Malignant central airway obstruction
Introduction
A significant proportion of lung cancer patients will develop obstruction of the central airways at some point in the course of disease and as many as 40% of lung cancer related deaths are a direct result of loco-regional disease (1). Although most commonly a result of primary lung cancer, malignant central airway obstruction (CAO) can result from any primary or metastatic intrathoracic malignancy. Malignancies adjacent to the airways such as esophageal carcinoma, thyroid cancer and primary mediastinal tumors can cause airway obstruction either by external compression or direct tumor growth into the airways, while extra thoracic cancers can metastasize to the airways with the commonest culprits being breast, colorectal and renal malignancies (2). Although much less common, malignant CAO can also occur as a result of primary airway malignancies (3-5).
Classification of malignant CAO
When bronchoscopic interventions are contemplated for malignant CAO, the lesion characteristics direct the selection of therapeutic modalities. Malignant CAO is classified as extraluminal (extrinsic), endoluminal (intrinsic), or mixed (combined intrinsic and extrinsic) (Figures 1,2). As a general principle, purely extraluminal compression is managed with dilation, if needed, and airway stents which exert an outward radial force to counter any centripetal forces. Purely endoluminal obstruction is primarily managed with modalities that debulk the intraluminal disease. Airway stents may be utilized following debulking in select cases to prevent recurrent tumor in-growth via a barrier effect if needed. Finally, mixed disease with both endoluminal disease and an extrinsic component typically require multimodality therapy with endoluminal debulking, possibly followed by airway stenting.
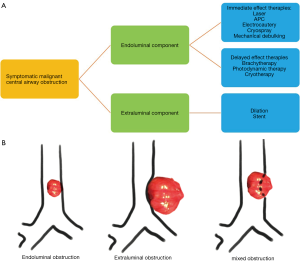
Clinical presentation of malignant CAO
Symptoms of malignant CAO can be mild such as cough and exertional dyspnea, but are often severe resulting in rest dyspnea, hemoptysis, post-obstructive infections, and asphyxiation (6,7). In patients with known intrathoracic malignancies the diagnosis of CAO may be relatively apparent, however, when a cancer diagnosis has not yet been established, patients will often present after receiving multiple courses of treatment for more common causes of dyspnea and wheezing such as asthma and COPD, especially when tumors are slow growing (8). With the increased use of computed tomography (CT) asymptomatic CAO is also occasionally encountered (9-11).
The degree of endoluminal obstruction which will result in symptoms is not entirely clear. Many consider an endoluminal diameter <50% of normal to be indicative of “significant airway obstruction”. A common myth, which has been propagated within the literature without clinical or physiological support, is that exertional symptoms related to tracheal CAO will not develop until the endoluminal diameter is <8 mm and that rest symptoms will not occur until the endoluminal diameter is <5 mm (11,12). Using a fixed arbitrary cut-off to decide if symptoms are attributable to CAO is problematic as multiple factors must be considered beyond simply endoluminal diameter. Although the degree of obstruction is the predominant determinant of flow limitation, as the Hagen–Poiseuille equation explains, flow through a tube depends not only on radius but also on other physiological factors such as length and the pressure differential across the tube which is determined by the patient’s ability to generate negative intrathoracic pressure. Similarly, dyspnea perception depends upon multiple variables. The ability of the patient to generate negative intrathoracic pressure is dependent upon muscle strength (which can be affected by cancer and cachexia), compliance of the chest wall and the elastic properties of the lung which might be altered by co-morbid parenchymal disease. Additionally, other factors such as metabolic demand, acuity of onset of obstruction, degree of post-obstructive atelectasis, severity of underlying pulmonary, cardiac and renal disease, pain and anxiety can all contribute to perceived dyspnea.
Initial work-up
The initial evaluation of a patient with malignant CAO is dictated by the clinical situation and stability of the patient. While thorough pre-treatment evaluation is desirable, when patients present in extremis, urgent intervention is often required and even basic pre-procedural tests such as plain radiographs might be unavailable. In this section, we will review the most common and useful tests for the evaluation of patients with malignant CAO but with the understanding that the potential benefits of tests must be weighed against risks associated with delaying intervention or symptom exacerbation related to the testing itself.
