Chronic lung allograft dysfunction phenotypes and treatment
Introduction
Although survival has improved spectacularly following lung transplantation, it still limbs behind that after other solid organ transplantations (1). Although improvement in surgical techniques and clinical expertise led to an improved in initial survival, long-term overall outcome remains poor. Graft failure and chronic lung allograft dysfunction (CLAD) are the major culprits for this inferior long-term outcome (2). CLAD has been introduced recently as an overarching term encompassing all forms of chronic (>3 weeks) pulmonary function decline. Next to pulmonary function decline with a known cause (either graft-related, i.e., acute rejection, recurrence of native disease, infection, suture problems; or non-graft related, i.e., obesitas, pleural fluid, diaphragm dysfunction), there is also a large proportion of patients in whom no clear cause can be identified for the decline in pulmonary function which is therefore assumed to be due to chronic rejection. Within this review, we will describe historic and current evidence for CLAD classification and its clinical implications (diagnosis, pathology, radiology, risk factors and mechanisms) with a particular focus on treatment.
History
Historically, the term bronchiolitis obliterans syndrome (BOS) has been universally linked with chronic rejection post-transplant. BOS was defined as a persistent, obstructive decrease in forced expiratory volume in 1 second (FEV1) with at least 20% compared to the mean of the two best post-transplant values, in the absence of other identifiable causes such as acute rejection, infection, suture problems… Further stratification was made according to the relative decrease in FEV1 and consequently a grading system was introduced being BOS1 (FEV1 66–80% of best), BOS2 (FEV 50–65% of best) and BOS3 (<50% of best). BOS was thought to be a functional reflection of obliterative bronchiolitis (OB). OB was considered to be the pathological hallmark of chronic rejection, but can also be found in other conditions such as pulmonary graft versus host disease after hematopoietic stem cell transplantation, auto-immune disorders especially rheumatoid arthritis, inhalation of toxins like sulfur mustard or as a post-infectious complication following childhood viral infection (3). OB is a pathological scarring or filling of the airway lumen with collagenous matrix leading to airflow limitation. The clinical definition of BOS based on serial pulmonary function measurements was deemed necessary given the poor sensitivity and specificity to diagnose OB on transbronchial biopsies (4). However, already in the initial pathological descriptions of explant lungs of patients suffering from chronic rejection some discrepancies were observed, such as the occurrence of a restrictive pulmonary function decline and significant pleural thickening (5,6). Nevertheless, in the following decades, the term BOS was universally utilized when referring to chronic rejection. The first elements to break this dogma came in 2003 when Gerhardt et al. found a proportion of patients with established BOS, who improved their pulmonary function upon azithromycin treatment (7). In some patients, this FEV1 improvement was so pronounced that criteria for BOS were no longer fulfilled. This was confirmed by several other groups (8,9) and led to the first proposition of phenotypes of chronic rejection, leading to a novel phenotype called neutrophilic reversible allograft dysfunction or azithromycin responsive allograft dysfunction (10), which is nowadays considered as a reversible cause of CLAD and therefore is no longer thought to be a manifestation of chronic rejection (11). It was only in 2010 that a restrictive pulmonary function defect came apparent when Woodrow and colleagues defined a group of patients with so called ‘restrictive BOS’ (12). However, this description had no clinical implications (i.e., no survival difference) and therefore it was only in 2011 when Sato et al. identified a restrictive allograft syndrome (RAS) in patients with a decline in total lung capacity and infaust prognosis that general interest was aroused for what was thought to be a novel manifestation of chronic rejection (13). Typically, these patients presented with a restrictive pulmonary function, persistent CT infiltrates and most interestingly inferior survival compared to the obstructive (BOS) patients. Since literature on known causes of CLAD is rare, we will emphasize and contrast BOS to RAS and compare clinical characteristics, with special emphasis on treatment.
BOS
Diagnosis, radiology and pathology
BOS remains the most common phenotype of chronic rejection (65–75%). Typical characteristics include an obstructive pulmonary function defect and air trapping/mosaic attenuation on expiratory CT. Median survival after diagnosis is between 3–5 years. However, even within BOS there is significant heterogeneity: patients with an early (<2 years post-transplant) or a high grade onset (FEV1 decline >35%) have inferior survival compared to patients with late and low grade onset (14). Analysis of explant specimens at redo transplantation has revealed OB in all BOS lungs (15), and the lesions seem to be segmental with 40–60% of the small airways appearing obstructed as of generation 6 on (16), which may explain the obstructive pulmonary function. OB is thought to be the end-result of persistent damage to the bronchial epithelium leading to an excessive inflammatory response, leading to local (myo-) fibroblast recruitment, fibrosis and ultimately complete obliteration of the airway lumen by fibrotic matrix.
