Is bronchoscopy an obsolete tool in cystic fibrosis? The role of bronchoscopy in cystic fibrosis and its clinical use
Introduction
Cystic fibrosis (CF) is an autosomal recessive disease causing mutational defects of the CF transmembrane conductance regulator (CFTR) protein leading to multi organ involvement such as bronchiectasis, chronic sinusitis, pancreatic insufficiency, hepatobiliary disease, CF related diabetes, and osteoporosis. This gene is located on the long arm of chromosome 7. The median predicted survival of CF patients is 41.6 years based on the CF patient registry in 2015 (1). The mutation in the CFTR protein causes dysregulation of the chloride movement through the channel resulting in the production of thick, viscous mucus in the regions with the most CFTR expression (2). This buildup of thick mucus causes obstruction and inflammation leading to defective mucociliary clearance, chronic infection, and eventually organ failure (3).
Progressive respiratory disease remains the leading cause of morbidity and mortality in CF (2). The vicious cycle of mucus plugging, vigorous neutrophilic inflammation with the release of interleukin (IL)-8 neutrophil elastase have led to bronchiectasis by causing destruction of the airway tissue (4). With the profound neutrophilic inflammation, there is a release of deoxyribonucleic acid (DNA) that is broken down in the infected mucus increasing the viscosity of the secretions (5). Over time with small and medium airway obstruction and recurrent infections, the lung parenchyma undergoes atelectasis, collapse and pneumonia. The bronchial arterial circulation undergoes dilatation and neovascularity likely due to vascular endothelial growth factors in response to inflammation along with anastomotic changes at the capillary level (6). Several studies have looked into the various microbiological communities in CF with particular focus on certain organisms that are pathognomonic for CF such as Haemophilus influenza, Staphylococcus aureus, Pseudomonas aeruginosa, and Burkholderia cepacia complex (7).
CFTR dysfunction is based on the mutations initiating at the level of protein translation with the loss or incomplete protein expression to conducting defects. The mutations are classified from I to V. Table 1 depicts the type of mutation and examples of mutations in the specific class. Some mutations may have features of more than one class. By understanding the different types of mutations, this helps to understand the disease presentation and design newer therapies specific for each mutation class (2).
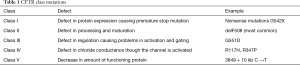
Full table
Management of CF lung disease includes airway clearance with beta agonist bronchodilators, mucolytic agents such as inhaled dornase alpha and hypertonic saline, inhaled antibiotics such as tobramycin, aztreonam, and colistimethate in combination with chest physiotherapy and other percussive and oscillatory devices (3). CFTR modulators improve the function of the dysfunctional CFTR protein based on the mutation. Two such drugs that are used are Ivacaftor specific for gating mutations such as G551D mutation and defect in chloride conductance R117H and Lumacaftor-Ivacaftor for homozygous F508del mutation which is involved with CFTR protein trafficking and folding and channel gating. Studies have shown improvement in lung function and quality of life (3).
Microbiome in CF
The microbiology in the CF lung has a distinctive presentation over a period of time. Most often in the younger population, Staphylococcus aureus and Haemophilus influenza (nontypeable) followed by Pseudomonas aeruginosa. Different strains of Pseudomonas aeruginosa have been identified in several regions of the respiratory system (8,9). Other pathogens that are seen in the advance stage of CF include Burkholderia cepacia complex, Stenotrophomonas maltophilia, and fungi such as Aspergillus fumigatus and Scedosporium apiospermum. Non-tuberculous mycobacteria (NTM) are increasingly prevalent in CF with the most frequently identified species include Mycobacterium avium complex and Mycobacterium abscessus (10).
Sputum cultures obtained quarterly from CF patients may not reveal the extensive microbiota in the airway as there are regional differences and diversity of the microbiota. However sputum cultures remain useful in monitoring most of the pathogens in connection with exacerbations (7). It has been established that the microbiome diversity decreases in advanced lung disease and in older CF patients whereas the microbiome in the younger CF patients have a higher diversity. This is likely due to use of antibiotics due to recurrent pulmonary exacerbations and the progressive decline in FEV1. It has also been suggested that pulmonary exacerbations may be due to spread of infection from other areas of affected airways to the unaffected airways instead of increased burden of the microbiome.
