Modern diagnostic and therapeutic interventional radiology in lung cancer
Introduction
Imaging has an important role in the multidisciplinary management of primary lung cancer, and is necessary to establish the diagnosis; localise, characterise and stage the tumour; map relevant nodal, vascular and bronchial anatomy for treatment planning; and for surveillance of treatment efficacy and development of metachronous tumours. Image-guided treatment of primary lung cancers can be performed in select cases. This article reviews the imaging modalities currently used for the evaluation of lung cancers, and discusses image-guided percutaneous interventional techniques for histopathologic diagnosis and local tumour treatment. Lung cancer screening is beyond the scope of this article.
Imaging modalities
Computed tomography (CT) is the imaging modality of choice for the initial evaluation of suspected or proven lung cancers. Positron emission tomography (PET)/CT is the most accurate imaging modality for the staging of primary lung cancers. Magnetic resonance (MR) imaging is useful for evaluation of superior sulcus (Pancoast) tumours and suspected malignant invasion of the chest wall, mediastinum or spine. The current recommended imaging required for lung cancer staging is CT scan of the thorax and PET/CT from skull base to mid-thigh (1).
Computed tomography
Advanced CT scanners permit a high-resolution, comprehensive evaluation of the entire chest in a single breath-hold lasting several seconds with an improved radiation dose profile to generate an isotropic dataset that allows detailed anatomical assessment as well as functional assessment of lung cancers. Radiation dose reduction is achieved by utilising automatic tube current modulation and iterative reconstruction techniques, which enable a CT examination to be performed either at a reduced dose with a similar image quality or at the same dose with improved image quality (2,3). Improved detection of small lung tumours is achieved by rapid acquisition and new visualisation techniques. Rapid acquisition reduces respiratory and cardiac motion artefacts that allow more accurate depiction of lung nodules, especially in the lung bases and in the para-cardiac lung. New visualisation techniques, such as maximum intensity projection, volume rendering, stereographic display and computer-aided detection, enhance lung cancer detection and enable the reader to differentiate small lung nodules from vessels (4). Isotropic dataset acquisition permits easy multiplanar reconstructions, including high-resolution angiograms and three-dimensional reconstruction of vascular and bronchial anatomy, for surgical or percutaneous interventional planning.
Staging
Despite recent advances in CT technology, lung cancer staging with CT remains suboptimal but is routinely performed because it remains excellent for local staging of T1 and T2 tumours, is able to delineate T3 and T4 tumours, guides selection of the most appropriate lymph nodes and the invasive technique for nodal sampling, and allows triage of patients to non-surgical therapy when unequivocal distant metastases are present. Limitations of CT for staging include accurate detection of early mediastinal and chest wall invasion, mediastinal staging and detection of small extrathoracic metastases. With regards to local tumour extent, differentiation between absent, minimal and gross T3 and T4 disease is of critical clinical significance as it determines whether the tumour is completely resectable and the surgical approach (5). The utility of thin-section CT has not significantly improved the detection of malignant invasion of the parietal pleura. One study of 90 patients using a 4-detector CT showed sensitivity, specificity and accuracy of 42%, 100% and 83%, respectively, for the detection of chest wall invasion (6). Another small study using a 4-detector CT showed the use of multiplanar reconstructions can improve the sensitivity, specificity and accuracy of CT to 86%, 96% and 95%, respectively, for the detection of chest wall invasion (7). With regards to malignant nodal involvement, a recent meta-analysis showed a pooled sensitivity and specificity of 55% and 81%, respectively, for the detection of malignant mediastinal lymph nodes when a widely accepted definition of normal-sized lymph nodes of a short-axis diameter of ≤1 cm on a transverse CT scan image is used to differentiate benign from malignant lymph nodes (8). These results show that nodal size criterion alone is insufficient for the accurate detection of nodal metastases because metastases to normal-sized lymph nodes are missed and enlarged lymph nodes can be reactive or hyperplastic in aetiology. Recent studies suggest that evaluation of nodal morphology and CT enhancement pattern can improve the accuracy of CT for the detection of nodal metastases in lung cancer (9,10). With regards to distant metastases, CT is inferior to PET/CT for detection of extrapulmonary metastases with an accuracy of 88% compared to 97% with PET/CT (11). To our knowledge, there has been no study that examined the accuracy of CT for lung cancer staging using CT scanners with more than 16-detector rows.
Post-treatment evaluation and surveillance
There is currently no consensus on the optimal follow-up and surveillance programme for patients with proven lung cancers. CT has been recommended for the routine evaluation and surveillance of patients who have undergone therapy with curative-intent for non-small cell lung cancer (NSCLC), but routine imaging surveillance is not recommended in asymptomatic patients with advanced lung cancer who are not undergoing therapy (12-14). CT evaluation of response to treatment is usually dependent on morphologic changes in tumour and nodes. However, morphology is not a good indicator of early response to treatment and a positive response can be manifested as a delayed reduction in size or paradoxical increase in size (15). CT can be effective for post-treatment surveillance with one study showing CT detected 93% of new lung cancers and 61% of recurrences in a cohort of over 1,000 patients after resection of early-stage NSCLC (16).
New developments
Recent advances in CT technology have allowed investigation of novel methods for the evaluation of lung cancers including nodule volumetry, nodule perfusion analysis, dual-energy applications and computer-aided detection. Quantitative analysis of lung nodules by assessment of a nodule’s volume can be performed using semi-automated or automated segmentation tools that allow assessment of nodule stability or progression over time. The rate of growth of a nodule is a predictor of the likelihood of malignancy, and volumetric analysis can be used to predict tumour response to treatment (17). CT perfusion analysis of nodules may allow better characterisation of the nodule in order to determine likelihood of malignancy as well as earlier determination of treatment response compared to morphologic change in size (18,19). The likelihood of malignancy is considered low when contrast uptake is below 30 Hounsfield units (HU) (20). Dual-energy CT is a technique that allows differentiation of iodine from other materials, such as soft tissue and bone, due to iodine’s stronger photoelectric absorption (21). This method allows visualisation of the degree and pattern of enhancement within a mass following contrast-enhanced CT. One study showed that the degree of enhancement within a pulmonary nodule can be used to differentiate benign from malignant tumours with a sensitivity, specificity and accuracy of 92%, 70% and 82%, respectively (22). In another study, moderate correlation was found between the maximum iodine attenuation and SUVmax in thoracic nodes in patients with NSCLC, but poor correlation in those patients with small cell lung cancer (23). The authors suggest that moderate correlation in NSCLC could be explained by moderate specificity of PET for determination of malignant nodes, and the difference in correlation seen with NSCLC compared to SCLC due to differing tumour biology such as angiogenic ability.