Risk factors and mechanisms of BOS
Many risk factors for BOS have been identified such as acute rejection [specifically acute rejections associated with pulmonary function decline (17)], lymphocytic bronchiolitis, infection and colonization with micro-organisms (i.e., Pseudomonas aeruginosa and Aspergillus fumigatus), donor and recipient genetics, primary graft dysfunction, particulate matter and presence of HLA antibodies, or antibodies to self-antigens (18). Especially regarding the latter, progress has been made the last years. De novo development of donor specific antibodies occurs frequently (35–60%) and is independently associated with CLAD (19,20). Similarly, antibodies to self-antigens (like K-α1 tubulin and collagen V) have been demonstrated to increase the risk for subsequent BOS development (21).
Since the mechanisms of BOS remain mostly elusive, novel evidence is accumulating with the use of the mice orthotopic lung transplant model. Depending on the type of mismatch, immunosuppression and the duration of follow-up, lesions compatible with OB can be found in transplanted mice lung. Given the advantage of genetics knockouts in mice and possibility of invasive sampling, this model is an excellent set-up to study underlying mechanisms. For example, it was shown that progressive loss of self-tolerance through epitope spreading promotes airway fibrosis (22). In another experiment, it has been shown that the murine lung allograft fibrosis originates mostly from the donor (23). However, these results cannot be directly extrapolated from mice to men as in humans, 32% of OB lesions are occupied by recipient and not donor fibroblasts (24). Moreover, human OB mostly develops in small airways, yet mice lack small airways, which is an additional problem to overcome. Therefore, experimental research has also focused on in vitro culture of bronchial epithelial cells, which showed that transition of epithelial cells to a mesenchymal phenotype can contribute to the fibroblast accumulation in OB lesions (25). Interestingly, Pseudomonas can significantly aggravate this so called epithelial-mesenchymal transition (EMT), which is important given that colonization with pseudomonas occurs frequently post-transplant and is independently associated with a higher prevalence of BOS (26).
For decades, the search has been ongoing to identify an appropriate marker for BOS. Given the heterogeneous nature of CLAD, it comes to no surprise that at this moment, there are no universally applied biomarkers for BOS diagnosis. Broncho-alveolar lavage (BAL) analysis may provide insights in the lung micro-environment (27). Using BAL, the first important markers for BOS came to light, which included neutrophils and markers of neutrophil activation (CXL-8, MMP-9) (28). Later, however, it became clear that patients with elevated BAL neutrophilia and IL-8 are those who display the best response to treatment with neomacrolides (most commonly azithromycin which was denominated azithromycin responsive allograft dysfunction or neutrophilic responsive allograft syndrome, see above). The same was later seen with BAL IL-17, which has been implicated in BOS. IL-17 is a major pro-inflammatory molecule inducing the release of IL-8, but is also implicated in the response to self-antigens. However, IL-17 staining in the lamina propria later revealed no difference in BOS compared to stable patients (29), while orthotopic lung transplantation in major mismatch mouse strains did not reveal a difference between wildtype and IL-17 knock-out mice (30). In patients with lymphocytic bronchiolitis, who were treated with azithromycin, IL-17 positive cells disappear from the lamina propria and FEV1 increases (31). Nevertheless, these patients may later still develop BOS, without IL-17 involvement. Evidence from other groups nevertheless suggest an important role for IL-17 in CLAD as treatment with an anti-IL-17 antibody or with halofuginone (which reduces IL-17), may attenuate features of chronic rejection in a murine transplant model (32,33).
Overall, none of the historically identified proteins seem to be a good biomarker for BOS development. In fact, a recent BAL cytokine and chemokine analysis, could not detect any molecule that was differentially regulated between stable (non-rejecting) patients and patients with BOS (34). Consequently, some groups have tried to identify blood markers blood for BOS development, but so far none have proven to be very sensitive and specific.
Treatment
The widespread use of the neomacrolides has significantly impacted CLAD incidence and long-term survival. In fact, a randomized placebo controlled prevention trial with azithromycin initiated at hospital discharge following transplantation has shown that patients taking azithromycin demonstrate better pulmonary function, as well as decreased BAL neutrophilia and lower CLAD prevalence (35). A recent post-hoc analysis of this trial revealed that these long-term beneficial effects persisted and that azithromycin was able to significantly postpone the development of CLAD (36). Treatment with macrolides in established CLAD also seems to be an adequate treatment option (37) but given the rarity of randomized controlled trials in this field, we do not know if either prophylactic or targeted treatment is superior. An expert task force concluded that currently available therapies have not shown a significant benefit in preventing or treating BOS, although investigation of possible underlying gastro-oesophageal reflux and a trial with macrolides in BOS is recommended (18). Some other therapies have shown promise in smaller, mostly single-center studies which are briefly discussed below.