The microbiome identified in the lower airways is distinct from upper airway microbiome (11). Studies have shown various stages of disease in different regions of CF lungs as well as several pathogens distributed disproportionately throughout the lung (11,12). Combination of culture based studies and culture independent molecular studies have shown increased diversity and increased prevalence of anaerobic bacteria. With the increasing identification of uncommon bacteria, its relation to pulmonary exacerbations and decline in FEV1 still remains in question.
Table 2 demonstrates the most common pathogens seen in CF.
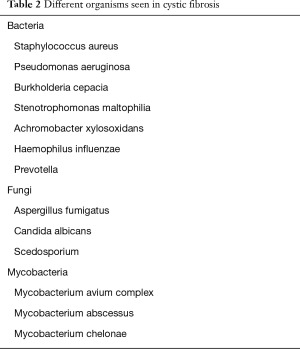
Full table
Utility of bronchoscopy in diagnosis and culture guided therapy in CF
Studies have shown sputum obtained from stable non CF bronchiectasis individuals may be useful as inexpensive and least invasive method to evaluate airway colonization of pathogens. However, not all individuals with bronchiectasis demonstrate the pathogenic microbiota in the lower airways with standard sputum collection. Induced sputum has been shown a preferred technique in evaluating airway colonization of pathogens (13).
In CF, expectorated sputum is useful for identifying pathogens for the management of pulmonary disease especially in individuals who are able to expectorate. In those who are not able to expectorate or have mild disease, sputum induction with nebulized 3 percent saline has been effective in measuring the inflammatory state which is comparable to expectorated samples (14). Bronchoalveolar lavage (BAL) cultures has been shown as a reliable method for in evaluating CF pathogens in comparison to nasopharyngeal cultures as well as identifying anaerobes in the younger population as compared to older population who have a less diverse microbiota (15-17).
Several studies have shown BAL culture from the most damaged lobe represented all the organisms as seen in the rest of the lung and that there is increased diversity in protected brush sampling as compared to BAL (16,17). Sputum cultures have been beneficial in identifying numerous bacteria but it does not reflect the entire diversity of the microbiome in the lower airways as compared to samples obtained by BAL or protected brush sample (15). With the increasing identification of diversity and bacterial burden noted on molecular based studies, there seems to be no increased bacterial burden in pulmonary exacerbations (17).
Henig et al. had looked at the airways of adult CF patients by sampling sputum induction using hypertonic saline, expectorated sputum and BAL with fiberoptic bronchoscope. The results showed that samples obtained by sputum induction had advantages including less quantity of squamous cells, marginally better microbiological results compared to BAL specimens, and similar quantities of inflammatory mediators. Each sampling techniques revealed similar characteristics that was predominant in the airways (18).
Early identification of organisms, especially Pseudomonas aeruginosa, is important in the early eradication of Pseudomonas aeruginosa to prevent chronic infections and to identify other lower respiratory organisms to target appropriate antibiotics which is not easily identified with oral pharyngeal swabs and in those who cannot expectorate. Aaron et al. had looked at the presence of biofilm-forming Pseudomonas aeruginosa and whether the strains differ between sputum collection or protected brush specimens and BAL using fiberoptic bronchoscopy. Both BAL and protected brushings were not superior to sputum specimens for identifying the different strains of Pseudomonas aeruginosa and the differences in the antibiotic susceptibility. It was also shown that the sputum samples showed a better representation of the Pseudomonas aeruginosa strains since the sputum samples represented not only from distal airways from the upper airways as well as compared to BAL samples (19).
Jain et al. reviewed that there was no benefit in bronchoscopy guided therapy compared to standard therapy which included sputum, throat swab, and cough swab on FEV1 and FVC and BMI in children. There were also increased hospital admissions in the BAL directed treatment but with shorter duration of stay. However, there was no significant reduction in the health care cost between the two groups (20). Wainwright et al. have shown that BAL directed therapy provided no benefit in regards to preventing structural changes on radiological imaging or reducing Pseudomonas aeruginosa infection in children. However, there were adverse events related to bronchoscopy with BAL which included clinical deterioration within 24 hours of BAL (4.8%), readmission post procedure (2.3%), contaminated bronchoscope (0.4%), fever greater than 38.5 degree Celsius (7.6%), and transient worsening of cough post procedure (29%) (20).