Radiographic evaluation
Chest radiograph
Although routinely obtained, basic chest radiographs rarely provide significant information in the evaluation of CAO and are far less sensitive that CT (13). Plain radiographs can occasionally provide clinical value and are sometimes the only imaging modalities available in situations where patients are unable to lie flat for axial imaging due to orthopnea and hypoxia. Additionally, baseline plain radiographs can provide rapid post-intervention comparison as it is often impractical to obtain serial CT scans. The standard postero-anterior (PA) film is a good tool for lateralizing pathology, however, assessment of the central airways is often limited by mediastinal and bony structures overlying the central airways. The lateral radiograph provides a less obscured evaluation of the trachea and can often detect abnormalities not seen on the frontal film, especially when obstruction occurs from tumors involving the anterior or posterior walls of the airway.
Computer tomography
CT has long been recognized as the imaging modality of choice for patients with CAO and can provide valuable information for procedural planning through relatively accurate estimations of lesion length, degree of airway narrowing and anatomic relationships to structures surrounding the airways (13). The development of thin-section volumetric multi-detector CT and software innovations have been a breakthrough in the imaging of the central airways allowing for improvements in speed of acquisition, spatial resolution and the ability to use post-processing techniques to provide 2-D and 3-D rendering to augment the axial views. Extraluminal 3-D rendering allows for more anatomically relevant evaluation of the airways in respect to surrounding structures, while intraluminal reconstructions allow for a virtual bronchoscopic evaluation. The main advantage of the virtual bronchoscopy is the ability to bypass the obstruction to view the distal airways even with high grade or complete luminal obstruction which precludes the passage of the bronchoscope. Although physicians are sometimes more comfortable with specific viewing planes, all the available CT renderings can provide complementary information to aid in characterizing the obstruction and inform management decisions and it has been shown that simultaneous viewing of axial, multiplanar and 3-D renderings on a single workstation improves precision and accuracy in central airway disease (14). When ordering a chest CT, it is important to communicate the concern for CAO with the radiologist. CAO is a relatively infrequent finding on chest imaging and evaluation of the central airways may be overlooked in the absence of clinical history. In a recent retrospective review of pre-treatment chest CT in 42 patients who eventually underwent therapeutic bronchoscopic interventions for CAO, the radiologist failed to identify CAO in 31% of studies and in the subgroup of patients that did not have CAO reported there was a significant delay in time to therapeutic intervention (15).
When evaluating CAO by CT, it is important to note the maximal degree of obstruction, length of obstruction and luminal diameter of the normal airways, especially when considering stent placement as this knowledge can help determine the appropriate sized stent. When the mainstem bronchi are involved, the contralateral mainstem can be used to estimate the normal diameter. The right mainstem is usually slightly larger than the left but the difference is usually minimal. CT scanning can over-estimate the degree of obstruction when mucus or blood is present and is complementary to bronchoscopic evaluation. Defining the type of obstruction (intrinsic, extrinsic or mixed) should be attempted, but CT can be misleading particularly when trying to assess tumor ingrowth from extrinsic malignancies since mucosal and cartilaginous planes can be difficult to visualize and secretions at the site of obstruction can masquerade as intrinsic disease (Figure 3). Other important considerations include the location of obstruction, craniocaudal length, and relationship to surrounding structures. For tracheal tumors, the distance from the cricoid cartilage to upper edge of tumor and distance from lower edge to carina are important for procedural and surgical planning. In the setting of post-obstructive atelectasis comparison films from previous time points can be extremely useful as decision to intervene is often deferred if distal lung is not considered viable. Although there is no data available that clearly predicts viability of obstructed parenchyma, the most likely indicators of non-viable lung are duration of atelectasis (>4 weeks) and presence of inhomogeneous enhancement indicating necrosis (16,17).