Despite attenuation of neutrophils by azithromycin, in a subset of patients elevated airway neutrophilia later can redevelop. These patients usually present with a colonized graft (mostly pseudomonas) and demonstrate inferior survival compared to patients without neutrophilia (38). Interestingly, IL-1α is increased in BAL of those patients indicating that these alarmins might play an important role in the pathophysiology of BOS (39). Macrolide treatment does not seem to affect these patients (40). However extracorporeal photopheresis (ECP), a leukapheresis-based procedure, was beneficial (41), and seems to be mainly an adequate treatment for patient with macrolide resistant airway neutrophilia (42). Part of this beneficial effect can be explained by effects of ECP on reducing inflammatory cytokines, chemokines and donor specific antibodies (43).
Montelukast, a cysteinyl leukotriene inhibitor is another possible treatment for BOS. A case series demonstrated a less pronounced decrease in pulmonary function in patients treated with montelukast compared to never treated patients (44). In a randomized placebo-controlled trial, montelukast was shown to be beneficial especially BOS stage 1 compared to placebo, but in later BOS stages no beneficial effects were seen (Ruttens et al. submitted).
As a last option for BOS, redo transplantation can be considered, amounting to about 5% of the total number of transplantations being performed annually. Although survival is not as good compared to a primary transplantation, for a well-selected group of patients redo transplantation may be the only option to improve outcome and quality of life (45). Given the scarcity of donor organs, this is not an option offered at every transplant center.
Restrictive CLAD (rCLAD)
Diagnosis, radiology and pathology
Besides the most commonly known BOS phenotype, the rCLAD seems to be gaining a lot of interest lately. Diagnostics remains troublesome at the moment. The initial report by Sato et al. used a decline in TLC of at least 10% to diagnose patients suffering from a restrictive pulmonary function defect (13), while Todd et al. used a FVC decrease >20% (46) and Verleden et al. used a combination of TLC and FEV1/FVC (47). The common denominator in all these patients is the presence of persistent pleuroparenchymal infiltrates on CT imaging. Therefore Suhling et al. proposed to use a combination of pulmonary infiltrates on CT and pulmonary function measurements, specifically a TLC decrease >20% (48). In single-lung transplanted recipients, accurate rCLAD diagnosis is more complicated, given the confounding effect of the native lung, but a FVC decrease >20% was also associated with a poor outcome in a multi-center cohort study (49). This poor outcome is also a common denominator in all aforementioned studies: independent of the criteria used to diagnose restriction, outcome was worse in patients with a restrictive (rCLAD) vs. an obstructive (BOS) pulmonary function defect, with a median post-diagnosis survival of 6–18 months in rCLAD compared to 3–5 years in BOS (50). Prevalence of rCLAD is quite similar across different centers with 25–35% of CLAD patients affected (50). It is important to note that this classification is not absolute and that patients can evolve at any time during their post-transplant course from BOS to RAS or vice versa. Most often, patients evolve from an obstructive to a restrictive form of CLAD, however the opposite has also been described (13). Evolution from BOS to rCLAD is very difficult to diagnose, given the underlying severe obstruction, but it does not seem to imply a worse prognosis (51). A representative case with an initial BOS diagnosis is shown in Figure 1, as well as his evolution towards later rCLAD.
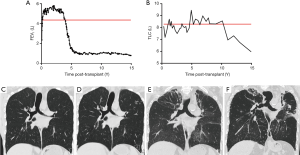
Nowadays diagnostic guidelines for rCLAD are lacking, CT is not implemented as a diagnostic criterium for rCLAD. However, rCLAD typically shows significantly different radiology compared to BOS, as there are signs of (sub)pleural thickening and pleuroparenchymal infiltrates. The land-mark study of Sato et al. showed an apical predominance in a significant subset of patients (13), which was in line with the earlier observation of upper-lobe dominant fibrosis post-lung transplantation (52). However, we recently demonstrated that there are also patients with diffuse or basal-dominated infiltrates on CT and interestingly, these patients had a worse outcome compared to patients with apical dominated fibrosis (51), while the degree of consolidation, ground glass or reticulation did not correlate with survival post diagnosis (53). CT could also be used as alternative tool to diagnose rCLAD, as lungs have significantly lower lung volume compared to baseline, while the volume of lungs in BOS remains stable or even increases (54). This could provide an easy to interpret, add-on tool to diagnose rCLAD when pulmonary function tests are inconclusive. Thorough investigation of rCLAD explant lungs using CT and microCT demonstrated disappearing airways on CT, with OB in 30–40% of the remaining airways. Further, microCT showed a decrease in the number of terminal bronchioles (the last conducting airway before the alveoli). Therefore, this indicates that the airways are also involved in rCLAD, although the proportion of OB lesions was not that high as in pure BOS (55). Next to this airway involvement, the alveoli looked completely different reflecting interstitial and/or alveolar fibrosis.