NTM are increasingly prevalent in CF as the CF population continues to age with the fact that there is an increase in organism load with advancing age. Studies have shown patients with non-tuberculous mycobacteria were older, have mild pulmonary disease, and often with Staphylococcus aureus in the sputum cultures (21-23). Isolating NTM is a challenge and often question whether the presence of NTM pose as a colonizer or cause for infection in the lungs (24). The combination of clinical evidence, radiological findings, and positive cultures including sputum and bronchial washings with non-tuberculous mycobacteria is essential for the diagnosis of non-tuberculous mycobacterial lung disease but it becomes a challenge in CF where identical symptoms have been associated with other pathogens (10,25). Tomashefski et al. had looked at the autopsies of CF patients who had positive sputum cultures for non-tuberculous mycobacteria and demonstrated only a small portion of patients with histological evidence of the disease (26). Tanaka et al. had demonstrated combination of computed tomography findings suggestive of Mycobacterium avium complex (MAC) along with positive bronchial washings for MAC and biopsies revealing granulomas indicated invasive disease rather than colonization in non CF patients (27). It is recommended that in CF patients who have positive cultures for NTM with clinical deterioration including pulmonary function and have excluded other conditions should be evaluated for NTM treatment (28-31). Bronchoscopy with BAL and transbronchial biopsies can be considered if there is a strong indication for evaluating and treating NTM.
Role of bronchoscopy in noninfectious causes in CF
Although there were studies to show the usefulness of bronchoscopy with endobronchial biopsies help to understand airway remodeling and to decelerate the progressive airway damage in children, hemoptysis has been noted more frequently in older CF patients with progressive lung disease associated with Pseudomonas aeruginosa, Burkholderia cepacia, and Staphylococcus aureus along with persistent airway inflammation and angiogenesis of bronchial arteries which are bordering to the airways (32-34).
Endobronchial biopsies have been useful in understanding airway remodeling and inflammatory changes in early CF disease including in those who are asymptomatic (33). Despite the detailed understanding of airway remodeling, sampling has been limited to mostly in the proximal larger airways, smaller areas sampled, and lesser thickness of the airways as supposed to full thickness of the airways (34). Airway inflammation has been demonstrated in infants especially in those with negative cultures for the common bacterial and viral pathogens. Other changes noted include increase in airway smooth muscle cells which could play a role in airway hyper-responsiveness and destruction with fibrotic changes to cartilage which can lead to tracheomalacia and bronchomalacia seen in advanced CF disease (34-38). Reticular basement membrane thickening which has been demonstrated as part of airway remodeling in CF safe guards the airways by limiting proteolytic enzymes released from neutrophilic infiltration to the subepithelial space thus slowing down airway narrowing. As the disease progresses, the reticular basement membranes start to thin causing further inflammation of the airways (33). Vascular changes causing increased blood flow has been seen which is thought to be related to endothelial dysfunction potentiating vasoconstrictor pathways (34,39).
Lobar atelectasis has been reported up to 11% of CF patients (40,41). This is due various factors such as progression of disease, allergic bronchopulmonary aspergillosis, factors that would further alter the viscosity of sputum such as hyperglycemia and hemoptysis. Bronchoscopy with instillation of recombinant human DNase as single or sequential therapies have been reported after failure of standard of treatment including intravenous antibiotics, intermittent positive pressure breathing, and airway clearance therapy (42-46). Figure 1 shows a computed tomography image of patient with CF demonstrating progressive right lung atelectasis.
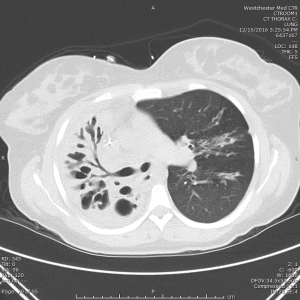
Plastic bronchitis has also been reported in CF. Though it is rare, this is caused by acellular bronchial mucus casts made with mucin. One of the possibilities that cause plastic bronchitis is lymphatic drainage into the bronchus. Mechanical removal may be required with foreign body forceps (47,48).
