Assessment of pulmonary microstructural changes by hyperpolarized 129Xe diffusion-weighted imaging in an elastase-instilled rat model of emphysema
Introduction
Chronic obstructive pulmonary disease (COPD) had moved from the fourth to third most common cause of death worldwide between 1990 and 2010 (1). Emphysema, a primary subcategory of COPD, is characterized as pathology of permanent enlargement of airspaces distal to the terminal bronchioles, accompanied by the destruction of the alveolar walls without obvious fibrosis (2). COPD deteriorates pulmonary function progressively. So far, tobacco smoke, occupational dust and chemicals are the principal risk factors for COPD worldwide.
Conventional methods to diagnose and evaluate emphysema mainly include pulmonary function test (PFT) and computed tomography (CT). PFT is able to provide only gross assessment of the pulmonary status, which is insensitive to the early changes (2). While CT is more sensitive in detecting early localized emphysematous changes. However, the radiation dose limited the repeated measure to make a definitive diagnosis or monitor disease progression in clinical trials (3,4). Proton magnetic resonance imaging (MRI) in human lung imaging is challenging. Some researchers present a technique to quantify the spectral line broadening of the human lung in a single breath-hold, which could be suitable for clinical applications on patients with lung diseases (5). Zhang et al. (6) use dynamic oxygen-enhanced MRI to evaluate lung condition in asthma, the result shows that severe asthmatic group had significantly smaller enhancing fraction. But these imaging methods require additional devices and new data algorithms.
Since lung is an unfriendly environment for proton MRI, some researchers turn their attention to MR gas contrast agents (7,8). Hyperpolarized 3He and 129Xe are suitable gaseous contrast agents for pulmonary MRI, which can provide new methods for visually and noninvasively evaluating pulmonary structure in vivo (8-11). Compared with 129Xe, 3He has higher spin polarization and larger gyromagnetic ratio. These properties make hyperpolarized 3He easy to provide higher MRI signal intensity. Therefore, previous studies mainly focused on hyperpolarized 3He MRI (12-14). However, the limited supply and low nature abundance of 3He make it difficult to permit hyperpolarized 3He MRI of the lung to translate from the research community to a clinical tool (15). In contrast, 129Xe is naturally abundant and its cost is relatively low, and it also can get good image quality with the advanced MRI equipment. Thus, 129Xe is a feasible alternative to 3He as a gas contrast agent for MRI of the lung. More and more researchers shift their focus to 129Xe.
Hyperpolarized 3He diffusion-weighted imaging (DWI) had demonstrated that it is a useful and advanced technique for identifying changes of pulmonary microstructure at the alveolar level by measuring hyperpolarized gas diffusivity in the lung air spaces. While the study of hyperpolarized 129Xe DWI is relatively less. Apparent diffusion coefficient (ADC) is derivative parameter of DWI. ADC value not only can quantitatively describe the relative difficult degree of hyperpolarized gas diffusivity (16-18), but also reflect limitations of the structures of alveolar wall, as well as the walls of bronchioles, alveolar ducts, sacs, and other branches of the airway tree on diffusion of the gas atoms (19,20). In pulmonary emphysema, because of the destruction of alveolar walls, the restrictions to diffusion maybe decreased, and ADC value increased. The purpose of this study was to explore the feasibility of hyperpolarized 129Xe MR DWI in evaluation of pulmonary microstructural changes in experimental rat model of pancreatic porcine elastase (PPE)-induced emphysema.
Methods
Animal model preparation
All the animals were taken care according to the National Institutes of Health Guide and the experiment was approved by the Animal Ethics Committee of the Medical School of Wuhan University. Sixteen male Sprague-Dawley (SD) rats (130–150 g) were randomly separated into two groups: elastase group and control group. In elastase group, orotracheally instilled 200 units/100 g·body weight PPE (Biosharp, Hefei, China) in 0.1 mL saline (n=8); In control group, orotracheal instilled with isodose saline (n=8). After instillation, all rats were then gently shaken to facilitate the distribution of the elastase or saline (21). We had monitored the physiology and behavior of the rats for the next 48 h, such as respiratory, diet and drink, and no adverse reactions were noted. The rats were observed up to 30 days after elastase or saline instillation. On the 30th day, their mean mass was 234 g (elastase group) and 240 g (control group), respectively.