On pathological examination pleuroparenchymal fibro-elastosis is the most common histological pattern of rCLAD (56). Molecular analysis of this alveolar fibro-elastosis pattern revealed that the initial changes are a non-specific fibrin reaction to a yet unknown injury, which progresses to a failed attempt to resolve this, resulting in manifest fibro-elastosis (57). The Melbourne group first described such a pattern consistent with acute fibrinous and organising pneumonia (AFOP) on transbronchial biopsy, which is also associated with a non-obstructive pulmonary function decline, persistent infiltrates and poor outcome in surviving patients (58). Thus, AFOP and rCLAD are likely to represent two entities (acute-chronic) of the same fibrotic spectrum.
Risk factors and mechanisms
The body of evidence for risk factors specific for rCLAD is not that robust as for BOS, although it seems that many risk factors are similar between both phenotypes. Indeed, acute cellular rejection, lymphocytic bronchiolitis, colonization with Pseudomonas, infection, and BAL neutrophilia were equally important for later BOS and rCLAD (59). Of interest BAL and blood eosinophilia, a cell that is mostly discarded in lung transplantation because of its low relative abundance, shows a strong association with subsequent development of rCLAD (60). Moreover, in patients diagnosed with rCLAD, BAL and blood eosinophilia are also able to dissect those rCLAD patients with the worst prognosis, indicating that eosinophilia could serve as an easy marker for rCLAD development and prognosis following diagnosis (51). Other studies focused on particular (inflammatory) cytokines and chemokines. For example, specific increase in pro-inflammatory alveolar alarmins (61), IL-6 and IP-10 (34) could be important in the pathophysiology of rCLAD. An immunohistochemistry study of rCLAD explant lungs revealed pronounced inflammation, with a significant increase in macrophages, neutrophils, mast-cells, eosinophils, CD8 T-cells and interestingly B-cells. These B-cells were organized in lymphoid follicles, which is a common finding in other chronic respiratory diseases (62). Given this presence of lymphoid follicles, it comes as no surprise that immunoglobulin levels were also increased in rCLAD (63). Therefore, this raises the question to which extent rCLAD overlaps with chronic antibody-mediated rejection (AMR). AMR is an acute or subacute form of graft injury wherein antibodies against donor human leukocyte antigens cause characteristic lung histology (for instance neutrophilic capillaritis) with or without evidence of endothelial C4d staining (64). The presence of HLA antibodies seems to be more associated with rCLAD compared to BOS which is in line with the hypothesis of (at least part) overlap (65).
Of interest is also that in BAL, VEGF levels are decreased in rCLAD patients (34), which is in line with the hypothesis that the capillary network is of importance, which was also demonstrated in a descriptive pathological study (66). In contrast, the lymphatics do not seem to be altered in rCLAD, which is surprising given the predominant distribution (pleural and septal) of fibrosis in rCLAD (67). Despite these interesting observations, more research is needed to elucidate the pathophysiological mechanisms in rCLAD.
Treatment
Similar as in BOS, treatment of rCLAD remains troublesome. The disease course is very unpredictable, given that the disease evolution follows a stepwise pattern of decline: an acute phase characterised by acute lung injury (diffuse alveolar damage, DAD), followed by a resolution stage, during which fibrosis further develops (68). Therefore, patients who at first seem stable can evolve rapidly to a more severe (sometimes even life-threatening) disease stage requiring urgent redo transplantation or death. In that respect, it is important to realize that survival after redo transplantation for rCLAD is inferior compared to BOS, which by itself is already worse compared to survival after primary transplant [3-year survival of 67% in BOS and 33% in rCLAD (69)]. Also, CLAD more frequently redevelops following redo transplantation for rCLAD, again limiting long-term survival. Given these disappointing results, anti-fibrotic treatment may be a good option, based on the positive experience in IPF patients, where it has been shown to slow down the FVC decline (70). Although the experience in treating rCLAD patients is limited at this moment, case reports of successful treatment with pirfenidone (71) and nintedanib (72) described stabilization of the disease, which may be considered a success given the bad prognosis after diagnosis. Nevertheless, no large cohorts have been described so far and therefore more evidence is needed before antifibrotics can be introduced in general clinical practice. ECP therapy does not seem to be able to slow down rCLAD progression and therefore does not seem a viable option (42). Another drug with potential to slow down disease progression is alemtuzumab (Campath-1H), an antagonist of CD52 which is expressed on B-cells, lymphocytes, dendritic cells and monocytes. This drug was found to improve interstitial changes and lung function in four patients who likely had rCLAD (73), while it was also described in successful treatment of persistent acute rejection (74). Another approach of treating rCLAD might be trying to decrease or erase HLA antibodies by using plasmapheresis, intravenous immunoglobulins and rituximab, which has shown to be partly successful in at least reducing the antibody titre (75). However, true efficacy in treating or stabilizing rCLAD remains unknown. Therefore, at present, there are little effective therapeutic options for rCLAD. Hopefully, a better understanding of the pathophysiological mechanisms will lead to a rapid and efficient therapeutic strategy which is desperately needed given the poor outcome of these patients.