Positron emission tomography
Solitary pulmonary nodule
PET with F-18 deoxyglucose (FDG) is a useful technique for the characterisation of pulmonary nodules to distinguish between benign and malignant lesions. Two meta-analyses showed the sensitivity and specificity of FDG-PET for the diagnosis of malignant pulmonary nodules were about 96% and 80%, respectively (24,25). The significance of a PET-positive result is dependent on the clinical context and the prevalence of granulomatous and infectious disease, which are recognised causes of false positive PET results. False negative results can occur in small (<10 mm) nodules due to partial volume effect or the effect of respiratory blurring, or in some subtypes of lung malignancy with a low intrinsic FDG avidity, such as adenocarcinoma in situ. On a practical level, a PET-positive study often implies that biopsy or intervention is warranted to obtain pathological confirmation (26), while a PET-negative study may allow conservative approach and avoidance of unnecessary invasive procedures (27). There is evidence that the use of FDG-PET is cost-effective in the management of solitary pulmonary nodules (28,29).
Staging
PET is the most accurate imaging modality for the assessment of nodal and distant metastases from lung cancer, which is vital for treatment planning. PET has been found to be more accurate than CT in the staging of mediastinal nodal disease in many clinicopathological studies, including two meta-analyses that showed the sensitivity and specificity of PET was 79-85% and 90-91%, respectively, compared to 60-61% and 77-79% for CT (30,31). The accuracy of nodal assessment is further increased with PET/CT, which has an excellent negative predictive value of 91% in the mediastinal assessment of early-stage disease (32,33). Despite the high accuracy of PET/CT in nodal staging, there remains a significant false positive rate that is more common with larger (>1 cm) nodes, which is often due to reactive or granulomatous nodal disease (34). With the increased availability of minimally invasive mediastinal nodal sampling procedures, such as endobronchial ultrasound and endoscopic ultrasound, it is imperative to obtain pathological confirmation of PET-positive nodes before denying surgery to patients with potentially curable disease (35,36). PET is the imaging modality of choice in the assessment for distant metastases of lung cancer due to its whole body imaging capability and high tumour to background contrast which allows superior detection of both osseous and soft tissue metastases (37-39). There is a significant incidence of unrecognised distant metastatic disease when staging with conventional CT and bone scintigraphy. One study showed distant metastases were only identified with PET in 7.5%, 18% and 24% of stage I, stage II and stage III disease, respectively (40). Up to 20% of patients who are thought to be operable when staged with conventional imaging are found to be inoperable following PET and, therefore, PET is considered essential prior to curative treatment to avoid unnecessary futile surgical intervention (41,42). PET/CT has been shown to be superior to standalone PET or CT in the detection of distant disease mainly due to the ability of PET/CT to obtain anatomical correlation to reduce false positive PET interpretation of physiologic uptake in normal structures (43). A recent meta-analysis showed PET/CT was significantly superior to PET, MR imaging and bone scintigraphy for the detection of bony metastases with a pooled PET/CT sensitivity and specificity of 92% and 98%, respectively (44). Given the high background FDG uptake in the brain, FDG-PET is not the optimal imaging modality for the exclusion of cerebral metastases, which should be evaluated by MR imaging when clinically indicated (45). The availability of both functional and structural information on PET/CT also facilitates the selection of stage critical lesions for biopsy to allow pathological confirmation. The use of PET and PET/CT is cost-effective in the staging of NSCLC (46-48) with a recent randomised clinical trial showing cost savings of 899 Euro per patient and 4,495 Euro per avoided thoracotomy (49). There is also a strong correlation between PET-stage and survival in both surgical and radical radiotherapy candidates which suggests that PET provides prognostically significant information (50,51).
Radiotherapy planning
FDG-PET and PET/CT have been found to have a critical role in patient selection and target volume definition in patients with locoregionally advanced NSCLC considered for curative or radical radiotherapy. Radical radiotherapy is given with curative intent to non-surgical patients with gross locoregional tumour that can be encompassed by high-dose radiation in the absence of distant disease (52). A number of prospective studies investigating the utility of PET in the staging of potential candidates for radical radiotherapy found 25-30% of the patients were unsuitable for radical treatment owing to the presence of more advanced disease that was not shown on conventional imaging (53-55). PET-assisted radiotherapy treatment volume contouring has been found to be more accurate and significantly different from conventional treatment volumes, and a change in radiation volume was found in more than 30% of the patients (53,56,57). Survival benefit has also been shown with PET/CT-assisted radical radiotherapy. In one study, the 4-year survival of stage IIIA patients managed with PET/CT-assisted radical radiotherapy is 32%, which is superior to outcome with CT-assisted radical radiotherapy (58).
Post-treatment evaluation
A prospective study of 73 patients comparing FDG-PET with CT for the assessment of response following radical radiotherapy and chemoradiation of NSCLC showed significantly more complete responders on PET (34 patients) than CT (10 patients). PET response was more predictive of survival duration than CT response, and is the only prognostic factor associated with survival duration on multifactor analysis (59). A more recent paper also reported a high metabolic tumour volume post definitive treatment for NSCLC was an independent poor prognostic factor (60). FDG-PET has also been found to provide prognostically significant response assessment in NSCLC patients undergoing induction chemotherapy. In a prospective study involving 31 patients with stage III unresectable disease, complete response on PET was more accurate than response on CT, and PET showed superior correlation with longer time to progression and overall survival (61). The ability of FDG-PET to provide superior prognostic information has also been reported in the setting of induction chemotherapy prior to surgical resection or chemoradiation, and there may be a role of PET in treatment selection and planning (62,63).
Magnetic resonance imaging
Modern MR techniques have overcome the principal problem of magnetic field inhomogeneities due to the numerous air-soft tissue interfaces when imaging the lung as well as artefacts associated with cardiac and respiratory movement to produce diagnostic images. The utility of MR for the diagnosis, staging, radiotherapy planning and post-treatment evaluation in lung tumours is under-utilised and has been investigated at only a few centres. The sensitivity, specificity, positive predictive value and negative predictive value of MR for the detection of lung carcinoma and non-calcified lesions greater than 5 mm are close to 100% (64). Therefore, MR can potentially be used for lung cancer screening, but to our knowledge, there are no prospective trials investigating the utility of MR for this purpose (65,66). MR imaging with diffusion-weighted imaging (DWI) can be used to predict benignity of pulmonary lesions. One prospective study of 66 patients showed DWI had a sensitivity, specificity and positive predictive value of 95%, 73% and 87%, respectively, for the diagnosis of a malignant lesion (67).
In current clinical practice, MR imaging is primarily used for the assessment of suspected chest wall or mediastinal invasion by lung cancer due to the superior soft tissue contrast resolution of MR. Comparative studies between MR and FDG-PET/CT have shown the two techniques to be equivalent for staging NSCLC (68-70). The strength of MR is in the detection of cerebral and hepatic metastases, while PET/CT is better at nodal staging. A recent prospective study showed MR imaging with DWI was superior to PET/CT for the detection and nodal assessment of NSCLC (71). MR also allows differentiation of viable tumour from necrotic tumour and atelectasis, and is helpful in radiotherapy planning (72). Post-treatment tumour response has been investigated using MR techniques such as magnetization transfer, blood oxygen level dependent MR, and perfusion and diffusion imaging. Dynamic contrast-enhanced perfusion allows assessment of tumour angiogenesis, which has the potential to predict chemotherapy response in NSCLC patients, but its use is unproven for monitoring of anti-angiogenic therapy (73). MR perfusion can also be used for prediction of postoperative lung function (74). With regards to DWI, the apparent diffusion coefficient (ADC) measures the magnitude of diffusion of water molecules within tissue. Cell swelling or shrinkage is reflected in changes in ADC values. In general, an initial decrease in tumour ADC measurement performed within 30 days of treatment (Figure 1), and an increase in tumour ADC measurement 30 days following treatment were found to be predictive of a positive outcome, and our own unpublished data support similar findings (75,76).