MRI protocol
The natural abundance 129Xe gases were polarized by a homebuilt system via the spin exchange optical pumping (SEOP) method, with rubidium vapor used as the alkali metal (7,8). Then the hyperpolarized 129Xe gas was prepared. 129Xe hyperpolarization level achieved 12%. All MR studies were performed on a 7.0 T micro MR scanner (Bruker BioSpec 70/20 USR, Germany) which was modified with a broadband amplifier to allow operation at the 129Xe resonant frequency. A homebuilt birdcage radio-frequency coil was used for 129Xe imaging.
Before imaging, rats received anesthetizing and intubating, and were passive ventilated by a home-built ventilator, which can ventilate with oxygen or hyperpolarized 129Xe. A 3-way pipe was used to connect the ventilator and intubation. Rats were scanned in a supine position and ventilated with oxygen at a rate of 17 breaths per min with a tidal volume of 3.0 mL. For the ventilation of oxygen, the breath consisted of 400 ms inhalation and 800 ms for exhalation, no breath-hold. During 129Xe imaging, breathing gas was switched from oxygen to hyperpolarized 129Xe. The breath consisted of 500 ms inhalation, 3,000 ms breath-hold, and 1,000 ms exhalation. The ventilator triggered the MRI scanner at the beginning of the breath-hold, preceded by three hyperpolarized 129Xe wash-in breaths to wash-out the oxygen in the lungs thereby maximizing signal. Ventilation image required only one breath-hold for the imaging. For diffusion MRI, there needed two breath-holds, one for b =0 s/mm2, the other for b =5 s/mm2. The inspiratory pressure was monitored by the ventilator continuously and still under 15 cmH2O.
Coronal ventilation images were required by using a spoiled gradient recalled echo pulse sequences with the parameters as follows: echo time/repetition time =5.65/11.26 ms, matrix size =64×64, flip angle =10°, field of view =5×5 cm2, bandwidth =50 kHz, number of slice =1, slice thickness =10 cm. Following acquisition of ventilation images, two different b-value fast images were acquired from the diffusion weighted sequence. Diffusion-weighted MR protocol was obtained based on a gradient-echo pulse sequences with the bipolar gradient waveform in the frequency-encoding direction. The bipolar gradient waveform consisted of the following: ramp up/down time was 0.123 ms, the width of the gradient pulse δ was 2 ms, diffusion time Δ was 2 ms (no separation time between gradient pulses), The b values were set to 0 and 15 s/cm2 (22). Coronal images covering the whole lung were acquired with echo time/repetition time =5.65/11.26 ms, matrix size =64×64, flip angle =10°, field of view =5×5 cm2, bandwidth =50 kHz, number of slice =1, slice thickness =10 cm.
Histology analysis
After imaging, the rats were euthanized, their lungs were extracted and fixed by filling with 10% formalin solution to an airway pressure of 25 cmH2O for 24 h (23). The lungs were processed for conventional histology, sectioned into six slides covering three transverse regions of the lung, and stained with haematoxylin and eosin (HE). Five representative images from each slide were acquired using a microscope (Nikon Eclipse TS 100, Nikon, Japan). The mean linear intercepts (MLIs) for these images were calculated with a 10*10 grid using Image-Pro Plus 6.0 software (Media Cybernetics, Bethesda, MD, USA) to evaluate the presence and severity of emphysematous changes.
Data processing and statistical analysis
The MR data were postprocessed using routines written in MATLAB (MathWorks, Natick, MA, USA). Lungs were segmented from background noise by applying a lower threshold value of signal intensity, and the lung parenchyma was segmented from the major airways to eliminate bias from ADC values in the trachea and primary bronchi by using a relatively higher threshold value. After segmentation, the ADC was calculated by the Eq. [1], as follows:
where S0 (b =0 s/cm2) and S1 (b =15 s/cm2) are the signal intensity values from a pixel without and with diffusion gradient. The signal intensities were fit on a pixel-by-pixel basis to compute maps of ADC. Then the mean ADC value for the whole lung were calculated with segmenting out the background noise and major airways.
A paired t-test implemented the measured ADCs and MLIs between the 2 groups. Spearman’s correlation coefficient tested the correlations between the ADC values and the MLI values from the rats. For all statistical comparisons, the threshold for significance was set to P=0.05. All analyses were performed using GraphPad Prism 6.0 software (GraphPad Software Inc., La Jolla, CA, USA).
Results
The mean mass of the 2 groups was 234 g (elastase group) and 240 g (control group), respectively (P>0.05). Pathological results showed that all model rats (n=8) had emphysema. MR scans of all rats (n=16) were completed successfully. On the hyperpolarized 129Xe ventilation MRI, there existed no distinct ventilation defects in both groups (Figure 1). All rat lungs in both groups showed a homogenous gas distribution, no obvious difference was found.