Conclusions
BOS and rCLAD are separate entities within CLAD, with their own clinical, radiological and pathological characteristics (see Figure 2 for illustration). To what degree these syndromes differ is at this moment unknown. Given the overlap in risk factors and the fact that OB lesions are detected in both syndromes, and the possible evolution of one syndrome to another, there is likely at least some degree of overlap between BOS and rCLAD. More importantly, rigorous identification of the different phenotypes is clearly needed for both clinical and scientific purposes. Further advance in this field is limited by the absence of uniform diagnostic criteria for rCLAD, which makes the design of multicentre studies nearly impossible. Yet, given the rather low incidence of rCLAD in individual centers, monocentric studies are currently hampered by the number of patients that can be included. Only by doing so, we can adequately power and design clinical trials which are desperately needed given the disappointing outcome after lung transplantation compared to other solid organ transplantations. These different phenotypes are nonetheless an indication that the future will probably lie in individualized therapy, needed to further improve survival.
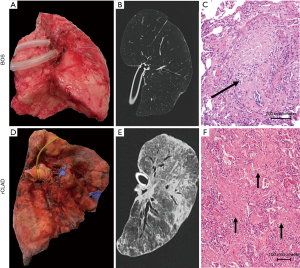
Acknowledgements
Funding: SE Verleden is sponsored by grants from the FWO (FWO12G8715N and 1515816N). R Vos is supported by UZ Leuven (STG15/023). BM Vanaudenaerde is sponsored by the KU Leuven (C24/15/030).
Footnote
Conflicts of Interest: The authors have no conflicts of interest to declare.
References
- Opelz G, Döhler B, Ruhenstroth A, et al. The collaborative transplant study registry. Transplant Rev (Orlando) 2013;27:43-5. [Crossref] [PubMed]
- Yusen RD, Edwards LB, Dipchand AI, et al. The Registry of the International Society for Heart and Lung Transplantation: Thirty-third Adult Lung and Heart-Lung Transplant Report-2016; Focus Theme: Primary Diagnostic Indications for Transplant. J Heart Lung Transplant 2016;35:1170-84. [Crossref] [PubMed]
- Barker AF, Bergeron A, Rom WN, et al. Obliterative bronchiolitis. N Engl J Med 2014;370:1820-8. [Crossref] [PubMed]
- Chamberlain D, Maurer J, Chaparro C, et al. Evaluation of transbronchial lung biopsy specimens in the diagnosis of bronchiolitis obliterans after lung transplantation. J Heart Lung Transplant 1994;13:963-71. [PubMed]
- Yousem SA, Burke CM, Billingham ME. Pathologic pulmonary alterations in long-term human heart-lung transplantation. Hum Pathol 1985;16:911-23. [Crossref] [PubMed]
- Burke CM, Theodore J, Dawkins KD, et al. Post-transplant obliterative bronchiolitis and other late lung sequelae in human heart-lung transplantation. Chest 1984;86:824-9. [Crossref] [PubMed]
- Gerhardt SG, McDyer JF, Girgis RE, et al. Maintenance azithromycin therapy for bronchiolitis obliterans syndrome: results of a pilot study. Am J Respir Crit Care Med 2003;168:121-5. [Crossref] [PubMed]
- Verleden GM, Vanaudenaerde BM, Dupont LJ, et al. Azithromycin reduces airway neutrophilia and interleukin-8 in patients with bronchiolitis obliterans syndrome. Am J Respir Crit Care Med 2006;174:566-70. [Crossref] [PubMed]
- Gottlieb J, Szangolies J, Koehnlein T, et al. Long-term azithromycin for bronchiolitis obliterans syndrome after lung transplantation. Transplantation 2008;85:36-41. [Crossref] [PubMed]
- Vanaudenaerde BM, Meyts I, Vos R, et al. A dichotomy in bronchiolitis obliterans syndrome after lung transplantation revealed by azithromycin therapy. Eur Respir J 2008;32:832-43. [Crossref] [PubMed]
- Verleden GM, Raghu G, Meyer KC, et al. A new classification system for chronic lung allograft dysfunction. J Heart Lung Transplant 2014;33:127-33. [Crossref] [PubMed]
- Woodrow JP, Shlobin OA, Barnett SD, et al. Comparison of bronchiolitis obliterans syndrome to other forms of chronic lung allograft dysfunction after lung transplantation. J Heart Lung Transplant 2010;29:1159-64. [Crossref] [PubMed]
- Sato M, Waddell TK, Wagnetz U, et al. Restrictive allograft syndrome (RAS): a novel form of chronic lung allograft dysfunction. J Heart Lung Transplant 2011;30:735-42. [Crossref] [PubMed]