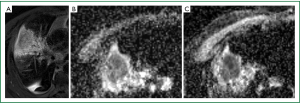
Image-guided percutaneous interventions
Image-guided percutaneous biopsy can be performed for confirmation of diagnosis and treatment planning. In non-surgical candidates, image-guided percutaneous therapy can be performed with curative-intent or for palliation, and these techniques include cryoablation, radiofrequency ablation and microwave ablation.
Percutaneous biopsy
Percutaneous needle core biopsy is a minimally invasive procedure that can be used to confirm the diagnosis of lung malignancy. The most common complications are pneumothorax and bleeding. A higher risk of pneumothorax has been reported to occur with biopsy of smaller lesions and deeper lesions. Biopsy of lesions less than 2 cm in size is associated with an 11 times greater risk of pneumothorax than lesions greater than 4 cm, and this may be explained by the prolonged procedure time required to successfully biopsy smaller lesions (77). This study also showed that the risk of a pneumothorax is negligible for lesions abutting the pleura because the needle does not need to cross aerated lung, but there is a seven-fold increase in the rate of pneumothorax for biopsy of lesions less than 2 cm from pleura and a four-fold rate for lesions greater than 2 cm (77). Hence, the authors advocated a longer oblique needle path for biopsy of sub-pleural nodules to minimise pneumothorax risk, but a different study suggested that a smaller needle-to-pleura angle increases the risk of a pneumothorax (78). Other potential factors for the higher risk of a pneumothorax when lesions less than 2 cm from the pleura are biopsied include multiple punctures and difficulty anchoring a heavy hub cutting needle. A higher risk of pneumothorax following biopsy in patients with obstructive pulmonary disease has been reported in some studies (78,79), but other studies did not find this association (77,80,81). Factors that were not associated with an increased risk of pneumothorax include biopsy of cavitary lesions, biopsy needle size and patient positioning following biopsy (77). Bleeding is the second most common complication of percutaneous lung biopsy, and the two main predisposing factors were lesion size and distance of lesion from the pleural surface. A six-fold increase in bleeding was shown for lesions less than 2 cm in size compared to those greater than 4 cm in size, and lesions greater than 2 cm from the pleura have a ten-fold bleeding risk compared to those abutting the pleural surface (77,82). The presence of a pleural effusion on the side of the biopsy was associated with a decreased risk of bleeding and was found to be an independent risk factor for bleeding following biopsy (77).
Cryoablation
Cryoablation is a percutaneous minimally invasive technique used for the treatment of lung tumours in non-surgical candidates. Cryoablation causes coagulative necrosis of tumour cells and its vasculature. During cryoablation, a 2 to 3 cm rim of normal tissue surrounding the lesion should be ablated to achieve a margin of safety. The cryoablation probe is introduced into the tumour and cooled to –40 °C for about 10 minutes, then thawed for 8 minutes and then cooled once again for a further 10 minutes. Ice formation around the probe disrupts cell membrane function and enzymes, and creates a relative hypertonic extracellular environment causing intracellular dehydration by osmosis. The rapid return of water into the cell during the thawing process causes cell lysis. Direct damage to small (<3 mm) vessel walls and vessel stasis supplying the tumour may also play a role in tumour destruction (83). There is limited long-term outcome data for lung tumours treated with cryoablation. A Japanese series of 20 patients with 35 treated lesions followed for a median of 28 months showed a local recurrence rate of 20% (84). Another study investigated patients with stage I NSCLC who were unsuitable for standard surgical resection, and showed a 3-year survival rate of 77%, 88% and 87% when treated with cryoablation, radiofrequency ablation (RFA) or sublobar resection, respectively (85). Although cryoablation is comparable to other ablative techniques for the management of non-surgical candidates with lung cancer, longer term follow-up data are required to determine its role in the management of lung cancers.
Radiofrequency ablation
RFA is a technique that involves the placement of an electrode into tissue to cause focal destruction with thermal energy, which is generated by friction secondary to oscillating tissue ions that occur when an alternating electric current in the frequency of 460-500 kHz (radio waves) is applied. Heating tissue to 50 °C for at least 5 minutes causes cells to undergo coagulative necrosis. Therapeutic RFA aims to heat tissues to 60-100 °C, which leads to near instant cell death through protein denaturation (86). Pulmonary lesions are ideal for RFA because air in lung parenchyma surrounding the lesion provides thermal insulation to allow concentration of the applied radiofrequency (RF) energy within the lesion. Non-surgical candidates or patients who refuse surgery are potential candidates for RFA and the decision to treat with RFA should ideally be made in consultation with the interdisciplinary pulmonary tumour board. The most suitable lesions for RFA are less than 3 cm in size, and patients with stage I NSCLC are ideal candidates. Reported long-term survival rates after RFA of stage I NSCLC at 1, 2, 3, 4 and 5 years are 78%, 57%, 36%, 27% and 27%, respectively (87). RFA in combination with conventional radiotherapy has been used to treat inoperable lung tumours because hypoxic cells in the centre of tumour respond poorly to radiotherapy alone. A study showed cumulative survival rates of 50% and 39% at 2 and 5 years, respectively, following combined RFA and conventional radiotherapy for stage I NSCLC (88), and dual therapy achieves better local tumour control and patient survival compared to radiotherapy alone (89). Furthermore, RFA can be used to treat small slow growing pulmonary metastases (90) and to palliate patients with larger tumours that cause chest pain, dyspnoea, cough or haemoptysis (87). RFA of larger tumours often requires multiple overlapping ablations to ensure satisfactory tumour coverage, and recurrence rates tend to be higher in larger tumours than with smaller tumours (87,89).
Microwave ablation
Microwave ablation (MWA) represents the most recent addition to the growing armamentarium of minimally invasive thermal ablation therapies for the nonsurgical treatment of lung malignancies (91). Microwaves are electromagnetic waves with a frequency range that extends from 300 MHz to 300 GHz. However, microwave generators for clinical use operate at frequencies of 915 MHz or 2.45 GHz (92). Microwaves agitate water molecules, which are small electric dipoles, in the target tissue, and they spin between 2 and 5 billion times per second in an attempt to follow the rapidly alternating electric field (92,93). This leads to heat generation through friction. Conduction and convection allow further tissue heating beyond the directly agitated water molecules (94). Temperatures thus generated, usually in excess of 100 °C, lead to almost instantaneous irreversible cell damage. The centrifugally growing coagulation necrosis around the active tip of the microwave antenna is spherically shaped. It should ideally encompass the target tumour and a circumferentially surrounding safety margin (Figure 2). This safety margin, ideally at least 6 mm in thickness, is necessary to destroy tumour cell nests and satellite foci in the immediate periphery of the tumour not perceivable on cross-sectional imaging. A smaller safety margin carries a higher risk for local recurrence (95).
