11. [Crossref] [PubMed]
- Mandarino NR, Junior F, Salgado JV, et al. Is vitamin d deficiency a new risk factor for cardiovascular disease? Open Cardiovasc Med J 2015;9:40-9. [Crossref] [PubMed]
- Prasad P, Kochhar A. Interplay of vitamin D and metabolic syndrome: A review. Diabetes Metab Syndr 2016;10:105-12. [Crossref] [PubMed]
- Holick MF. Vitamin D deficiency. N Engl J Med 2007;357:266-81. [Crossref] [PubMed]
- Lee S, Ahuja V, Masaki K, et al. A Significant Positive Association of Vitamin D Deficiency with Coronary Artery Calcification among Middle-aged Men: For the ERA JUMP Study. J Am Coll Nutr 2016;35:614-20. [Crossref] [PubMed]
- Masson S, Agabiti N, Vago T, et al. The fibroblast growth factor-23 and Vitamin D emerge as nontraditional risk factors and may affect cardiovascular risk. J Intern Med 2015;277:318-30. [Crossref] [PubMed]
- Thompson J, Nitiahpapand R, Bhatti P, et al. Vitamin D deficiency and atrial fibrillation. Int J Cardiol 2015;184:159-62. [Crossref] [PubMed]
- Karakis I, Pase MP, Beiser A, et al. Association of Serum Vitamin D with the Risk of Incident Dementia and Subclinical Indices of Brain Aging: The Framingham Heart Study. J Alzheimers Dis 2016;51:451-61. [Crossref] [PubMed]
- Meehan M, Penckofer S. The Role of Vitamin D in the Aging Adult. J Aging Gerontol 2014;2:60-71. [Crossref] [PubMed]
- Kestenbaum B, Katz R, de Boer I, et al. Vitamin D, parathyroid hormone, and cardiovascular events among older adults. J Am Coll Cardiol 2011;58:1433-41. [Crossref] [PubMed]
- Siadat ZD, Kiani K, Sadeghi M, et al. Association of vitamin D deficiency and coronary artery disease with cardiovascular risk factors. J Res Med Sci 2012;17:1052-5. [PubMed]
- Richards JB, Valdes AM, Gardner JP, et al. Higher serum vitamin D concentrations are associated with longer leukocyte telomere length in women. Am J Clin Nutr 2007;86:1420-5. [PubMed]
- Chatterjee K. Neurohormonal activation in congestive heart failure and the role of vasopressin. Am J Cardiol 2005;95:8B-13B. [Crossref] [PubMed]
- Esler MD, Turner AG, Kaye DM, et al. Aging effects on human sympathetic neuronal function. Am J Physiol 1995;268:R278-85. [PubMed]
- von Lueder TG, Krum H. New medical therapies for heart failure. Nat Rev Cardiol 2015;12:730-40. [Crossref] [PubMed]
- Mentz RJ, O'Connor CM. Pathophysiology and clinical evaluation of acute heart failure. Nat Rev Cardiol 2016;13:28-35. [Crossref] [PubMed]
- Grodin JL. Pharmacologic Approaches to Electrolyte Abnormalities in Heart Failure. Curr Heart Fail Rep 2016;13:181-9. [Crossref] [PubMed]
- Goldsmith SR. A new approach to treatment of acute heart failure. J Cardiol 2016;67:395-8. [Crossref] [PubMed]
- McMurray JJ, Adamopoulos S, Anker SD, et al. ESC Guidelines for the diagnosis and treatment of acute and chronic heart failure 2012: The Task Force for the Diagnosis and Treatment of Acute and Chronic Heart Failure 2012 of the European Society of Cardiology. Developed in collaboration with the Heart Failure Association (HFA) of the ESC. European Heart Journal 2012;33:1787-847. [Crossref] [PubMed]
- Mollace V, Gliozzi M, Capuano A, et al. Modulation of RAAS-natriuretic peptides in the treatment of HF: Old guys and newcomers. Int J Cardiol 2017;226:126-31. [Crossref] [PubMed]
- Cermakova P, Eriksdotter M, Lund LH, et al. Heart failure and Alzheimer's disease. J Intern Med 2015;277:406-25. [Crossref] [PubMed]