- Finlen Copeland CA, Snyder LD, Zaas DW, et al. Survival after bronchiolitis obliterans syndrome among bilateral lung transplant recipients. Am J Respir Crit Care Med 2010;182:784-9. [Crossref] [PubMed]
- Martinu T, Howell DN, Davis RD, et al. Pathologic correlates of bronchiolitis obliterans syndrome in pulmonary retransplant recipients. Chest 2006;129:1016-23. [Crossref] [PubMed]
- Verleden SE, Vasilescu DM, Willems S, et al. The site and nature of airway obstruction after lung transplantation. Am J Respir Crit Care Med 2014;189:292-300. [Crossref] [PubMed]
- Davis WA, Finlen Copeland CA, Todd JL, et al. Spirometrically significant acute rejection increases the risk for BOS and death after lung transplantation. Am J Transplant 2012;12:745-52. [Crossref] [PubMed]
- Meyer KC, Raghu G, Verleden GM, et al. An international ISHLT/ATS/ERS clinical practice guideline: diagnosis and management of bronchiolitis obliterans syndrome. Eur Respir J 2014;44:1479-503. [Crossref] [PubMed]
- Le Pavec J, Suberbielle C, Lamrani L, et al. De-novo donor-specific anti-HLA antibodies 30 days after lung transplantation are associated with a worse outcome. J Heart Lung Transplant 2016;35:1067-77. [Crossref] [PubMed]
- Tikkanen JM, Singer LG, Kim SJ, et al. De Novo DQ Donor-Specific Antibodies Are Associated with Chronic Lung Allograft Dysfunction after Lung Transplantation. Am J Respir Crit Care Med 2016;194:596-606. [Crossref] [PubMed]
- Angaswamy N, Tiriveedhi V, Sarma NJ, et al. Interplay between immune responses to HLA and non-HLA self-antigens in allograft rejection. Hum Immunol 2013;74:1478-85. [Crossref] [PubMed]
- Subramanian V, Ramachandran S, Banan B, et al. Immune response to tissue-restricted self-antigens induces airway inflammation and fibrosis following murine lung transplantation. Am J Transplant 2014;14:2359-66. [Crossref] [PubMed]
- Mimura T, Walker N, Aoki Y, et al. Local origin of mesenchymal cells in a murine orthotopic lung transplantation model of bronchiolitis obliterans. Am J Pathol 2015;185:1564-74. [Crossref] [PubMed]
- Bröcker V, Länger F, Fellous TG, et al. Fibroblasts of recipient origin contribute to bronchiolitis obliterans in human lung transplants. Am J Respir Crit Care Med 2006;173:1276-82. [Crossref] [PubMed]
- Borthwick LA, Suwara MI, Carnell SC, et al. Pseudomonas aeruginosa induced airway epithelial injury drives fibroblast activation: a mechanism in chronic lung allograft dysfunction. Am J Transplant 2016;16:1751-65. [Crossref] [PubMed]
- Botha P, Archer L, Anderson RL, et al. Pseudomonas aeruginosa colonization of the allograft after lung transplantation and the risk of bronchiolitis obliterans syndrome. Transplantation 2008;85:771-4. [Crossref] [PubMed]
- Kennedy VE, Todd JL, Palmer SM. Bronchoalveolar lavage as a tool to predict, diagnose and understand bronchiolitis obliterans syndrome. Am J Transplant 2013;13:552-61. [Crossref] [PubMed]
- Riise GC, Williams A, Kjellström C, et al. Bronchiolitis obliterans syndrome in lung transplant recipients is associated with increased neutrophil activity and decreased antioxidant status in the lung. Eur Respir J 1998;12:82-8. [Crossref] [PubMed]
- Verleden SE, Vos R, Vandermeulen E, et al. Involvement of interleukin-17 during lymphocytic bronchiolitis in lung transplant patients. J Heart Lung Transplant 2013;32:447-53. [Crossref] [PubMed]
- Yamada Y, Vandermeulen E, Heigl T, et al. The role of recipient derived interleukin-17A in a murine orthotopic lung transplant model of restrictive chronic lung allograft dysfunction. Transpl Immunol 2016;39:10-7. [Crossref] [PubMed]
- Vos R, Verleden SE, Ruttens D, et al. Azithromycin and the treatment of lymphocytic airway inflammation after lung transplantation. Am J Transplant 2014;14:2736-48. [Crossref] [PubMed]
- Oishi H, Martinu T, Sato M, et al. Halofuginone Treatment reduces interleukin-17A and ameliorates features of chronic lung allograft dysfunction in a mouse orthotopic lung transplant model. J Heart Lung Transplant 2016;35:518-27. [Crossref] [PubMed]
- Fan L, Benson HL, Vittal R, et al. Neutralizing IL-17 prevents obliterative bronchiolitis in murine orthotopic lung transplantation. Am J Transplant 2011;11:911-22. [Crossref] [PubMed]