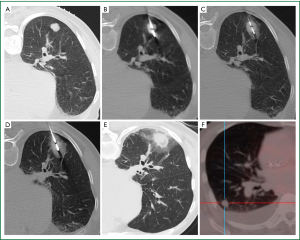
Advantages of MWA over RFA
There are several advantages of microwave over RF energy. RF heating requires an electrical conduction path and is, therefore, less effective in areas of low electrical conductivity and high baseline impedance, such as lung parenchyma. This results in heating of the target tissue only immediately adjacent to the RF electrode (96,97). Microwaves are capable of propagating through many types of tissue and effectively heating them, even those with low electrical conductivity, high impedance or low thermal conductivity (98). Unlike RF and laser, microwaves can penetrate through the charred or desiccated tissues that build up around all hyperthermic ablation applicators which result in limited power delivery for non-microwave energy systems (99). Multiple microwave antennae can be simultaneously powered to maximise the ablation volume when placed in close proximity to each other, or when widely spaced, to simultaneously ablate several tumours, such as multiple pulmonary metastases (95). Larger and more homogeneous ablation volumes can be achieved with MWA because the rapid heating with MWA results in less susceptibility to heat sink effect (92). Further advantages of MWA over RFA include no requirement for grounding pads which avoids potential pad site burns, and implanted cardiac devices are less prone to malfunction (100,101).
Detailed histopathological assessment of microwave ablated lesions has confirmed the concentric layered ablation zones post RFA described by Clasen et al. (102). The central inner necrosis is surrounded by an intermediate zone of equally irreversibly destroyed tissue corresponding to the safety margin strived for. The outer zone of ground glass opacity encompasses a haemorrhagic ring, which in turn is surrounded by oedema and a lymphocytic infiltrate (103). In these outermost layers, there was only partial thermal cell destruction seen with RFA (102). Vital histochemical nicotinamide adenine dinucleotide staining of resected lung tumours which have undergone intra-operative MWA immediately prior to resection confirmed cellular death (because of a lack of mitochondrial enzymatic activity) in a much larger ablation zone (104). No viable cells could be detected within five of the six ablation zones; uniform cellular death was shown to extend through sharp well-demarcated transition zones separating viable and nonviable ablated cells (104).
Patient selection and method
Patients selected for MWA are usually deemed medically inoperable. Exclusion criteria for MWA include uncontrolled primary tumour, radiologic evidence of lymph node metastases, extrathoracic spread, infiltration of the chest wall, mediastinal structures, main bronchi or main pulmonary arteries, sepsis and irreversible coagulopathy (94,105). Patients who have undergone prior surgery (including pneumonectomy), chemotherapy or radiotherapy are usually not excluded. Some patients may have resectable tumours, but have declined surgery. The patient is positioned in the CT scanner that allows the shortest and safest access route to the tumour. Crossing of fissures should be avoided whenever possible. The skin is prepped and draped as usual, and local anaesthetic is infiltrated along the needle tract. A short cut into the skin allows for a smooth passage of the antenna. Under visual guidance, preferably CT fluoroscopy, the antenna is advanced in a stepwise manner into the tumour. Calcified pleural plaques and cartilage should be avoided because the fragile microwave antenna is at risk of fracture if forced through rigid tissue (106). Conscious sedation or general anaesthesia can be used with no difference in complications and outcome between the two modalities (107). Single ablation duration depends on tumour size, location and power capacity of the generator, but usually does not exceed 20 minutes. It is advisable to perform a limited CT scan during the ablation cycle to identify any displacement of the antenna from its original position or early complications. Only a few centres routinely administer prophylactic antibiotics for the procedure (108). Immediate post-procedural recovery includes continuous monitoring for pulse and oxygen saturation, and blood pressure measurements taken every 15-20 minutes. Other observations are performed as per the hospital’s policy. A baseline chest radiograph is performed 3 to 4 hours following the ablation. In most centres, patients are hospitalised after the procedure and discharged the following day provided no complications have occurred. In principle, the procedure can be performed on an outpatient basis, but it is recommended to observe them overnight because of the potential for delayed complications.
Complications
Complications resulting from ablation procedures should be classified in accordance with the Common Terminology Criteria for Adverse Events (CTCAE) (109). The complication rate from MWA varies and can be expected to be higher in patients who have poor underlying pulmonary reserve. Pneumothorax is the most common immediate complication with a reported incidence of up to 43% (110), but less than one-third of these patients require a chest tube (105,110,111). Post-ablation syndrome, defined as a constellation of productive cough with or without minor haemoptysis, residual soreness in the treated area, and fever occurring several days after ablation, is reported in 2% of cases (105). Small pleural effusions not requiring thoracentesis occur in around a quarter of ablations. Cavitary changes are reported in up to 43% of ablated tumours, 14% of which display air-fluid levels that usually involute spontaneously (105). Infective complications (abscess, pneumonia) are rare (105,110). Chest wall emphysema occurs in approximately 20% of cases (unpublished author’s experience) and is usually concurrent with a pneumothorax (Figure 3). Ablated tumours abutting the visceral pleura result in pleural thickening in over one-third of cases (105), and prolonged pleural retractions occur in a small proportion of these cases. Up to 15% of patients require hospitalisation after MWA primarily due to pneumothoraces (105).
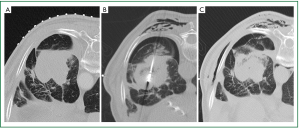
There is scarce comparable long-term outcome data for the effectiveness of MWA in lung cancer owing to the relatively recent addition of MWA to the armamentarium of minimally invasive hyperthermal treatment modalities; different MWA protocols that use different ablation systems operating at different frequencies with different shaft cooling mechanisms, different antennae size and different active tip lengths; and heterogeneous patient population treated, including treatment-naïve primary lung cancer, locally recurrent primary lung cancer following prior therapy, synchronous or metachronous lung cancers, and pulmonary metastases. Two recent publications using the same MWA device and with similar protocols showed promising short to mid-term results. In a homogeneous patient population of early stage NSCLC, the local control rate was 88% and 75% at a median follow-up of 6 months and 1 year, respectively (110,111).
