Role of CXC group chemokines in lung cancer development and progression
Introduction
Lung cancer is by far the leading cause of cancer death among both men and women; about one out of four cancer deaths are from lung cancer (1). Understanding of cancerogenesis requires a holistic approach including, analysis of epidemiological and clinical data, molecular testing of blood biomarkers and thorough analysis of tumor cells and immune cell infiltrate of the tumor. Data acquired over the last few decades has confirmed heterogeneity of cancer as a disease (2) which indicates there is no single perfect biomarker for lung cancer, but a panel of multiple parameters (3,4) could be a much more objective diagnostic and prognostic tool.
Solid tumors like lung cancer contain in addition to tumor cells, also various types of stromal cells, such as fibroblasts and endothelial cells. Moreover, tumors are infiltrated by inflammatory cells, including neutrophils, macrophages and lymphocytes (5). Tumor cells, stromal cells, as well as the tumor-associated leukocytes contribute to the local production of chemokines inside the tumor and affect systemic circulating chemokine levels (Figure 1).
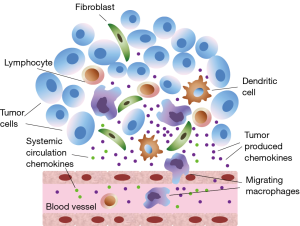
In our study we have focused on a group of CXC chemokines, which are members of a big family of cytokines undoubtedly involved in tumor growth regulation and metastasizing pathways (6). Previous research by our group has confirmed diagnostic and prognostic value of CXC chemokines as biomarkers (7,8).
Clinical and translational research on lung cancer patients undergoing surgical treatment can provide valuable scientific data and unique opportunity to study tumor microenvironment. For better understanding of CXC chemokine involvement in the process of carcinogenesis we have studied the cohort of early stage non-small cell lung cancer patients undergoing surgery with curative intent. Our aim was to assess CXC chemokine ligand (CXCL) levels in patient blood samples representing systemic and tumor microenvironment; and subsequently calculate chemokine concentration gradients in blood after passing through tumor vessel bed in order to determine chemokine involvement in the process of cancerogenesis. To ensure holistic approach our additional goal was to determine CXC chemokine receptor (CXCR) expression intensity in tumor tissue, and assess composition of tumor immune cell (TIC) infiltrate.
Methods
Patients
Between June 2010 and December 2011, patients with NSCLC (n=54) who had radical lung resection (open lobectomy with lymphadenectomy) and postoperative clinical, radiological and pathological stage IA−IIB adenocarcinoma (n=26) or squamous lung cancer (n=28) were enrolled in a single center prospective study. There were 18 women in the cohort. All patients had no neoadjuvant and/or adjuvant therapy. Patients were followed-up annually after surgery for up to six years. General status of the patient and the information about disease outcome and relapse was obtained during follow-up visits, and confirmed with the National Cancer registry. The final collection of data in September 2016 showed relapse in 24 patients (44%).
Procedure
During surgical procedure peripheral blood samples were taken from cubital vein into 5 mL vacutainer tubes using standard phlebotomy technique, simultaneously tumor draining blood samples were collected from pulmonary vein of lung lobe being resected (9). Tubes were centrifuged at room temperature for 10 minutes at 1,300 g. Following centrifugation plasma was immediately archived at –70 °C pending utilization. Prior to ELISA assay samples were defrosted at room temperature and processed according to ELISA kit manufacturer protocol. CXCL1, CXCL4, CXCL5, CXCL6, CXCL7, CXCL8, CXCL9, CXCL10, CXCL11 and CXCL12 levels were determined by ELISA kit with sensitivity 1 pg/mL and detection range 1–15,000 pg/mL (Raybiotech, USA, USA). Each standard or sample was assayed in duplicate. The CXC chemokine concentrations in plasma were calculated from the standard curve. Chemokine levels are given as mean with 95% confidence intervals.