- Domenighetti AA, Wang Q, Egger M, et al. Angiotensin II-mediated phenotypic cardiomyocyte remodeling leads to age-dependent cardiac dysfunction and failure. Hypertension 2005;46:426-32. [Crossref] [PubMed]
- Dai DF, Rabinovitch PS. Cardiac aging in mice and humans: the role of mitochondrial oxidative stress. Trends Cardiovasc Med 2009.19213-20. [PubMed]
- Wang M, Zhang J, Walker SJ, et al. Involvement of NADPH oxidase in age-associated cardiac remodeling. J Mol Cell Cardiol 2010;48:765-72. [Crossref] [PubMed]
- Kovacs A, Fulop GA, Kovacs A, et al. Renin overexpression leads to increased titin-based stiffness contributing to diastolic dysfunction in hypertensive mRen2 rats. Am J Physiol Heart Circ Physiol 2016;310:H1671-82. [Crossref] [PubMed]
- Nakayama H, Nishida K, Otsu K. Macromolecular Degradation Systems and Cardiovascular Aging. Circ Res 2016;118:1577-92. [Crossref] [PubMed]
- Zhu D, Shi J, Zhang Y, et al. Central angiotensin II stimulation promotes beta amyloid production in Sprague Dawley rats. PloS one 2011;6:e16037. [Crossref] [PubMed]
- Troncone L, Luciani M, Coggins M, et al. Abeta Amyloid Pathology Affects the Hearts of Patients With Alzheimer's Disease: Mind the Heart. J Am Coll Cardiol 2016;68:2395-407. [Crossref] [PubMed]
- Zhou J, Xu X, Liu JJ, et al. Angiotensin II receptors subtypes mediate diverse gene expression profile in adult hypertrophic cardiomyocytes. Clin Exp Pharmacol Physiol 2007;34:1191-8. [PubMed]
- Dai DF, Johnson SC, Villarin JJ, et al. Mitochondrial oxidative stress mediates angiotensin II-induced cardiac hypertrophy and Galphaq overexpression-induced heart failure. Circ Res 2011;108:837-46. [Crossref] [PubMed]
- Dai DF, Chen T, Szeto H, et al. Mitochondrial targeted antioxidant Peptide ameliorates hypertensive cardiomyopathy. J Am Coll Cardiol 2011;58:73-82. [Crossref] [PubMed]
- Zorov DB, Filburn CR, Klotz LO, et al. Reactive oxygen species (ROS)-induced ROS release: a new phenomenon accompanying induction of the mitochondrial permeability transition in cardiac myocytes. J Exp Med 2000;192:1001-14. [Crossref] [PubMed]
- Somanna NK, Valente AJ, Krenz M, et al. The Nox1/4 Dual Inhibitor GKT137831 or Nox4 Knockdown Inhibits Angiotensin-II-Induced Adult Mouse Cardiac Fibroblast Proliferation and Migration. AT1 Physically Associates With Nox4. J Cell Physiol 2016;231:1130-41. [Crossref] [PubMed]
- Wu Z, Yu Y, Liu C, et al. Role of p38 mitogen-activated protein kinase in vascular endothelial aging: interaction with Arginase-II and S6K1 signaling pathway. Aging (Albany NY) 2015;7:70-81. [Crossref] [PubMed]
- Hale TM. Persistent phenotypic shift in cardiac fibroblasts: impact of transient renin angiotensin system inhibition. J Mol Cell Cardiol 2016;93:125-32. [Crossref] [PubMed]
- Pringle KG, Sykes SD, Lumbers ER. Circulating and intrarenal renin-angiotensin systems in healthy men and nonpregnant women. Physiol Rep 2015;3:e12586. [Crossref] [PubMed]
- Ziff OJ, Covic A, Goldsmith D. Calibrating the impact of dual RAAS blockade on the heart and the kidney - balancing risks and benefits. Int J Clin Pract 2016;70:537-53. [Crossref] [PubMed]
- Bolignano D, Mattace-Raso F, Sijbrands EJ, et al. The aging kidney revisited: a systematic review. Ageing Res Rev 2014;14:65-80. [Crossref] [PubMed]
- Buglioni A, Burnett JC Jr. Pathophysiology and the cardiorenal connection in heart failure. Circulating hormones: biomarkers or mediators. Clin Chim Acta 2015;443:3-8. [Crossref] [PubMed]
- Aroor AR, Demarco VG, Jia G, et al. The role of tissue Renin-Angiotensin-aldosterone system in the development of endothelial dysfunction and arterial stiffness. Front Endocrinol (Lausanne) 2013;4:161. [Crossref] [PubMed]