The ADC maps all showed a homogeneous distribution in control group. In elastase group, the distributions were inhomogeneous, and corresponding ADC histograms exhibited wider (Figure 2). The mean ADC value of elastase group was 0.0313±0.0005 cm2/s, which was significantly higher than that of the control group (0.0288±0.0007 cm2/s, P<0.0001) (Table 1). In both groups, the mean ADC values were all smaller than the free diffusion coefficient of the hyperpolarized 129Xe in the air (24), which indicated that the diffusion of hyperpolarized 129Xe was restricted by the lung microstructure.
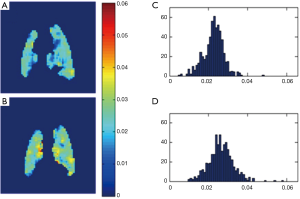
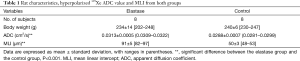
Full table
In elastase group, the mean MLI was 91±5 µm, which was significantly higher than that of the control group (50±3 µm, P<0.0001). There was moderate linear correlation (r=0.724) between the MLI and ADC value among all 16 rats (Figure 3). It showed an obvious trend that higher MLI corresponding to higher diffusivity. HE-stained histology slide of the elastase group demonstrated enlarged airspaces in elastase group. The control group showed homogenous pulmonary parenchyma on lung histology (Figure 4).
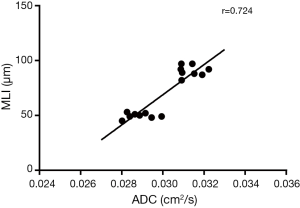
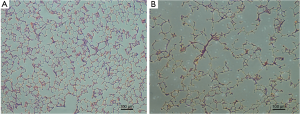
Discussion
Elastase-induced experimental pulmonary emphysematous model is a classic method. In our rat model of emphysema, the histology showed the pulmonary alveolar walls were destroyed, it means emphysema happened and the models were prepared successfully. Combined the modeling time and the metric of MLI (91 µm), the emphysema maybe attributed to mild to moderate degree (25).
Hyperpolarized 129Xe lung ventilation MRI has been shown the sensitivity to disease severity in COPD, cystic fibrosis, asthma and other obstructive lung diseases (20,26,27). This technique can resolve regional impairment in lung ventilation caused by airway obstruction before changes are seen in spirometry and before structural alterations of lung disease are manifested on CT (27). However, in this study, 129Xe ventilation MRI existed no distinct defects of ventilation in both groups, all rats showed a very homogenous hyperpolarized 129Xe gas distribution. It is well known that the elastase-instilled rat model induces regular distribution of airspace enlargement, and does not result in narrowing of terminal bronchioles. In our study, despite the alveolar walls were destroyed, the hyperpolarized 129Xe gas could get into lung airspace freely without any restriction. Hyperpolarized 129Xe ventilation image was only sensitive to the density of the inhaled gas within the lung. Hence, the ventilation images were extremely similar and uniform in both groups in our study, although some minor or subtle ventilation inhomogeneity can be observed in some cases. It suggested that hyperpolarized 129Xe ventilation image maybe not the most sensitive method for the detection of emphysema without airway constriction or blockage.
The ADC value could distinguish the two groups quantitatively. This was consistent with morphological parameter MLI, which was measured by histology. In elastase group, the alveolar dimensions increased with the partial destruction of alveolar walls, thus restriction of gas diffusion reduced. Then the ADC value increased. The increase of ADC value presented the changes of pulmonary microstructure. ADC maps showed a uniform ADC distribution in control group, which implied no emphysema occurred.
In this study, there was moderate linear correlation (r=0.724) between the MLI and their ADC values among all 16 rats. It indicated that the ADC value of hyperpolarized 129Xe could reflect the changes of pulmonary microstructure. This result is related with other studies published earlier (18,28). Mata et al. (28) reported the ADC of 129Xe in a rabbit model showed a strong correlation with MLI (r=0.8). Xu et al. (18) presented a strong correlation between transverse ADC of hyperpolarized 3He and MLI (r=0.9). Boudreau et al. (29) measured the longitudinal (DL) and transverse (DT) diffusion coefficients at different diffusion time, and found a strong correlation (r=0.9) between DT and MLI when diffusion time was 6 ms. Thomen et al. (30) measured the ADC values and MLI in human lungs with COPD and idiopathic pulmonary fibrosis (IPF), showed a moderate correlation (r=0.59) between ADC and MLI. These results further support the feasibility of hyperpolarized 129Xe DWI in the detection of the changes of pulmonary microstructure.