Post-treatment evaluation
CT is the imaging modality of choice for follow-up despite additional radiation exposure. A common post-ablation surveillance protocol is to perform a CT study the day following ablation to assess for complications, and this study can be used as a reference for comparison with subsequent studies, which are performed at 3, 6 and 12 months during the first year, and at 6-monthly intervals thereafter (110). A thin (<5 mm) symmetric circumferential rim of peripheral enhancement is seen up to 6 months following ablation and is considered a sign of benign reactive enhancement. Irregular focal soft-tissue enhancement of >15 HU is, however, considered to be a sign of residual or recurrent disease (105). The initial size of the ablation zone is supposed to be much larger than the treated tumour as it encompasses the surrounding safety margin. Continuous shrinkage thereafter should occur and this usually leaves a small focus of atelectasis (Figure 4) or scar. FDG-PET imaging is considered to be more sensitive for detecting early tumour recurrence. The specificity, however, is low in the early post-ablation period. Performing FDG-PET scans sooner than 6 months following ablation should be discouraged to ensure a low false-positive rate (112,113). In addition to the FDG uptake values, the pattern of FDG uptake is also indicative of ablation success or failure (113). Modified response evaluation criteria in solid tumours (RECIST) criteria, which incorporate both the CT and FDG-PET appearances of the lesion following ablation, are considered the most appropriate tool for follow-up assessment (114).
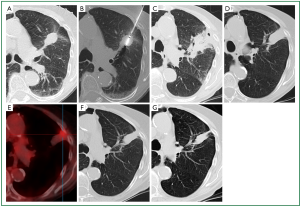
Conclusions
The current challenge for imaging is to exploit the advantages of each imaging modality and integrate them into a clinically useful algorithm. At present, CT and PET/CT are recommended for lung cancer staging, and MR imaging is used for evaluation of suspected T3 and T4 disease. A few recent studies suggest that MR is equivalent to FDG-PET/CT for staging NSCLC. New developments in CT, PET/CT and MR have the potential to provide improved anatomical and functional assessment of lung cancers that result in more individualized and targeted therapy. Cryoablation, RFA and MWA are promising powerful percutaneous techniques for curative-intent therapy or localised palliation of lung cancer, but available short- to mid-term data suggest MWA to be superior to RFA. However, more mid- and long-term data are required to assess for survival and cancer-free outcome following such therapies.
Acknowledgements
Disclosure: The authors declare no conflict of interest.
References
- Ravenel JG, Mohammed TL, Movsas B, et al. ACR Appropriateness Criteria® noninvasive clinical staging of bronchogenic carcinoma. J Thorac Imaging 2010;25:W107-11. [PubMed]
- Willemink MJ, Leiner T, de Jong PA, et al. Iterative reconstruction techniques for computed tomography part 2: initial results in dose reduction and image quality. Eur Radiol 2013;23:1632-42. [PubMed]
- Vardhanabhuti V, Loader RJ, Mitchell GR, et al. Image quality assessment of standard- and low-dose chest CT using filtered back projection, adaptive statistical iterative reconstruction, and novel model-based iterative reconstruction algorithms. AJR Am J Roentgenol 2013;200:545-52. [PubMed]
- Walsh SL, Nair A, Hansell DM. Post-processing applications in thoracic computed tomography. Clin Radiol 2013;68:433-48. [PubMed]
- Stoelben E, Ludwig C. Chest wall resection for lung cancer: indications and techniques. Eur J Cardiothorac Surg 2009;35:450-6. [PubMed]
- Bandi V, Lunn W, Ernst A, et al. Ultrasound vs. CT in detecting chest wall invasion by tumor: a prospective study. Chest 2008;133:881-6. [PubMed]
- Higashino T, Ohno Y, Takenaka D, et al. Thin-section multiplanar reformats from multidetector-row CT data: utility for assessment of regional tumor extent in non-small cell lung cancer. Eur J Radiol 2005;56:48-55. [PubMed]
- Silvestri GA, Gonzalez AV, Jantz MA, et al. Methods for staging non-small cell lung cancer: diagnosis and management of lung cancer, 3rd ed: American College of Chest Physicians evidence-based clinical practice guidelines. Chest 2013;143:e211S-50S.
- Volterrani L, Mazzei MA, Banchi B, et al. MSCT multi-criteria: a novel approach in assessment of mediastinal lymph node metastases in non-small cell lung cancer. Eur J Radiol 2011;79:459-66. [PubMed]
- Takahashi Y, Takashima S, Hakucho T, et al. Diagnosis of regional node metastases in lung cancer with computer-aided 3D measurement of the volume and CT-attenuation values of lymph nodes. Acad Radiol 2013;20:740-5. [PubMed]
- De Wever W, Vankan Y, Stroobants S, et al. Detection of extrapulmonary lesions with integrated PET/CT in the staging of lung cancer. Eur Respir J 2007;29:995-1002. [PubMed]
- Pfister DG, Johnson DH, Azzoli CG, et al. American Society of Clinical Oncology treatment of unresectable non-small-cell lung cancer guideline: update 2003. J Clin Oncol 2004;22:330-53. [PubMed]
- Crinò L, Weder W, van Meerbeeck J, et al. Early stage and locally advanced (non-metastatic) non-small-cell lung cancer: ESMO Clinical Practice Guidelines for diagnosis, treatment and follow-up. Ann Oncol 2010;21:v103-15. [PubMed]
- Colt HG, Murgu SD, Korst RJ, et al. Follow-up and surveillance of the patient with lung cancer after curative-intent therapy: diagnosis and management of lung cancer, 3rd ed: American College of Chest Physicians evidence-based clinical practice guidelines. Chest 2013;143:e437S-54S.