Difference in circulating chemokine concentrations between systemic and tumor vascular beds can be described as chemokine concentration gradient. Chemokine gradient was calculated according to the formula: [(TCV-PCV)/PCV] ×100, where TCV—tumor circulation representing value, PCV—peripheral circulation representing value.
TCV and PCV values were compared using two-tailed paired t-test. CXC chemokine level gradients were compared between patients with remission and patients with relapse using unpaired two-tailed t-test.
Expression of CXCR in tumor tissue and TIC infiltrate were assessed by immunohistochemistry. Resected tumor tissue samples were fixed in formalin and embedded in paraffin. Samples were cut into 3–4 µm sections, dried and dewaxed. The slides were then incubated in hematoxylin and washed in distilled water, followed by incubation in acidified eosin solution and washing. The hematoxylin and eosin stained tumor sections were assessed by two independent pathologists to determine histological subtype of the tumor and for differentiating the tumor cells from tumor stroma. Verified tumor specimens containing representative tumor and tumor stroma tissue were further processed—dewaxed slides covered with 0.5% trypsin solution were placed in a humidified container and then into the 37 °C incubator for 10 minutes. Slides were washed in water/tris-buffered saline (TBS) and blocked in 10% normal serum with 1% bovine serum albumin (BSA) in TBS for 2 hours at room temperature. We followed the specific standardized protocol supplied by the manufacturer (Santa Cruz Biotechnology, USA). Omission of the primary antibody served as a negative control. Primary antibody (Table 1) diluted in TBS with 1% BSA applied and slides incubated for 30 minutes. Slides were washed and secondary antibody diluted to the concentration recommended by the manufacturer in TBS with 1% BSA was applied to the slides, and incubated for 1 hour at room temperature. Slides were rinsed in TBS and developed with chromogen diaminobenzidine (DAB) for 10 minutes at room temperature, rinsed in distilled water, counterstained with hematoxylin. Slides were dehydrated, cleared and mounted.
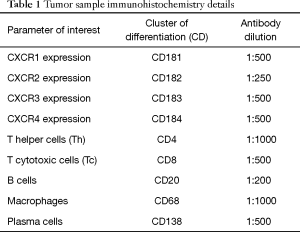
Full table
TIC infiltrate was defined as composition of immune cells including granulocytes, macrophages, plasma cells, T helper cells, T cytotoxic cells and B cells in tumor stroma and tumor tissue. The average number of positively stained cells per four high power fields was calculated. In order to provide an overview of CXCR expression patterns, staining intensity of tumor cells and tumor stromal cells was assessed using semi-quantitative score (negative =0, weak =1, moderate =2 or strong =3).
Associations between variables were analyzed with Pearson correlation test, and P values less than 0.05 marked as significant.
This study has been approved by Pauls Stradins Clinical University Hospital Foundation ethical committee (Nr. 500210-4L), and was conducted in accordance with the ethical standards of the Helsinki Declaration of the World Medical Association. The informed consent was obtained from all patients.
Results
Initially we have compared chemokine concentration in paired blood samples (n=54) representing systemic and tumor bed circulation; chemokine concentration gradients were calculated accordingly and showed in Table 2. Statistically significant decrease in chemokine concentration was found for CXCL4 (P=0.002) and CXCL5 (P=0.011), and statistically significant concentration increase was found for CXCL7 (P=0.001).
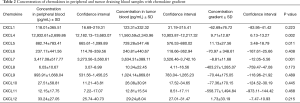
Full table
For further analysis we have divided patients according to the follow-up data into two groups based on cancer recurrence. Chemokine levels and concentration gradients are showed in Table 3 for patients with no recurrence (n=30) and Table 4 for patients with relapse (n=24) accordingly.
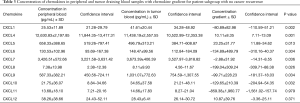
Full table
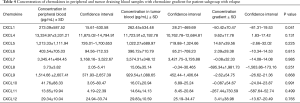
Full table
Tumor tissue samples from 40 patients undergone full immunohistochemical analysis after initial evaluation by pathologists, other samples were excluded due to staining artifacts and excessive tumor heterogeneity. Further analysis comprised of quantification of immune cells infiltrating tumor revealing prevalence of T cytotoxic cells and macrophages followed by T helper cells, B cells and plasma cells (Table 5).