Despite the limitation number of subjects, we still detected a moderate correlation between ADC value and MLI. A greater number of animals would allow us to detect animals at different time points, which might be more useful for evaluating the dynamic changes of pulmonary microstructure. Another limitation, single slice for ventilation and DWI might have been too thick (10 mm), the images were coronal projections, which leads to superposition of parenchyma. This may affect the accuracy of ventilation image and DWI results. In addition, only two b values were used for DWI fit. The result was susceptible to background noise. In future studies, we will use multiple b values to fit the data and perform more models for calculation, such as diffusion kurtosis imaging (DKI) model or Webel model. These mathematical models may reflect more features of emphysema.
Conclusions
Using hyperpolarize 129Xe DWI, we quantitatively observed the differences of pulmonary microstructure in emphysema rat models. We also found a moderate correlation between the ADC value and MLI. 129Xe ADC value is a promising biomarker for the detection of pulmonary emphysema.
Acknowledgements
Thanks to the Wuhan Institute of Physics and Mathematics, Chinese Academy of Sciences for access to scanning facilities.
Funding: The study was supported by Major Program of the National Natural Science Foundation of China (grant No. 81227902) and National Science Foundation of China (grant No. 81171315).
Footnote
Conflicts of Interest: The authors have no conflicts of interest to declare.
Ethical Statement: All the animals were taken care according to the National Institutes of Health Guide and the experiment was approved by the Animal Ethics Committee of the Medical School of Wuhan University.
References
- Burney PG, Patel J, Newson R, et al. Global and regional trends in COPD mortality, 1990-2010. Eur Respir J 2015;45:1239-47. [Crossref] [PubMed]
- Celli BR, MacNee W. ATS/ERS Task Force. Standards for the diagnosis and treatment of patients with COPD: a summary of the ATS/ERS position paper. Eur Respir J 2004;23:932-46. [Crossref] [PubMed]
- Smith-Bindman R, Lipson J, Marcus R, et al. Radiation dose associated with common computed tomography examinations and the associated lifetime attributable risk of cancer. Arch Intern Med 2009;169:2078-86. [Crossref] [PubMed]
- Pearce MS, Salotti JA, Little MP, et al. Radiation exposure from CT scans in childhood and subsequent risk of leukaemia and brain tumours: a retrospective cohort study. Lancet 2012;380:499-505. [Crossref] [PubMed]
- Carinci F, Meyer C, Breuer FA, et al. In vivo imaging of the spectral line broadening of the human lung in a single breathhold. J Magn Reson Imaging 2016;44:745-57. [Crossref] [PubMed]
- Zhang WJ, Niven RM, Young SS, et al. Dynamic oxygen-enhanced magnetic resonance imaging of the lung in asthma--initial experience. Eur J Radiol 2015;84:318-26. [Crossref] [PubMed]
- Albert MS, Cates GD, Driehuys B, et al. Biological magnetic resonance imaging using laser-polarized 129Xe. Nature 1994;370:199-201. [Crossref] [PubMed]
- Albert MS, Balamore D. Development of hyperpolarized noble gas MRI. Nucl Instrum Methods Phys Res A 1998;402:441-53. [Crossref] [PubMed]
- Tustison NJ, Avants BB, Flors L, et al. Ventilation-based segmentation of the lungs using hyperpolarized (3)He MRI. J Magn Reson Imaging 2011;34:831-41. [Crossref] [PubMed]
- He M, Robertson SH, Kaushik SS, et al. Dose and pulse sequence considerations for hyperpolarized (129)Xe ventilation MRI. Magn Reson Imaging 2015;33:877-85. [Crossref] [PubMed]
- Paulin GA, Svenningsen S, Jobse BN, et al. Differences in hyperpolarized (3) He ventilation imaging after 4 years in adults with cystic fibrosis. J Magn Reson Imaging 2015;41:1701-7. [Crossref] [PubMed]