- Junker K, Thomas M, Schulmann K, et al. Tumour regression in non-small-cell lung cancer following neoadjuvant therapy. Histological assessment. J Cancer Res Clin Oncol 1997;123:469-77. [PubMed]
- Lou F, Huang J, Sima CS, et al. Patterns of recurrence and second primary lung cancer in early-stage lung cancer survivors followed with routine computed tomography surveillance. J Thorac Cardiovasc Surg 2013;145:75-81. [PubMed]
- Revel MP, Merlin A, Peyrard S, et al. Software volumetric evaluation of doubling times for differentiating benign versus malignant pulmonary nodules. AJR Am J Roentgenol 2006;187:135-42. [PubMed]
- Fraioli F, Anzidei M, Zaccagna F, et al. Whole-tumor perfusion CT in patients with advanced lung adenocarcinoma treated with conventional and antiangiogenetic chemotherapy: initial experience. Radiology 2011;259:574-82. [PubMed]
- Tacelli N, Santangelo T, Scherpereel A, et al. Perfusion CT allows prediction of therapy response in non-small cell lung cancer treated with conventional and anti-angiogenic chemotherapy. Eur Radiol 2013;23:2127-36. [PubMed]
- Yi CA, Lee KS, Kim EA, et al. Solitary pulmonary nodules: dynamic enhanced multi-detector row CT study and comparison with vascular endothelial growth factor and microvessel density. Radiology 2004;233:191-9. [PubMed]
- Johnson TR, Krauss B, Sedlmair M, et al. Material differentiation by dual energy CT: initial experience. Eur Radiol 2007;17:1510-7. [PubMed]
- Chae EJ, Song JW, Seo JB, et al. Clinical utility of dual-energy CT in the evaluation of solitary pulmonary nodules: initial experience. Radiology 2008;249:671-81. [PubMed]
- Schmid-Bindert G, Henzler T, Chu TQ, et al. Functional imaging of lung cancer using dual energy CT: how does iodine related attenuation correlate with standardized uptake value of 18FDG-PET-CT? Eur Radiol 2012;22:93-103. [PubMed]
- Gould MK, Maclean CC, Kuschner WG, et al. Accuracy of positron emission tomography for diagnosis of pulmonary nodules and mass lesions: a meta-analysis. JAMA 2001;285:914-24. [PubMed]
- Hellwig D, Ukena D, Paulsen F, et al. Meta-analysis of the efficacy of positron emission tomography with F-18-fluorodeoxyglucose in lung tumors. Basis for discussion of the German Consensus Conference on PET in Oncology 2000. Pneumologie 2001;55:367-77. [PubMed]
- Gould MK, Sanders GD, Barnett PG, et al. Cost-effectiveness of alternative management strategies for patients with solitary pulmonary nodules. Ann Intern Med 2003;138:724-35. [PubMed]
- Hashimoto Y, Tsujikawa T, Kondo C, et al. Accuracy of PET for diagnosis of solid pulmonary lesions with 18F-FDG uptake below the standardized uptake value of 2.5. J Nucl Med 2006;47:426-31. [PubMed]
- Keith CJ, Miles KA, Griffiths MR, et al. Solitary pulmonary nodules: accuracy and cost-effectiveness of sodium iodide FDG-PET using Australian data. Eur J Nucl Med Mol Imaging 2002;29:1016-23. [PubMed]
- Lejeune C, Al Zahouri K, Woronoff-Lemsi MC, et al. Use of a decision analysis model to assess the medicoeconomic implications of FDG PET imaging in diagnosing a solitary pulmonary nodule. Eur J Health Econ 2005;6:203-14. [PubMed]
- Dwamena BA, Sonnad SS, Angobaldo JO, et al. Metastases from non-small cell lung cancer: mediastinal staging in the 1990s--meta-analytic comparison of PET and CT. Radiology 1999;213:530-6. [PubMed]
- Gould MK, Kuschner WG, Rydzak CE, et al. Test performance of positron emission tomography and computed tomography for mediastinal staging in patients with non-small-cell lung cancer: a meta-analysis. Ann Intern Med 2003;139:879-92. [PubMed]
- Shim SS, Lee KS, Kim BT, et al. Non-small cell lung cancer: prospective comparison of integrated FDG PET/CT and CT alone for preoperative staging. Radiology 2005;236:1011-9. [PubMed]
- Yang W, Fu Z, Yu J, et al. Value of PET/CT versus enhanced CT for locoregional lymph nodes in non-small cell lung cancer. Lung Cancer 2008;61:35-43. [PubMed]
- Al-Sarraf N, Gately K, Lucey J, et al. Lymph node staging by means of positron emission tomography is less accurate in non-small cell lung cancer patients with enlarged lymph nodes: analysis of 1,145 lymph nodes. Lung Cancer 2008;60:62-8. [PubMed]
- Eloubeidi MA, Cerfolio RJ, Chen VK, et al. Endoscopic ultrasound-guided fine needle aspiration of mediastinal lymph node in patients with suspected lung cancer after positron emission tomography and computed tomography scans. Ann Thorac Surg 2005;79:263-8. [PubMed]
- Herth FJ, Ernst A, Eberhardt R, et al. Endobronchial ultrasound-guided transbronchial needle aspiration of lymph nodes in the radiologically normal mediastinum. Eur Respir J 2006;28:910-4. [PubMed]
- Pieterman RM, van Putten JW, Meuzelaar JJ, et al. Preoperative staging of non-small-cell lung cancer with positron-emission tomography. N Engl J Med 2000;343:254-61. [PubMed]
- Yun M, Kim W, Alnafisi N, et al. 18F-FDG PET in characterizing adrenal lesions detected on CT or MRI. J Nucl Med 2001;42:1795-9. [PubMed]
- Cheran SK, Herndon JE 2nd, Patz EF Jr. Comparison of whole-body FDG-PET to bone scan for detection of bone metastases in patients with a new diagnosis of lung cancer. Lung Cancer 2004;44:317-25. [PubMed]
- MacManus MP, Hicks RJ, Matthews JP, et al. High rate of detection of unsuspected distant metastases by pet in apparent stage III non-small-cell lung cancer: implications for radical radiation therapy. Int J Radiat Oncol Biol Phys 2001;50:287-93. [PubMed]
- van Tinteren H, Hoekstra OS, Smit EF, et al. Effectiveness of positron emission tomography in the preoperative assessment of patients with suspected non-small-cell lung cancer: the PLUS multicentre randomised trial. Lancet 2002;359:1388-93. [PubMed]
- Fischer B, Lassen U, Mortensen J, et al. Preoperative staging of lung cancer with combined PET-CT. N Engl J Med 2009;361:32-9. [PubMed]
- Lardinois D, Weder W, Hany TF, et al. Staging of non-small-cell lung cancer with integrated positron-emission tomography and computed tomography. N Engl J Med 2003;348:2500-7. [PubMed]
- Qu X, Huang X, Yan W, et al. A meta-analysis of 18FDG-PET-CT, 18FDG-PET, MRI and bone scintigraphy for diagnosis of bone metastases in patients with lung cancer. Eur J Radiol 2012;81:1007-15. [PubMed]
- Ettinger DS, Akerley W, Bepler G, et al. Non-small cell lung cancer. J Natl Compr Canc Netw 2010;8:740-801. [PubMed]
- Dietlein M, Weber K, Gandjour A, et al. Cost-effectiveness of FDG-PET for the management of potentially operable non-small cell lung cancer: priority for a PET-based strategy after nodal-negative CT results. Eur J Nucl Med 2000;27:1598-609. [PubMed]