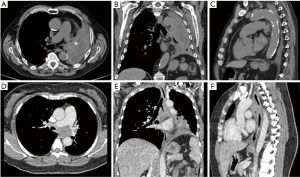
Pulmonary function testing
Spirometry can be a useful tool to assess for airflow limitation from CAO and document post treatment effect (Figure 4). Unlike in peripheral airway disease,, CAO does not typically result in significant reductions in the forced expiratory volume at 1 second (FEV1) or vital capacity (VC) until obstruction is relatively severe (18,19). However, the peak inspiratory (PIF) and peak expiratory (PEF) flow rates are often significantly reduced. In tracheal obstruction, this results in truncation of the inspiratory and expiratory limbs of the flow volume loop while in unilateral mainstem obstruction a biphasic curve may be seen resulting from delayed airflow in the affected lung (19,20). There are several limitations to spirometry as a tool to detect and quantify malignant CAO including poor sensitivity when obstruction in not severe, lack quantifiable data to characterize the severity of obstruction or response to treatment, difficulty in interpretation in the setting of concomitant peripheral obstructive disease, and concerns with safety and reproducibility when performing standard spirometry in patients with significant respiratory impairment related to CAO (21-23). Although beyond the scope of this review, multiple quantitative spirometric criteria have been proposed to detect the disproportionate reduction in maximal flow rates responsible for the classic truncation of the inspiratory and expiratory limbs seen on visual assessment of flow volume curves (24,25). Additionally, effort independent technologies such as vibration response imaging (VRI) and impulse oscillometry (IOS) have shown promise as alternative methods to detect and quantify CAO but the role of these technologies remains unclear (26-29).
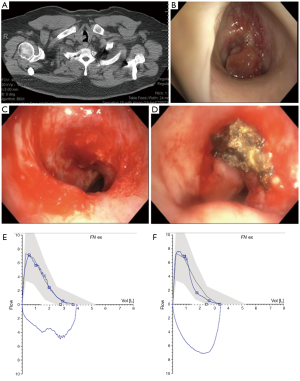
Diagnostic flexible bronchoscopy
White light flexible bronchoscopy as a diagnostic tool can provide a real-time assessment of CAO with the ability to distinguish tumor from associated blood, secretions or necrotic tissue. It is considered the gold standard in assessing morphology and degree of CAO. A major advantage of flexible bronchoscopy when compared to CT is the ability to biopsy lesions when obstructing pathology is unknown as histological subtyping is an important factor when contemplating therapeutic intervention. Tumors which are highly sensitive to radiation or chemotherapy, such as small cell lung cancer, may not require bronchoscopic intervention if the patient is minimally symptomatic and the obstruction is not critical. Flexible bronchoscopy in the setting of CAO can have significant risks, especially when performed without immediate access to the equipment and skill sets necessary to manage possible complications. Even gentle tumor manipulation can induce bleeding and edema which may convert a previously stable partial airway obstruction into an airway emergency. One must also be cautious about the use of sedative agents, particularly neuromuscular blocking agents, due to the potential to induce complete airway obstruction secondary to loss of respiratory drive muscle tone (30).
Therapeutic bronchoscopy overview
While systemic chemotherapy, radiotherapy and occasionally surgery may be options in the definitive long-term management of malignant CAO, bronchoscopic modalities are the cornerstone of treatment in the acute phase and often result in dramatic and near immediate symptomatic improvement. Successful patient outcomes require systematic decision making that includes the consideration of various factors that are discussed further in this section.
Technical success
Technical success in therapeutic bronchoscopy is typically defined as a post-intervention endoluminal diameter of at least 50% of the original airway. This is a largely arbitrary definition but is based on the underlying belief that symptoms generally do not occur unless airway obstruction exceeds 50% of the lumen. From a technical standpoint, when performed by experienced practitioners, successful recanalization of the airways in usually achievable with most studies citing technical success rates approximating 90% (31-33). It is important to note that this represents the appropriateness of patient selection, since all these studies had intrinsic significant selection bias. Hespanhol et al. in a retrospective review of over 800 therapeutic bronchoscopic procedures for severe obstruction within the trachea or mainstem bronchi developed a prediction model for technical success. In this model tracheal location, pure endobronchial disease and extrinsic compression predicted favorable results while left mainstem obstruction and mucosal infiltration of tumor were associated with reduced likelihood of success (34). Similarly, in the multi-institutional ACCP Quality Improvement Registry, Evaluation, and Education (AQuIRE) registry endoluminal obstruction and stent placement favored technical success while left mainstem obstruction favored failure. Additional predictors of failure highlighted in the AQuIRE registry data and not included in Hespanhol model included ASA score >3, renal failure, primary lung cancer and presence of tracheoesophageal fistula (33).