Tracheomalacia has been associated in children with CF especially in severe disease. The prevalence in children with CF is about 15% as compared to 1:2,100 rate estimated in the general pediatric population. It is more common in adults with CF with prevalence of 69%. Some of the factors that have been associated with tracheomalacia include (I) severe CFTR function leading to severe reduction in FEV1 developing secondary tracheomalacia due to airway infection, (II) abnormalities of the tracheal development predisposing to collapse, (III) combination of congenital tracheomalacia with airway obstruction and infection. Apart from spirometry and computed tomography of the chest, flexible bronchoscopy is done to evaluate for dynamic airway collapse. Positive pressure ventilation can help improve respiratory symptoms by splinting open the airways. Pseudomonas aeruginosa has been linked with early and recurrent infections in children with CF and tracheomalacia. Other medical therapies that have been used include mucolytic therapy and Huff-cough. Placement of biodegradable airway stent to manage localized segments of tracheomalacia has been done (38,49-51).
Bronchoscopy is used for several reasons in CF most often for cultures directed for therapy but is used less often in the advent of similar microbiota seen in induced sputum. BAL has been considered ideal in obtaining cultures especially in those who are unable to expectorate. Now with routine monitoring of surveillance cultures done as outpatient in CF centers and analyzing the microbiota of the CF patients including the use of molecular studies, BAL cultures and protect brush sampling is almost as equivalent to sputum induction with better tolerance and less adverse events in sputum induction. Bronchial washings and transbronchial biopsies are still considered in evaluating NTM disease if there is a strong indication. There is utility in performing bronchoscopy for noninfectious reasons such as lobar collapse and tracheomalacia as this may be underdiagnosed due to the progressive nature of CF.
Acknowledgements
None.
Footnote
Conflicts of Interest: The author has no conflicts of interest to declare.
References
- Cystic fibrosis Foundation. CF Patient Registry 2015 Annual Data Report. Cystic fibrosis Foundation, Bethesda, Maryland.
- Gibson RL, Burns JL, Ramsey BW. Pathophysiology and management of pulmonary infections in cystic fibrosis. Am J Respir Crit Care Med 2003;168:918-51. [Crossref] [PubMed]
- Rafeeq MM, Hussam AS. Cystic fibrosis: current therapeutic targets and future approaches. Journal of Translational Medicine 2017;15:84. [Crossref] [PubMed]
- Morris A, Beck JM, Schloss PD, et al. Lung HIV Microbiome Project. Comparison of the respiratory microbiome in healthy nonsmokers and smokers. Am J Respir Crit Care Med 2013;187:1067-75. [Crossref] [PubMed]
- Shak S, Capon DJ, Hellmiss R, et al. Recombinant human DNase I reduces the viscosity of cystic fibrosis sputum. Proc Natl Acad Sci USA 1990;87:9188-92. [Crossref] [PubMed]
- McDonald DM. Angiogenesis and remodeling of airway vasculature in chronic inflammation. Am J Respir Crit Care Med 2001;164:S39-45. [Crossref] [PubMed]
- Fodor AA, Klem ER, Gilpin DF, et al. The adult cystic fibrosis airway microbiota is stable over time and infection type, and highly resilient to antibiotic treatment of exacerbations. PLoS One 2012;7:e45001. [Crossref] [PubMed]
- Jung A, Kleinau I, Schonian G, et al. Sequential genotyping of Pseudomonas aeruginosa from upper and lower airways of cystic fibrosis patients. Eur Respir J 2002;20:1457-63. [Crossref] [PubMed]
- Armstrong DS, Grimwood K, Carlin JB, et al. Lower airway inflammation in infants and young children with cystic fibrosis. Am J Respir Crit Care Med 1997;156:1197-204. [Crossref] [PubMed]