- Farjah F, Flum DR, Ramsey SD, et al. Multi-modality mediastinal staging for lung cancer among medicare beneficiaries. J Thorac Oncol 2009;4:355-63. [PubMed]
- Schreyögg J, Weller J, Stargardt T, et al. Cost-effectiveness of hybrid PET/CT for staging of non-small cell lung cancer. J Nucl Med 2010;51:1668-75. [PubMed]
- Søgaard R, Fischer BM, Mortensen J, et al. Preoperative staging of lung cancer with PET/CT: cost-effectiveness evaluation alongside a randomized controlled trial. Eur J Nucl Med Mol Imaging 2011;38:802-9. [PubMed]
- Dunagan D, Chin R Jr, McCain T, et al. Staging by positron emission tomography predicts survival in patients with non-small cell lung cancer. Chest 2001;119:333-9. [PubMed]
- MacManus MR, Hicks R, Fisher R, et al. FDG-PET-detected extracranial metastasis in patients with non-small cell lung cancer undergoing staging for surgery or radical radiotherapy—survival correlates with metastatic disease burden. Acta Oncol 2003;42:48-54. [PubMed]
- De Ruysscher D, Nestle U, Jeraj R, et al. PET scans in radiotherapy planning of lung cancer. Lung Cancer 2012;75:141-5. [PubMed]
- Mac Manus MP, Hicks RJ, Ball DL, et al. F-18 fluorodeoxyglucose positron emission tomography staging in radical radiotherapy candidates with nonsmall cell lung carcinoma: powerful correlation with survival and high impact on treatment. Cancer 2001;92:886-95. [PubMed]
- Eschmann SM, Friedel G, Paulsen F, et al. FDG PET for staging of advanced non-small cell lung cancer prior to neoadjuvant radio-chemotherapy. Eur J Nucl Med Mol Imaging 2002;29:804-8. [PubMed]
- Kolodziejczyk M, Kepka L, Dziuk M, et al. Impact of [18F]fluorodeoxyglucose PET-CT staging on treatment planning in radiotherapy incorporating elective nodal irradiation for non-small-cell lung cancer: a prospective study. Int J Radiat Oncol Biol Phys 2011;80:1008-14. [PubMed]
- Deniaud-Alexandre E, Touboul E, Lerouge D, et al. Impact of computed tomography and 18F-deoxyglucose coincidence detection emission tomography image fusion for optimization of conformal radiotherapy in non-small-cell lung cancer. Int J Radiat Oncol Biol Phys 2005;63:1432-41. [PubMed]
- Pommier P, Touboul E, Chabaud S, et al. Impact of (18)F-FDG PET on treatment strategy and 3D radiotherapy planning in non-small cell lung cancer: A prospective multicenter study. AJR Am J Roentgenol 2010;195:350-5. [PubMed]
- Mac Manus MP, Everitt S, Bayne M, et al. The use of fused PET/CT images for patient selection and radical radiotherapy target volume definition in patients with non-small cell lung cancer: results of a prospective study with mature survival data. Radiother Oncol 2013;106:292-8. [PubMed]
- Mac Manus MP, Hicks RJ, Matthews JP, et al. Positron emission tomography is superior to computed tomography scanning for response-assessment after radical radiotherapy or chemoradiotherapy in patients with non-small-cell lung cancer. J Clin Oncol 2003;21:1285-92. [PubMed]
- Lee P, Bazan JG, Lavori PW, et al. Metabolic tumor volume is an independent prognostic factor in patients treated definitively for non-small-cell lung cancer. Clin Lung Cancer 2012;13:52-8. [PubMed]
- Decoster L, Schallier D, Everaert H, et al. Complete metabolic tumour response, assessed by 18-fluorodeoxyglucose positron emission tomography (18FDG-PET), after induction chemotherapy predicts a favourable outcome in patients with locally advanced non-small cell lung cancer (NSCLC). Lung Cancer 2008;62:55-61. [PubMed]
- Pöttgen C, Levegrün S, Theegarten D, et al. Value of 18F-fluoro-2-deoxy-D-glucose-positron emission tomography/computed tomography in non-small-cell lung cancer for prediction of pathologic response and times to relapse after neoadjuvant chemoradiotherapy. Clin Cancer Res 2006;12:97-106. [PubMed]
- Eschmann SM, Friedel G, Paulsen F, et al. 18F-FDG PET for assessment of therapy response and preoperative re-evaluation after neoadjuvant radio-chemotherapy in stage III non-small cell lung cancer. Eur J Nucl Med Mol Imaging 2007;34:463-71. [PubMed]
- Biederer J, Hintze C, Fabel M. MRI of pulmonary nodules: technique and diagnostic value. Cancer Imaging 2008;8:125-30. [PubMed]
- National Lung Screening Trial Research Team, Aberle DR, Adams AM, et al. Reduced lung-cancer mortality with low-dose computed tomographic screening. N Engl J Med 2011;365:395-409. [PubMed]
- Wu NY, Cheng HC, Ko JS, et al. Magnetic resonance imaging for lung cancer detection: experience in a population of more than 10,000 healthy individuals. BMC Cancer 2011;11:242. [PubMed]
- Gümüştaş S, Inan N, Akansel G, et al. Differentiation of malignant and benign lung lesions with diffusion-weighted MR imaging. Radiol Oncol 2012;46:106-13. [PubMed]
- Yi CA, Shin KM, Lee KS, et al. Non-small cell lung cancer staging: efficacy comparison of integrated PET/CT versus 3.0-T whole-body MR imaging. Radiology 2008;248:632-42. [PubMed]
- Ohno Y, Koyama H, Onishi Y, et al. Non-small cell lung cancer: whole-body MR examination for M-stage assessment--utility for whole-body diffusion-weighted imaging compared with integrated FDG PET/CT. Radiology 2008;248:643-54. [PubMed]
- Sommer G, Wiese M, Winter L, et al. Preoperative staging of non-small-cell lung cancer: comparison of whole-body diffusion-weighted magnetic resonance imaging and 18F-fluorodeoxyglucose-positron emission tomography/computed tomography. Eur Radiol 2012;22:2859-67. [PubMed]
- Usuda K, Zhao XT, Sagawa M, et al. Diffusion-weighted imaging is superior to positron emission tomography in the detection and nodal assessment of lung cancers. Ann Thorac Surg 2011;91:1689-95. [PubMed]
- Yang RM, Li L, Wei XH, et al. Differentiation of central lung cancer from atelectasis: comparison of diffusion-weighted MRI with PET/CT. PLoS One 2013;8:e60279. [PubMed]
- Ohno Y, Nogami M, Higashino T, et al. Prognostic value of dynamic MR imaging for non-small-cell lung cancer patients after chemoradiotherapy. J Magn Reson Imaging 2005;21:775-83. [PubMed]
- Iwasawa T, Saito K, Ogawa N, et al. Prediction of postoperative pulmonary function using perfusion magnetic resonance imaging of the lung. J Magn Reson Imaging 2002;15:685-92. [PubMed]
- Koh DM, Scurr E, Collins D, et al. Predicting response of colorectal hepatic metastasis: value of pretreatment apparent diffusion coefficients. AJR Am J Roentgenol 2007;188:1001-8. [PubMed]
- Sedlaczek O. Alteration of MR-DWI/ADC before and 24 hours after induction of chemotherapy in patients with NSCLC. Presented at 3rd World Congress of Thoracic Imaging, Seoul, 2013.