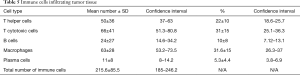
Full table
Immunohistochemistry showed moderate to strong expression intensity of CXCR1 (2.71±0.73) and CXCR3 (2.23±1.3); however expression intensity of CXCR2 (0.71±0.92) and CXCR4 (0.8±0.91) was weak. Dividing patients into subgroups according to recurrence status did not show any statistically significant difference, except for CXCR4. Patients with relapse had higher CXCR4 expression in tumor tissue (1.09±1.14), than patients with no relapse (0.57±0.65), P=0.045.
CXCR1 expression intensity correlated with tumor infiltration by macrophages (r=–0.63, P=0.044) and total number of tumor infiltrating immune cells (r=–0.37, P=0.049). CXCR2 expression intensity correlated with levels of systemic CXCL1 (r=0.7, P=0.025) and CXCL5 (r=–0.39, P=0.05); tumor infiltration by plasma cells (r=–0.52, P=0.018). CXCR3 expression intensity correlated with levels of systemic CXCL11 (r=–0.41, P=0.049); tumor infiltration by B cells (r=–0.45, P=0.01), T helper cells (r=–0.52, P=0.01) and T cytotoxic cells (r=–0.4, P=0.03).
CXCL1 gradient correlated with absolute number of B cells (r=–0.41, P=0.048); CXCL4 gradient correlated with absolute number of macrophages (r=–0.44, P=0.02) and total number of tumor infiltrating immune cells (r=0.59, P=0.01); CXCL5 gradient correlated with absolute number of T helper cells (r=0.53, P=0.008), percentage of T helper (r=0.5, P=0.01) and T cytotoxic (r=–0.46, P=0.038) cells in infiltrate. CXCL6 gradient correlated with absolute number of B cells (r=–0.54, P=0.02); CXCL7 gradient correlated with absolute number of T helper cells (r=0.49, P=0.03); CXCL8 gradient correlated with absolute number of T cytotoxic cell (r=0.43, P=0.035) and total number of tumor infiltrating cells (r=0.4, P=0.05). There was no statistically significant correlation between CXCL gradients and immune cell subpopulations
Discussion
Chemokines or chemotactic cytokines, and their receptors have been discovered as essential and selective mediators in leukocyte migration to inflammatory sites. Besides their functions in the immune system, they also play a critical role in tumor initiation, promotion and progression.
CXC chemokines are characteristically heparin binding proteins. On a structural level, they have four highly conserved cysteine amino acid residues, with the first two cysteines separated by one non-conserved aminoacid residue, hence the name CXC (10). Chemokines, which are structurally and functionally similar to growth factors, bind to G protein-coupled receptors on leukocytes and stem cells and process guanine nucleotide-binding proteins to initiate intracellular signaling cascades that promote migration towards the chemokine source (11). Although the CXC motif distinguishes this family from other chemokine families, a second structural domain within this family dictates their angiogenic potential. The NH2-terminus of the majority of the CXC chemokines containing three aminoacid residues (Glu-Leu-Arg: the ELR” motif) precedes the first cysteine aminoacid residue of the primary structure of these cytokines (12). The family members that contain the ELR motif (ELR+) are potent promoters of angiogenesis (13). In contrast, members that lack the ELR motif (ELR–) are potent inhibitors of angiogenesis. This difference suggests on a structural/functional level that members of the chemokine family are unique cytokines in their ability to behave in a disparate manner in the regulation of chemotaxis and angiogenesis. Most, if not all chemokines activate leukocytes through binding to G protein-coupled seven transmembrane receptors (GPCR) designated CXCR or CCR. The binding of a chemokine to its receptor results in the migration of immune cells by interactions with selectins and integrins. Subsequently, leukocytes infiltrate the tissue in response to a gradient of chemokines, produced at the site of inflammation. In addition, these GPCRs may account for the angiogenic or angiostatic action of chemokines. Chemokine characteristics and effects are summarized in Table 6.