- Favre GA, Esnault VL, Van Obberghen E. Modulation of glucose metabolism by the renin-angiotensin-aldosterone system. Am J Physiol Endocrinol Metab. 2015;308:E435-49. [Crossref] [PubMed]
- Lopez-Otin C, Blasco MA, Partridge L, et al. The hallmarks of aging. Cell 2013;153:1194-217. [Crossref] [PubMed]
- Chiao YA, Rabinovitch PS. The Aging Heart. Cold Spring Harb Perspect Med 2015;5:a025148. [Crossref] [PubMed]
- Basso N, Cini R, Pietrelli A, et al. Protective effect of long-term angiotensin II inhibition. Am J Physiol Heart Circ Physiol 2007;293:H1351-8. [Crossref] [PubMed]
- Group TCTS. Effects of enalapril on mortality in severe congestive heart failure. Results of the Cooperative North Scandinavian Enalapril Survival Study (CONSENSUS). N Engl J Med 1987;316:1429-35. [Crossref] [PubMed]
- Buford TW, Manini TM, Hsu FC, et al. Angiotensin-converting enzyme inhibitor use by older adults is associated with greater functional responses to exercise. J Am Geriatr Soc 2012;60:1244-52. [Crossref] [PubMed]
- Inserra F, Romano L, Ercole L, et al. Cardiovascular changes by long-term inhibition of the renin-angiotensin system in aging. Hypertension 1995;25:437-42. [Crossref] [PubMed]
- Su JB. Vascular endothelial dysfunction and pharmacological treatment. World J Cardiol 2015;7:719-41. [Crossref] [PubMed]
- Benigni A, Corna D, Zoja C, et al. Disruption of the Ang II type 1 receptor promotes longevity in mice. J Clin Invest 2009;119:524-30. [Crossref] [PubMed]
- Ponikowski P, Voors AA, Anker SD, et al. 2016 ESC Guidelines for the diagnosis and treatment of acute and chronic heart failure: The Task Force for the diagnosis and treatment of acute and chronic heart failure of the European Society of Cardiology (ESC). Developed with the special contribution of the Heart Failure Association (HFA) of the ESC. Eur J Heart Fail 2016;18:891-975. [Crossref] [PubMed]
- Yancy CW, Jessup M, Bozkurt B, et al. 2016 ACC/AHA/HFSA Focused Update on New Pharmacological Therapy for Heart Failure: An Update of the 2013 ACCF/AHA Guideline for the Management of Heart Failure: A Report of the American College of Cardiology/American Heart Association Task Force on Clinical Practice Guidelines and the Heart Failure Society of America. J Am Coll Cardiol 2016;68:1476-88. [Crossref] [PubMed]
- Lipsitz LA, Habtemariam D, Gagnon M, et al. Reexamining the Effect of Antihypertensive Medications on Falls in Old Age. Hypertension 2015;66:183-9. [Crossref] [PubMed]
- George CJ, Verghese J. Gait Performance in Hypertensive Patients on Angiotensin-Converting Enzyme Inhibitors. J Am Med Dir Assoc 2016;17:737-40. [Crossref] [PubMed]
- Zhou LS, Xu LJ, Wang XQ, et al. Effect of Angiotensin-Converting Enzyme Inhibitors on Physical Function in Elderly Subjects: A Systematic Review and Meta-Analysis. Drugs Aging 2015;32:727-35. [Crossref] [PubMed]
- d'Uscio LV, Shaw S, Barton M, et al. Losartan but not verapamil inhibits angiotensin II-induced tissue endothelin-1 increase: role of blood pressure and endothelial function. Hypertension 1998;31:1305-10. [Crossref] [PubMed]
- Harvey A, Montezano AC, Lopes RA, et al. Vascular Fibrosis in Aging and Hypertension: Molecular Mechanisms and Clinical Implications. Can J Cardiol 2016;32:659-68. [Crossref] [PubMed]
- Donato AJ, Gano LB, Eskurza I, et al. Vascular endothelial dysfunction with aging: endothelin-1 and endothelial nitric oxide synthase. Am J Physiol Heart Circ Physiol 2009;297:H425-32. [Crossref] [PubMed]