- Olivier KN, Weber DJ, Wallace RJ Jr, et al. Non-tuberculous mycobacteria. I: multicenter prevalence study in cystic fibrosis. Am J Respir Crit Care Med 2003;167:828-34. [Crossref] [PubMed]
- Gutierrez JP, Grimwood K, Armstrong DS, et al. Interlobar differences in bronchoalveolar lavage fluid from children with cystic fibrosis. Eur Respir J 2001;17:281-6. [Crossref] [PubMed]
- Smith DL, Smith EG, Pitt TL, et al. Regional microbiology of the cystic fibrosis lung: a post-mortem study in adults. J Infect 1998;37:41-3. [Crossref] [PubMed]
- Angrill J, Agusti C, de Celis R, et al. Bacterial colonization in patients with bronchiectasis: microbiological pattern and risk factors. Thorax 2002;57:15-9. [Crossref] [PubMed]
- Sagel SD, Kapsner R, Osberg I, et al. Airway inflammation in children with cystic fibrosis and healthy children assessed by sputum induction. Am J Respir Crit Care Med 2001;164:1425-31. [Crossref] [PubMed]
- Laguna TA, Wagner BD, Williams CB, et al. Airway Microbiota in Bronchoalveolar Lavage Fluid from Clinically Well Infants with Cystic Fibrosis. PLoS One 2016;11:e0167649. [Crossref] [PubMed]
- Hogan DA, Willger SD, Dolben EL, et al. Analysis of lung microbiota in bronchoalveolar lavage, protected brush and sputum samples from subjects with mild-to-moderate cystic fibrosis lung disease. PLoS One 2016;11:e0149998. [Crossref] [PubMed]
- Huang YJ. LiPuma JJ. The Microbiome in Cystic Fibrosis. Clin Chest Med 2016;37:59-67. [Crossref] [PubMed]
- Henig NR, Tonelli MR, Pier MV, et al. Sputum induction as a research tool for sampling the airways of subjects with cystic fibrosis. Thorax 2001;56:306-11. [Crossref] [PubMed]
- Aaron SD, Kottachchi D, Ferris WJ, et al. Sputum versus bronchoscopy for diagnosis of Pseudomonas aeruginosa biofilms in cystic fibrosis. Eur Respir J 2004;24:631-7. [Crossref] [PubMed]
- Jain K, Wainwright C, Smyth AR. Bronchoscopy-guided antimicrobial therapy for cystic fibrosis. Cochrane Database Syst Rev 2013;12:CD009530. [PubMed]
- Hjelte L, Petrini B, Kallenius G, et al. Prospective study of mycobacterial infections in patients with cystic fibrosis. Thorax 1990;45:397-400. [Crossref] [PubMed]
- Torrens JK, Dawkins P, Conway SP, et al. Non-tuberculous mycobacteria in CF. Thorax 1998;53:182-5. [Crossref] [PubMed]
- Olivier KN, Handler AJ, Lee JH, et al. Clinical impact of non-tuberculous mycobacteria on the course of CF lung disease: results of a multicenter, nested cohort study. Pediatr Pulm 2000;30:102-3.
- Cullen AR, Cannon CL, Mark EJ, et al. Mycobacterium abscessus infection in CF. Am J Respir Crit Care Med 2000;161:641-5. [Crossref] [PubMed]
- Griffith DE, Aksamit T, Brown-Elliott BA, et al. An official ATS/IDSA statement: diagnosis, treatment, and prevention of nontuberculous mycobacterial diseases. Am J Respir Crit Care Med 2007;175:367-416. [Crossref] [PubMed]
- Tomashefski JF Jr, Stern RC, Demko CA, et al. Nontuberculous mycobacteria in cystic fibrosis. An autopsy study. Am J Respir Crit Care Med 1996;154:523-8. [Crossref] [PubMed]
- Tanaka E, Amitani R, Nimi A, et al. Yield of computed tomography and bronchoscopy for the diagnosis of Mycobacterium avium complex pulmonary disease. Am J Respir Crit Care Med 1997;155:2041-6. [Crossref] [PubMed]
- Esther CR Jr, Esserman DA, Gilligan P, et al. Chronic Mycobacterium abscessus infection and lung function decline in cystic fibrosis. J Cyst Fibros 2010;9:117-23. [Crossref] [PubMed]
- Olivier KN, Weber DJ, Lee J-H, et al. for the Non-tuberculous Mycobacteria in Cystic fibrosis Study Group. Non-tuberculous mycobacteria. II: Nested-cohort study of impact on cystic fibrosis lung disease. Am J Respir Crit Care Med 2003;167:835-40. [Crossref] [PubMed]