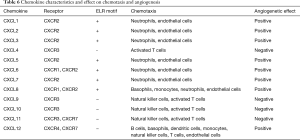
Full table
Chemokines are best known for inducing directional cell migration, particularly of leukocytes during inflammation. Prolonged inflammation is thought to facilitate carcinogenesis by providing a microenvironment that is ideal for tumor cell development and growth. Chemokines can stimulate or inhibit tumor development in an autocrine fashion by attracting cells with pro- and anti-tumoral activities. Chemokines affect tumor development indirectly by influencing angiogenesis, tumor-leukocyte interactions, as well as directly by influencing tumor transformation, survival and growth, invasion and metastasis (14). The role played by chemokines is rather complex as some chemokines may favor tumor growth and progression, while others may enhance anti-tumor immunity.
Biomarker gradient measurement is an important part of comprehensive analysis of the interactions between three highly complex systems—the tumor cells, the immune response and the tissue microenvironment. Our approach allows the differentially present proteins to be identified against a complex and variable background of proteomic profile. The analytic issue is reduced to determining what has changed in an individual pre- and post- passage through the affected lung to get around the problem of finding specific biomarkers in blood. Circulating CXC chemokine ligands produced in other tissues may get bound by CXCR expressed by tumor or tumor infiltrating immune cells thus decreasing concentration of CXCL in blood draining tumor. On the other hand CXCL can be produced by tumor or tumor infiltrating immune cells with following release of CXCL into blood draining tumor thus increasing concentration.
Interestingly, we have found statistically significant CXC chemokine concentration change for majority of chemokines—CXC1, CXC4, CXC5, CXC7, CXC8, CXC9 and CXC10 in a group of patients who had good clinical result after surgery with no evidence of relapse, on the other hand patients with cancer recurrence including local and systemic cancer spread did not show any change of chemokine concentration in blood except for CXCL1. These findings could be explained by immunoediting theory (15,16) where patients with no recurrence are going through tumor elimination or equilibrium phase with an active involvement of immune cells and chemokines bound or produced by tumor or immune cell infiltrate. Patients going through the next tumor development phase, which is escape phase, experience immunosuppression which allows tumor spread.
We have also found that chemokine levels and gradients correlate with CXCR receptor expression and number of tumor infiltrating immune cell subpopulations, which subsequently can facilitate development of immunoscore for lung cancer.
Major study limitations were performing the study in single institution and effect of surgical intervention on chemokine levels. At the moment of patient enrolment in the study in 2010 the main surgical technique for anatomical lung resection used in our institution was open approach via muscle sparing lateral thoracotomy which was rapidly replaced by VATS approach over the following 5 years. Any surgical intervention unavoidably causes release of chemokines and cytokines into systemic circulation from damaged tissue (17). Taking into account minimal trauma to soft tissue due to small incisions in case of VATS it could be hypothesized that VATS could cause less prominent tissue trauma cytokine influx into systemic circulation with potentially less influence on target chemokine concentrations.
Conclusions
Biomarker discovery strategy can be more productive when testing biomarker panels which are designed based on our knowledge about the process of carcinogenesis rather than randomly testing human proteome with preference given to quantity over quality.
Assessment of tumor microcirculation is useful for evaluation of different types of circulating biomarkers and application of our method can be very wide, integrating thoracic surgeons into translational cancer research.
Acknowledgements
The author thanks Dr. Jazeps Basko and Dr. Ainis Pirtnieks for sharing surgical skills; Dr. Darja Svirina and Dr. Jurijs Nazarovs for sharing secrets of pathohistology; Prof. Genadijs Ambalovs, Dr. Inta Jaunalksne and Prof. Dainis Krievins for general and financial support; Dimple Chudasama for language editing assistance; Dr. Evija Vilde and Jelena Serova for technical help; loving wife Irina and son Daniel for support and motivation.