- Park JB, Schiffrin EL. ET. (A) receptor antagonist prevents blood pressure elevation and vascular remodeling in aldosterone-infused rats. Hypertension 2001;37:1444-9. [Crossref] [PubMed]
- Ammarguellat FZ, Gannon PO, Amiri F, et al. Fibrosis, matrix metalloproteinases, and inflammation in the heart of DOCA-salt hypertensive rats: role of ET(A) receptors. Hypertension 2002;39:679-84. [Crossref] [PubMed]
- Zepeda R, Contreras V, Pissani C, et al. Venlafaxine treatment after endothelin-1-induced cortical stroke modulates growth factor expression and reduces tissue damage in rats. Neuropharmacology 2016;107:131-45. [Crossref] [PubMed]
- Francis GS, Felker GM, Tang WH. A Test in Context: Critical Evaluation of Natriuretic Peptide Testing in Heart Failure. J Am Coll Cardiol 2016;67:330-7. [Crossref] [PubMed]
- Volpe M, Carnovali M, Mastromarino V. The natriuretic peptides system in the pathophysiology of heart failure: from molecular basis to treatment. Clin Sci (Lond) 2016;130:57-77. [Crossref] [PubMed]
- Torres-Courchoud I, Chen HH. B-type natriuretic peptide and acute heart failure: Fluid homeostasis, biomarker and therapeutics. Rev Clin Esp 2016;216:393-8. [Crossref] [PubMed]
- Zhu Q, Xiao W, Bai Y, et al. The prognostic value of the plasma N-terminal pro-brain natriuretic peptide level on all-cause death and major cardiovascular events in a community-based population. Clin Interv Aging 2016;11:245-53. [Crossref] [PubMed]
- Santaguida PL, Don-Wauchope AC, Oremus M, et al. BNP and NT-proBNP as prognostic markers in persons with acute decompensated heart failure: a systematic review. Heart Fail Rev 2014;19:453-70. [Crossref] [PubMed]
- Sarzani R, Spannella F, Giulietti F, et al. NT-proBNP and Its Correlation with In-Hospital Mortality in the Very Elderly without an Admission Diagnosis of Heart Failure. PloS one 2016;11:e0153759. [Crossref] [PubMed]
- Lelli D, Pedone C, Rossi FF, et al. Clinical and echocardiographic characteristics of elderly hospitalized patients with high levels of NT-proBNP without clinical diagnosis of heart failure. Aging Clin Exp Res 2014;26:607-13. [Crossref] [PubMed]
- Nadrowski P, Chudek J, Grodzicki T, et al. Plasma level of N-terminal pro brain natriuretic peptide (NT-proBNP) in elderly population in Poland--the PolSenior Study. Exp Gerontol 2013;48:852-7. [Crossref] [PubMed]
- Redfield MM, Rodeheffer RJ, Jacobsen SJ, et al. Plasma brain natriuretic peptide concentration: impact of age and gender. J Am Coll Cardiol 2002;40:976-82. [Crossref] [PubMed]
- Chen C, Sung KT, Shih SC, et al. Age, Gender and Load-Related Influences on Left Ventricular Geometric Remodeling, Systolic Mid-Wall Function, and NT-ProBNP in Asymptomatic Asian Population. PloS one 2016;11:e0156467. [Crossref] [PubMed]
- Potter LR. Domain analysis of human transmembrane guanylyl cyclase receptors: implications for regulation. Front Biosci 2005;10:1205-20. [Crossref] [PubMed]
- Charles CJ, Espiner EA, Nicholls MG, et al. Clearance receptors and endopeptidase 24.11: equal role in natriuretic peptide metabolism in conscious sheep. Am J Physiol 1996;271:R373-80. [PubMed]
- Thomas CJ, McAllen RM, Salo LM, et al. Restorative effect of atrial natriuretic peptide or chronic neutral endopeptidase inhibition on blunted cardiopulmonary vagal reflexes in aged rats. Hypertension 2008;52:696-701. [Crossref] [PubMed]