- Verleden SE, Ruttens D, Vos R, et al. Differential cytokine, chemokine and growth factor expression in phenotypes of chronic lung allograft dysfunction. Transplantation 2015;99:86-93. [Crossref] [PubMed]
- Vos R, Vanaudenaerde BM, Verleden SE, et al. A randomised controlled trial of azithromycin to prevent chronic rejection after lung transplantation. Eur Respir J 2011;37:164-72. [Crossref] [PubMed]
- Ruttens D, Verleden SE, Vandermeulen E, et al. Prophylactic Azithromycin Therapy After Lung Transplantation: Post hoc Analysis of a Randomized Controlled Trial. Am J Transplant 2016;16:254-61. [Crossref] [PubMed]
- Corris PA, Ryan VA, Small T, et al. A randomised controlled trial of azithromycin therapy in bronchiolitis obliterans syndrome (BOS) post lung transplantation. Thorax 2015;70:442-50. [Crossref] [PubMed]
- Vandermeulen E, Verleden SE, Ruttens D, et al. BAL neutrophilia in azithromycin-treated lung transplant recipients: Clinical significance. Transpl Immunol 2015;33:37-44. [Crossref] [PubMed]
- Suwara MI, Vanaudenaerde BM, Verleden SE, et al. Mechanistic differences between phenotypes of chronic lung allograft dysfunction after lung transplantation. Transpl Int 2014;27:857-67. [Crossref] [PubMed]
- Bellon H, Vandermeulen E, Mathyssen C, et al. Interleukin-1α induced release of interleukin-8 by human bronchial epithelial cells in vitro: assessing mechanisms and possible treatment options. Transpl Int 2017;30:388-97. [Crossref] [PubMed]
- Benden C, Speich R, Hofbauer GF, et al. Extracorporeal photopheresis after lung transplantation: a 10-year single-center experience. Transplantation 2008;86:1625-7. [Crossref] [PubMed]
- Greer M, Dierich M, De Wall C, et al. Phenotyping established chronic lung allograft dysfunction predicts extracorporeal photopheresis response in lung transplant patients. Am J Transplant 2013;13:911-8. [Crossref] [PubMed]
- Baskaran G, Tiriveedhi V, Ramachandran S, et al. Efficacy of extracorporeal photopheresis in clearance of antibodies to donor-specific and lung-specific antigens in lung transplant recipients. J Heart Lung Transplant 2014;33:950-6. [Crossref] [PubMed]
- Verleden GM, Verleden SE, Vos R, et al. Montelukast for bronchiolitis obliterans syndrome after lung transplantation: a pilot study. Transpl Int 2011;24:651-6. [Crossref] [PubMed]
- Yusen RD, Edwards LB, Kucheryavaya AY, et al. The registry of the International Society for Heart and Lung Transplantation: thirty-first adult lung and heart-lung transplant report--2014; focus theme: retransplantation. J Heart Lung Transplant 2014;33:1009-24. [Crossref] [PubMed]
- Todd JL, Jain R, Pavlisko EN, et al. Impact of forced vital capacity loss on survival after the onset of chronic lung allograft dysfunction. Am J Respir Crit Care Med 2014;189:159-66. [PubMed]
- Verleden GM, Vos R, Verleden SE, et al. Survival determinants in lung transplant patients with chronic allograft dysfunction. Transplantation 2011;92:703-8. [Crossref] [PubMed]
- Suhling H, Dettmer S, Greer M, et al. Phenotyping Chronic Lung Allograft Dysfunction Using Body Plethysmography and Computed Tomography. Am J Transplant 2016;16:3163-70. [Crossref] [PubMed]
- DerHovanessian A, Todd JL, Zhang A, et al. Validation and Refinement of Chronic Lung Allograft Dysfunction Phenotypes in Bilateral and Single Lung Recipients. Ann Am Thorac Soc 2016;13:627-35. [Crossref] [PubMed]
- Verleden SE, Ruttens D, Vandermeulen E, et al. Restrictive chronic lung allograft dysfunction: Where are we now? J Heart Lung Transplant 2015;34:625-30. [Crossref] [PubMed]
- Verleden SE, Ruttens D, Vandermeulen E, et al. Predictors of survival in restrictive chronic lung allograft dysfunction after lung transplantation. J Heart Lung Transplant 2016;35:1078-84. [Crossref] [PubMed]
- Pakhale SS, Hadjiliadis D, Howell DN, et al. Upper lobe fibrosis: a novel manifestation of chronic allograft dysfunction in lung transplantation. J Heart Lung Transplant 2005;24:1260-8. [Crossref] [PubMed]
- Verleden SE, de Jong PA, Ruttens D, et al. Functional and computed tomographic evolution and survival of restrictive allograft syndrome after lung transplantation. J Heart Lung Transplant 2014;33:270-7. [Crossref] [PubMed]
- Saito T, Horie M, Sato M, et al. Low-dose computed tomography volumetry for subtyping chronic lung allograft dysfunction. J Heart Lung Transplant 2016;35:59-66. [Crossref] [PubMed]