- Yeow KM, Su IH, Pan KT, et al. Risk factors of pneumothorax and bleeding: multivariate analysis of 660 CT-guided coaxial cutting needle lung biopsies. Chest 2004;126:748-54. [PubMed]
- Saji H, Nakamura H, Tsuchida T, et al. The incidence and the risk of pneumothorax and chest tube placement after percutaneous CT-guided lung biopsy: the angle of the needle trajectory is a novel predictor. Chest 2002;121:1521-6. [PubMed]
- García-Río F, Pino JM, Casadevall J, et al. Use of spirometry to predict risk of pneumothorax in CT-guided needle biopsy of the lung. J Comput Assist Tomogr 1996;20:20-3. [PubMed]
- Anderson CL, Crespo JC, Lie TH. Risk of pneumothorax not increased by obstructive lung disease in percutaneous needle biopsy. Chest 1994;105:1705-8. [PubMed]
- Moore EH. Technical aspects of needle aspiration lung biopsy: a personal perspective. Radiology 1998;208:303-18. [PubMed]
- Fish GD, Stanley JH, Miller KS, et al. Postbiopsy pneumothorax: estimating the risk by chest radiography and pulmonary function tests. AJR Am J Roentgenol 1988;150:71-4. [PubMed]
- Hoffmann NE, Bischof JC. The cryobiology of cryosurgical injury. Urology 2002;60:40-9. [PubMed]
- Kawamura M, Izumi Y, Tsukada N, et al. Percutaneous cryoablation of small pulmonary malignant tumors under computed tomographic guidance with local anesthesia for nonsurgical candidates. J Thorac Cardiovasc Surg 2006;131:1007-13. [PubMed]
- Zemlyak A, Moore WH, Bilfinger TV. Comparison of survival after sublobar resections and ablative therapies for stage I non-small cell lung cancer. J Am Coll Surg 2010;211:68-72. [PubMed]
- Gazelle GS, Goldberg SN, Solbiati L, et al. Tumor ablation with radio-frequency energy. Radiology 2000;217:633-46. [PubMed]
- Simon CJ, Dupuy DE, DiPetrillo TA, et al. Pulmonary radiofrequency ablation: long-term safety and efficacy in 153 patients. Radiology 2007;243:268-75. [PubMed]
- Dupuy DE, DiPetrillo T, Gandhi S, et al. Radiofrequency ablation followed by conventional radiotherapy for medically inoperable stage I non-small cell lung cancer. Chest 2006;129:738-45. [PubMed]
- Grieco CA, Simon CJ, Mayo-Smith WW, et al. Percutaneous image-guided thermal ablation and radiation therapy: outcomes of combined treatment for 41 patients with inoperable stage I/II non-small-cell lung cancer. J Vasc Interv Radiol 2006;17:1117-24. [PubMed]
- Steinke K, Glenn D, King J, et al. Percutaneous imaging-guided radiofrequency ablation in patients with colorectal pulmonary metastases: 1-year follow-up. Ann Surg Oncol 2004;11:207-12. [PubMed]
- Wasser EJ, Dupuy DE. Microwave ablation in the treatment of primary lung tumors. Semin Respir Crit Care Med 2008;29:384-94. [PubMed]
- Simon CJ, Dupuy DE, Mayo-Smith WW. Microwave ablation: principles and applications. Radiographics 2005;25:S69-83. [PubMed]
- Carrafiello G, Laganà D, Mangini M, et al. Microwave tumors ablation: principles, clinical applications and review of preliminary experiences. Int J Surg 2008;6:S65-9. [PubMed]
- Vogl TJ, Naguib NN, Gruber-Rouh T, et al. Microwave ablation therapy: clinical utility in treatment of pulmonary metastases. Radiology 2011;261:643-51. [PubMed]
- Haemmerich D, Lee FT Jr. Multiple applicator approaches for radiofrequency and microwave ablation. Int J Hyperthermia 2005;21:93-106. [PubMed]
- Schramm W, Yang D, Haemmerich D. Contribution of direct heating, thermal conduction and perfusion during radiofrequency and microwave ablation. Conf Proc IEEE Eng Med Biol Soc 2006;1:5013-6. [PubMed]
- Brace CL. Radiofrequency and microwave ablation of the liver, lung, kidney, and bone: what are the differences? Curr Probl Diagn Radiol 2009;38:135-43. [PubMed]
- Lubner MG, Brace CL, Hinshaw JL, et al. Microwave tumor ablation: mechanism of action, clinical results, and devices. J Vasc Interv Radiol 2010;21:S192-203. [PubMed]
- Skinner MG, Iizuka MN, Kolios MC, et al. A theoretical comparison of energy sources--microwave, ultrasound and laser--for interstitial thermal therapy. Phys Med Biol 1998;43:3535-47. [PubMed]
- Steinke K, Gananadha S, King J, et al. Dispersive pad site burns with modern radiofrequency ablation equipment. Surg Laparosc Endosc Percutan Tech 2003;13:366-71. [PubMed]
- Skonieczki BD, Wells C, Wasser EJ, et al. Radiofrequency and microwave tumor ablation in patients with implanted cardiac devices: is it safe? Eur J Radiol 2011;79:343-6. [PubMed]
- Clasen S, Krober SM, Kosan B, et al. Pathomorphologic evaluation of pulmonary radiofrequency ablation: proof of cell death is characterized by DNA fragmentation and apoptotic bodies. Cancer 2008;113:3121-9. [PubMed]
- Crocetti L, Bozzi E, Faviana P, et al. Thermal ablation of lung tissue: in vivo experimental comparison of microwave and radiofrequency. Cardiovasc Intervent Radiol 2010;33:818-27. [PubMed]
- Wolf FJ, Aswad B, Ng T, et al. Intraoperative microwave ablation of pulmonary malignancies with tumor permittivity feedback control: ablation and resection study in 10 consecutive patients. Radiology 2012;262:353-60. [PubMed]
- Wolf FJ, Grand DJ, Machan JT, et al. Microwave ablation of lung malignancies: effectiveness, CT findings, and safety in 50 patients. Radiology 2008;247:871-9. [PubMed]
- Danaher LA, Steinke K. Hot tips on hot tips: technical problems with percutaneous insertion of a microwave antenna through rigid tissue. J Med Imaging Radiat Oncol 2013;57:57-60. [PubMed]
- Hoffmann RT, Jakobs TF, Lubienski A, et al. Percutaneous radiofrequency ablation of pulmonary tumors--is there a difference between treatment under general anaesthesia and under conscious sedation? Eur J Radiol 2006;59:168-74. [PubMed]
- Carrafiello G, Mangini M, Fontana F, et al. Complications of microwave and radiofrequency lung ablation: personal experience and review of the literature. Radiol Med 2012;117:201-13. [PubMed]
- Retrieved May 20, 2013. Available online:
- Little MW, Chung D, Boardman P, et al. Microwave ablation of pulmonary malignancies using a novel high-energy antenna system. Cardiovasc Intervent Radiol 2013;36:460-5. [PubMed]
- Liu H, Steinke K. High-powered percutaneous microwave ablation of stage I medically inoperable non-small cell lung cancer: a preliminary study. J Med Imaging Radiat Oncol 2013. [Epub ahead of print].
- Yoo DC, Dupuy DE, Hillman SL, et al. Radiofrequency ablation of medically inoperable stage IA non-small cell lung cancer: are early posttreatment PET findings predictive of treatment outcome? AJR Am J Roentgenol 2011;197:334-40. [PubMed]
- Singnurkar A, Solomon SB, Gönen M, et al. 18F-FDG PET/CT for the prediction and detection of local recurrence after radiofrequency ablation of malignant lung lesions. J Nucl Med 2010;51:1833-40. [PubMed]
- Herrera LJ, Fernando HC, Perry Y, et al. Radiofrequency ablation of pulmonary malignant tumors in nonsurgical candidates. J Thorac Cardiovasc Surg 2003;125:929-37. [PubMed]