- Kirby M, Kanhere N, Etemad-Rezai R, et al. Hyperpolarized helium-3 magnetic resonance imaging of chronic obstructive pulmonary disease exacerbation. J Magn Reson Imaging 2013;37:1223-7. [Crossref] [PubMed]
- Hamedani H, Shaghaghi H, Kadlecek SJ, et al. Vertical gradients in regional alveolar oxygen tension in supine human lung imaged by hyperpolarized 3He MRI. NMR Biomed 2014;27:1439-50. [Crossref] [PubMed]
- Sukstanskii AL, Quirk JD, Yablonskiy DA. Probing lung microstructure with hyperpolarized 3He gradient echo MRI. NMR Biomed 2014;27:1451-60. [Crossref] [PubMed]
- Möller HE, Chen XJ, Saam B, et al. MRI of the lungs using hyperpolarized noble gases. Magn Reson Med 2002;47:1029-51. [Crossref] [PubMed]
- Chen XJ, Hedlund LW, Möller HE, et al. Detection of emphysema in rat lungs by using magnetic resonance measurements of 3He diffusion. Proc Natl Acad Sci U S A 2000;97:11478-81. [Crossref] [PubMed]
- Wang C, Altes TA, Mugler JP 3rd, et al. Assessment of the lung microstructure in patients with asthma using hyperpolarized 3He diffusion MRI at two time scales: comparison with healthy subjects and patients with COPD. J Magn Reson Imaging 2008;28:80-8. [Crossref] [PubMed]
- Xu X, Boudreau M, Ouriadov A, et al. Mapping of (3) He apparent diffusion coefficient anisotropy at sub-millisecond diffusion times in an elastase-instilled rat model of emphysema. Magn Reson Med 2012;67:1146-53. [Crossref] [PubMed]
- Kaushik SS, Cleveland ZI, Cofer GP, et al. Diffusion-weighted hyperpolarized 129Xe MRI in healthy volunteers and subjects with chronic obstructive pulmonary disease. Magn Reson Med 2011;65:1154-65. [Crossref] [PubMed]
- Kirby M, Svenningsen S, Kanhere N, et al. Pulmonary ventilation visualized using hyperpolarized helium-3 and xenon-129 magnetic resonance imaging: differences in COPD and relationship to emphysema. J Appl Physiol (1985) 2013;114:707-15. [PubMed]
- Rubio ML, Sánchez-Cifuentes MV, Peces-Barba G, et al. Intrapulmonary gas mixing in panacinar- and centriacinar-induced emphysema in rats. Am J Respir Crit Care Med 1998;157:237-45. [Crossref] [PubMed]
- Sukstanskii AL, Yablonskiy DA. Lung morphometry with hyperpolarized 129Xe: theoretical background. Magn Reson Med 2012;67:856-66. [Crossref] [PubMed]
- Sasaki M, Chubachi S, Kameyama N, et al. Evaluation of cigarette smoke-induced emphysema in mice using quantitative micro-computed tomography. Am J Physiol Lung Cell Mol Physiol 2015;308:L1039-45. [Crossref] [PubMed]
- Chen XJ, Möller HE, Chawla MS, et al. Spatially resolved measurements of hyperpolarized gas properties in the lung in vivo. Part I: diffusion coefficient. Magn Reson Med 1999;42:721-8. [Crossref] [PubMed]
- Gonzalez Mangado N, Peces-Barba G, Cabanillas JJ, et al. Effect on single-breath washout and lung function of elastase-induced emphysema in rats. Am Rev Respir Dis 1993;148:735-43. [Crossref] [PubMed]
- Qing K, Mugler JP 3rd, Altes TA, et al. Assessment of lung function in asthma and COPD using hyperpolarized 129Xe chemical shift saturation recovery spectroscopy and dissolved-phase MRI. NMR Biomed 2014;27:1490-501. [Crossref] [PubMed]
- Thomen RP, Walkup LL, Roach DJ, et al. Hyperpolarized 129Xe for investigation of mild cystic fibrosis lung disease in pediatric patients. J Cyst Fibros 2017;16:275-82. [Crossref] [PubMed]
- Mata JF, Altes TA, Cai J, et al. Evaluation of emphysema severity and progression in a rabbit model: comparison of hyperpolarized 3He and 129Xe diffusion MRI with lung morphometry. J Appl Physiol (1985) 2007;102:1273-80. [PubMed]
- Boudreau M, Xu X, Santyr GE. Measurement of 129Xe gas apparent diffusion coefficient anisotropy in an elastase-instilled rat model of emphysema. Magn Reson Med 2013;69:211-20. [Crossref] [PubMed]
- Thomen RP, Quirk JD, Roach D, et al. Direct comparison of 129 Xe diffusion measurements with quantitative histology in human lungs. Magn Reson Med 2017;77:265-72. [Crossref] [PubMed]