- Verleden SE, Vasilescu DM, McDonough JE, et al. Linking clinical phenotypes of chronic lung allograft dysfunction to changes in lung structure. Eur Respir J 2015;46:1430-9. [Crossref] [PubMed]
- Ofek E, Sato M, Saito T, et al. Restrictive allograft syndrome post lung transplantation is characterized by pleuroparenchymal fibroelastosis. Mod Pathol 2013;26:350-6. [Crossref] [PubMed]
- Jonigk D, Izykowski N, Rische J, et al. Molecular Profiling in Lung Biopsies of Human Pulmonary Allografts to Predict Chronic Lung Allograft Dysfunction. Am J Pathol 2015;185:3178-88. [Crossref] [PubMed]
- Paraskeva M, McLean C, Ellis S, et al. Acute fibrinoid organizing pneumonia after lung transplantation. Am J Respir Crit Care Med 2013;187:1360-8. [Crossref] [PubMed]
- Verleden SE, Ruttens D, Vandermeulen E, et al. Bronchiolitis obliterans syndrome and restrictive allograft syndrome: do risk factors differ? Transplantation 2013;95:1167-72. [Crossref] [PubMed]
- Verleden SE, Ruttens D, Vandermeulen E, et al. Elevated bronchoalveolar lavage eosinophilia correlates with poor outcome after lung transplantation. Transplantation 2014;97:83-9. [Crossref] [PubMed]
- Saito T, Liu M, Binnie M, et al. Distinct expression patterns of alveolar “alarmins” in subtypes of chronic lung allograft dysfunction. Am J Transplant 2014;14:1425-32. [Crossref] [PubMed]
- Vandermeulen E, Lammertyn E, Verleden SE, et al. Immunological diversity in phenotypes of chronic lung allograft dysfunction: a comprehensive immunohistochemical analysis. Transpl Int 2017;30:134-43. [Crossref] [PubMed]
- Vandermeulen E, Verleden SE, Bellon H, et al. Humoral immunity in phenotypes of chronic lung allograft dysfunction: A broncho-alveolar lavage fluid analysis. Transpl Immunol 2016;38:27-32. [Crossref] [PubMed]
- Levine DJ, Glanville AR, Aboyoun C, et al. Antibody-mediated rejection of the lung: A consensus report of the International Society for Heart and Lung Transplantation. J Heart Lung Transplant 2016;35:397-406. [Crossref] [PubMed]
- Roux A, Bendib Le Lan I, Holifanjaniaina S, et al. Antibody-Mediated Rejection in Lung Transplantation: Clinical Outcomes and Donor-Specific Antibody Characteristics. Am J Transplant 2016;16:1216-28. [Crossref] [PubMed]
- Montero MA, Osadolor T, Khiroya R, et al. Restrictive allograft syndrome and idiopathic pleuroparenchymal fibroelastosis: do they really have the same histology? Histopathology 2017;70:1107-13. [Crossref] [PubMed]
- Traxler D, Schweiger T, Schwarz S, et al. The Lymphatic Phenotype of Lung Allografts in Patients With Bronchiolitis Obliterans Syndrome and Restrictive Allograft Syndrome. Transplantation 2017;101:310-5. [Crossref] [PubMed]
- Sato M, Hwang DM, Waddell TK, et al. Progression pattern of restrictive allograft syndrome after lung transplantation. J Heart Lung Transplant 2013;32:23-30. [Crossref] [PubMed]
- Verleden SE, Todd JL, Sato M, et al. Impact of CLAD Phenotype on Survival After Lung Retransplantation: A Multicenter Study. Am J Transplant 2015;15:2223-30. [Crossref] [PubMed]
- Noble PW, Albera C, Bradford WZ, et al. Pirfenidone in patients with idiopathic pulmonary fibrosis (CAPACITY): two randomised trials. Lancet 2011;377:1760-9. [Crossref] [PubMed]
- Vos R, Verleden SE, Ruttens D, et al. Pirfenidone: a potential new therapy for restrictive allograft syndrome? Am J Transplant 2013;13:3035-40. [Crossref] [PubMed]
- Suhling H, Bollmann B, Gottlieb J. Nintedanib in restrictive chronic lung allograft dysfunction after lung transplantation. J Heart Lung Transplant 2016;35:939-40. [Crossref] [PubMed]
- Kohno M, Perch M, Andersen E, et al. Treatment of intractable interstitial lung injury with alemtuzumab after lung transplantation. Transplant Proc 2011;43:1868-70. [Crossref] [PubMed]
- Reams BD, Musselwhite LW, Zaas DW, et al. Alemtuzumab in the treatment of refractory acute rejection and bronchiolitis obliterans syndrome after human lung transplantation. Am J Transplant 2007;7:2802-8. [Crossref] [PubMed]
- Hachem RR, Yusen RD, Meyers BF, et al. Anti-human leukocyte antigen antibodies and preemptive antibody-directed therapy after lung transplantation. J Heart Lung Transplant 2010;29:973-80. [Crossref] [PubMed]