Funding: This work was supported by Pauls Stradins Clinical University Hospital, State Research program in Medicine and Grant from Latvian science council (Nr. 008/04-2011).
Footnote
Conflicts of Interest: The author has no conflicts of interest to declare.
Ethical Statement: The study was approved by Pauls Stradins Clinical University Hospital Foundation ethical committee (Nr. 500210-4L) and written informed consent was obtained from all patients.
References
- Siegel RL, Miller KD, Jemal A. Cancer statistics, 2015. CA Cancer J Clin 2015;65:5-29. [Crossref] [PubMed]
- Alizadeh AA, Aranda V, Bardelli A, et al. Toward understanding and exploiting tumor heterogeneity. Nat Med 2015;21:846-53. [Crossref] [PubMed]
- Borgia JA, Basu S, Faber LP. Establishment of a multianalyte serum biomarker panel to identify lymph node metastases in nonsmall cell lung cancer. J Thorac Oncol 2009;4:338-47. [Crossref] [PubMed]
- Rinewalt D, Shersher DD, Daly S. Development of a serum biomarker panel predicting recurrence in stage I non-small cell lung cancer patients. J Thorac Cardiovasc Surg 2012;144:1344-50. [Crossref] [PubMed]
- Fridman WH, Pages F, Sautes-Fridman C, et al. The immune contexture in human tumours: impact on clinical outcome. Nat Rev Cancer 2012;12:298-306. [Crossref] [PubMed]
- Balkwill F. Cancer and the chemokine network. Nat Rev Cancer 2004;4:540-50. [Crossref] [PubMed]
- Spaks A, Svirina D, Spaka I, et al. CXC chemokine ligand 4 (CXCL4) is predictor of tumourangiogenic activity and prognostic biomarker in non-small cell lung cancer(NSCLC) patients undergoing surgical treatment. Biomarkers 2016;21:474-78. [Crossref] [PubMed]
- Spaks A, Jaunalksne I, Spaka I, et al. Diagnostic Value of Circulating CXC Chemokines in Non-small Cell Lung Cancer. Anticancer Res 2015;35:6979-83. [PubMed]
- Spaks A, Pirtnieks A, Grusina-Ujumaza J, et al. Application of Novel Methods for Non-Small Cell Lung Cancer (NSCLC) Biomarker Discovery. Acta Chir Latv 2013;13:13-6.
- Taub DD, Oppenheim JJ. Chemokines, inflammation and immune system. Ther Immunol 1994;1:229-46. [PubMed]
- Müller A, Homey B, Soto H, et al. Involvement of chemokine receptors in breast cancer metastasis. Nature 2001;410:50-6. [Crossref] [PubMed]
- Adams DH, Lloyd AR. Chemokines: Leucocyte recruitment and activation cytokines. Lancet 1997;349:490-95. [Crossref] [PubMed]
- Strieter RM, Polverini PJ, Kunkel SL, et al. The functional role of the ELR motif in CXC chemokine-mediated angiogenesis. J Biol Chem 1995;270:27348-57. [Crossref] [PubMed]
- Vandercappellen J, Liekens S, Bronckaers A, et al. The COOH-Terminal Peptide of Platelet Factor-4 Variant (CXCL4L1/PF-4var(47-70)) Strongly Inhibits Angiogenesis and Suppresses B16 Melanoma Growth In vivo. Mol Cancer Res 2010;8:322-34. [Crossref] [PubMed]
- Teng MW, Galon J, Fridman WH, et al. From mice to humans: developments in cancer immunoediting. J Clin Invest 2015;125:3338-46. [Crossref] [PubMed]
- Kim R, Emi M, Tanabe K. Cancer immunoediting from immune surveillance to immune escape. Immunology 2007;121:1-14. [Crossref] [PubMed]
- Clarke CN, Kuboki S, Tevar AA, et al. CXC chemokines play a critical role in liver injury, recovery, and regeneration. Am J Surg 2009;198:415-9. [Crossref] [PubMed]