- Venkatesan B, Tumala A, Subramanian V, et al. Transient silencing of Npr3 gene expression improved the circulatory levels of atrial natriuretic peptides and attenuated beta-adrenoceptor activation- induced cardiac hypertrophic growth in experimental rats. Eur J Pharmacol 2016;782:44-58. [Crossref] [PubMed]
- McMurray JJ, Packer M, Desai AS, et al. Angiotensin-neprilysin inhibition versus enalapril in heart failure. N Engl J Med 2014;371:993-1004. [Crossref] [PubMed]
- Claggett B, Packer M, McMurray JJ, et al. Estimating the Long-Term Treatment Benefits of Sacubitril-Valsartan. N Engl J Med 2015;373:2289-90. [Crossref] [PubMed]
- Wagner KH, Cameron-Smith D, Wessner B, et al. Biomarkers of Aging: From Function to Molecular Biology. Nutrients 2016;8:E338. [Crossref] [PubMed]
- Vegter EL, van der Meer P, de Windt LJ, et al. MicroRNAs in heart failure: from biomarker to target for therapy. Eur J Heart Fail 2016;18:457-68. [Crossref] [PubMed]
- Olivieri F, Bonafe M, Spazzafumo L, et al. Age- and glycemia-related miR-126-3p levels in plasma and endothelial cells. Aging (Albany NY) 2014;6:771-87. [Crossref] [PubMed]
- McGregor RA, Poppitt SD, Cameron-Smith D. Role of microRNAs in the age-related changes in skeletal muscle and diet or exercise interventions to promote healthy aging in humans. Ageing Res Rev 2014;17:25-33. [Crossref] [PubMed]
- Sayed D, Hong C, Chen IY, et al. MicroRNAs play an essential role in the development of cardiac hypertrophy. Circ Res 2007;100:416-24. [Crossref] [PubMed]
- Kumarswamy R, Thum T. Non-coding RNAs in cardiac remodeling and heart failure. Circ Res. 2013;113:676-89. [Crossref] [PubMed]
- Agostini M, Knight RA. miR-34: from bench to bedside. Oncotarget 2014;5:872-81. [Crossref] [PubMed]
- Chiao YA. MicroRNA-34a: a new piece in the cardiac aging puzzle. Circ Cardiovasc Genet 2013;6:437-8. [Crossref] [PubMed]
- Boon RA, Iekushi K, Lechner S, et al. MicroRNA-34a regulates cardiac ageing and function. Nature 2013;495:107-10. [Crossref] [PubMed]
- Loffredo FS, Pancoast JR, Lee RT. Keep PNUTS in your heart. Circ Res 2013;113:97-9. [Crossref] [PubMed]
- Djebali S, Davis CA, Merkel A, et al. Landscape of transcription in human cells. Nature. 2012;489:101-8. [Crossref] [PubMed]
- Guttman M, Amit I, Garber M, et al. Chromatin signature reveals over a thousand highly conserved large non-coding RNAs in mammals. Nature 2009;458:223-7. [Crossref] [PubMed]
- Wang H, Bei Y, Shi J, et al. Non-Coding RNAs in Cardiac Aging. Cell Physiol Biochem 2015;36:1679-87. [Crossref] [PubMed]
- Devaux Y, Zangrando J, Schroen B, et al. Long noncoding RNAs in cardiac development and ageing. Nat Rev Cardiol 2015;12:415-25. [Crossref] [PubMed]
- Piccoli MT, Bar C, Thum T. Non-coding RNAs as modulators of the cardiac fibroblast phenotype. J Mol Cell Cardiol 2016;92:75-81. [Crossref] [PubMed]
- Wang G, Lunardi A, Zhang J, et al. Zbtb7a suppresses prostate cancer through repression of a Sox9-dependent pathway for cellular senescence bypass and tumor invasion. Nat Genet 2013;45:739-46. [Crossref] [PubMed]
- Ratajczak MZ. Igf2-H19, an imprinted tandem gene, is an important regulator of embryonic development, a guardian of proliferation of adult pluripotent stem cells, a regulator of longevity, and a 'passkey' to cancerogenesis. Folia Histochem Cytobiol 2012;50:171-9. [Crossref] [PubMed]
- Kaneko M, Satomi T, Fujiwara S, et al. AT1 receptor blocker Azilsartan Medoxomil normalizes plasma miR-146a and miR-342-3p in a murine heart failure model. Biomarkers 2016.1-8. [Crossref] [PubMed]