- Martiniano SL, Nick JA. Non-tuberculous mycobacterial infections in cystic fibrosis. Clin Chest Med 2015;36:101-15. [Crossref] [PubMed]
- Ebert DL, Olivier KN. Non-tuberculous mycobacteria in the setting of cystic fibrosis. Clin Chest Med 2002;23:655-63. [Crossref] [PubMed]
- Flume PA, Yankaskas JR, Ebeling M, et al. Massive hemoptysis in cystic fibrosis. Chest 2005;128:729-38. [Crossref] [PubMed]
- Cohen-Cymberknoh M, Kerem E, Ferkol T, et al. Airway inflammation in cystic fibrosis: molecular mechanisms and clinical implications. Thorax 2013;68:1157-62. [Crossref] [PubMed]
- Regamey N, Jeffery PK, Alton E, et al. Airway remodeling and its relationship to inflammation in cystic fibrosis. Thorax 2011;66:624-9. [Crossref] [PubMed]
- Tiddens HA, Koopman LP, Lambert RK, et al. Cartilaginous airway wall dimensions and airway resistance in cystic fibrosis lungs. Eur Respir J 2000;15:735-42. [Crossref] [PubMed]
- Ogrinc G, Kampalath B, Tomashefski JF Jr. Destruction and loss of bronchial cartilage in cystic fibrosis. Hum Pathol 1998;29:65-73. [Crossref] [PubMed]
- Regamey N, Ochs M, Hilliard TN, et al. Increased airway smooth muscle mass in children with asthma, cystic fibrosis, and non-cystic fibrosis bronchiectasis. Am J Respir Crit Care Med 2008;177:837-43. [Crossref] [PubMed]
- McDermott S, Barry SC, Judge EP, et al. Tracheomalacia in adults with cystic fibrosis: determination of prevalence and severity with dynamic cine CT. Radiology 2009;252:577-86. [Crossref] [PubMed]
- Henno P, Maurey C, Danel C, et al. Pulmonary vascular dysfunction in end-stage cystic fibrosis: role of NF-kB and endothelin-1. Eur Respir J 2009;34:1329-37. [Crossref] [PubMed]
- Di Sant'Agnese PA. Bronchial obstruction with lobar atelectasis and emphysema in cystic fibrosis of the pancreas. Pediatrics 1953;12:178-90. [PubMed]
- Stern RC, Boat TF, Orenstein DM, et al. Treatment and prognosis of lobar and segmental atelectasis in cystic fibrosis Am Rev Respir Dis 1978;118:821-6. [PubMed]
- Flight WG, Hildage J, Kevin Webb A. Progressive unilateral lung collapse in cystic fibrosis--a therapeutic challenge. J R Soc Med 2012;105 Suppl 2:S44-9. [Crossref] [PubMed]
- Nagakumar P, Hilliard T. Recurrent lobar atelectasis in a child with cystic fibrosis. J R Soc Med 2012;105 Suppl 2:S50-2. [Crossref] [PubMed]
- Whitaker P, Brownlee K, Lee T, et al. Sequential bronchoscopy in the management of lobar atelectasis secondary to allergic bronchopulmonary aspergillosis. J Bronchology Interv Pulmonol 2011;18:57-60. [Crossref] [PubMed]
- Shah PL, Scott SF, Hodson ME. Lobar atelectasis in cystic fibrosis and treatment with recombinant human DNase I Respir Med 1994;88:313-5. [Crossref] [PubMed]
- Slattery DM, Waltz DA, Denham B, et al. Bronchoscopically administered recombinant human DNase for lobar atelectasis in cystic fibrosis Pediatr Pulmonol 2001;31:383-8. [Crossref] [PubMed]
- Seear M, Hui H, Magee F. Bronchial casts in children: a proposed classification based on nine cases and a review of the literature. Am J Respir Crit Care Med 1997;155:364-70. [Crossref] [PubMed]
- Salamone I, Mondello B, Lucanto MC, et al. Bronchial tree-shaped mucous plug in cystic fibrosis: imaging-guided management. Respirol Case Rep 2017;5:e00214. [Crossref] [PubMed]
- Fischer AJ, Sachinkumar SB, Adam RJ, et al. Tracheomalacia is associated with lower FEV1 and Pseudomonas acquisition in children with CF. Pediatr Pulmonol 2014;49:960-70. [Crossref] [PubMed]
- Boogaard R, Huijsmans SH, Pijnenburg MW, et al. Tracheomalacia and bronchomalacia in children: incidence and patient characteristics. Chest 2005;128:3391-7. [Crossref] [PubMed]
- Zopf DA, Hollister SJ, Nelson ME, et al. Bioresorbable airway splint created with a three-dimensional printer. N Engl J Med 2013;368:2043-5. [Crossref] [PubMed]