Complications of therapeutic bronchoscopy
Despite the extremely high technical success rates of therapeutic bronchoscopy, complications associated with therapeutic bronchoscopy are not rare. The first study to prospectively evaluate safety of rigid bronchoscopy in both malignant and benign disease found that up to 18% of patients developed respiratory complications though these were rarely life-threatening. Complications were more frequently observed in sicker patients with ASA scores of 3 or 4 and Karnofsky Performance Scale (KPS) below 70 (35). Another review from a larger, multi-institutional outcomes database of 554 therapeutic bronchoscopic procedures performed in 4 separate, high volume institutions found an overall complication rate of 19.8%, including hypoxia (oxygen saturation <90% for >1 minute), pneumothorax, escalation of care, bleeding, and hypotension. The overall 30-day mortality was 7.8%, however, only one of these deaths occurred perioperatively. This study included both benign and malignant disease, and although the majority of procedures were performed in benign disease, complications were more frequent (25%) in patients with malignancy, primarily due to increased bleeding complications. Other risk factors associated with complications included current tobacco use, hypertension, diabetes and presence of endobronchial disease (36). Recently the AQuIRE registry collaborators published the results from a large, prospective review of complications associated with therapeutic bronchoscopy for malignant CAO. This consisted of data from 1,115 procedures performed in 947 patients at 15 centers. Overall complications at 3.9% were much lower than previously reported, however, there was significant variability in complication rates between institutions, with institution specific complication rates ranging between 0.9% to 11.7%. When complications did occur, 54% resulted in adverse events such as extended hospitalization, permanent disability, death, or need for intervention to avoid permanent impairment (2.2% of all procedures), with 13.6% of reported complications resulting in death (0.5% of all procedures). Overall, the thirty-day mortality was 14.8%, with the majority of deaths related to progression of the underlying malignancy. Risk factors associated with all complications included urgent and emergent procedures, ASA >3, repeat procedures, and use of moderate sedation. Risk factors associated with death within 30 days included Zubrod score >1, ASA >3, presence of endoluminal or mixed obstruction and airway stent placement (37).
Decision making in malignant CAO
While it may be tempting to intervene at the first signs of endobronchial disease, one always needs to consider the delicate balance between risk and benefit. Unsuspected endobronchial disease can be discovered incidentally during bronchoscopy or on routine imaging without symptoms to suggest airway involvement (38,39). Except for the rare occasions when cure can be provided either through surgical or bronchoscopic means, it is important to remember that therapeutic interventions in the central airways are primarily palliative with the aim of relieving dyspnea or controlling hemorrhage as a bridge to more definitive therapy such as radiation or surgical resection. As such, in the absence of symptoms it is generally ill advised to intervene based solely on radiographic or bronchoscopic findings.
Intervening in an asymptomatic patient may be just as inappropriate as choosing to intervene in a profoundly ill patient when the risk of procedural complications and death are high. In carefully selected critically ill patients however, therapeutic bronchoscopic interventions can often have the most profound benefit. Previous work has shown that patients with advanced non-small cell lung cancer treated successfully with endoscopic modalities have survival rates similar to patients with similar staged NSCLC without CAO (40). Even in critically ill patients with CAO, previous work has shown that bronchoscopic interventions can often facilitate rapid extubation, de-escalation of care and long term survival (41).
There have been several studies that have attempted to identify patient and disease specific factors associated with successful palliation following therapeutic bronchoscopy for CAO. Patient perceived dyspnea and health-related quality of life (HRQOL) are important outcome measures in this population and interventions that provide a discernable benefit in either of these domains should be considered successful. Several studies have shown that it is possible to palliate symptoms in patients with dyspnea (33,42,43). Changes in dyspnea and HRQOL may not always parallel each other as HRQOL encompasses a variety of factors (33,41,42). As disease progresses, one can experience progressive decline in HRQOL related to progression of other cancer associated symptoms even as dyspnea improves. The largest study evaluating effects of therapeutic bronchoscopy on dyspnea and quality of life thus far was the AQuIRE registry discussed earlier. Paired data regarding pre and post intervention dyspnea and QOL was available in 197 patients for this analysis. In this cohort, 48% of patients had a significant improvement in dyspnea while 42% had significant improvement in HRQOL. With regards to HRQOL, those with more severe baseline dyspnea had the most benefit while those with lobar obstruction had reduced benefit (33).
Therapeutic bronchoscopy: tools and technical aspects
A variety of tools are available for the management of malignant CAO. Some tools, such as the rigid bronchoscope, have been used for almost two centuries and act as a platform for other modalities. Other tools have been customized for use in the airways after successful use in related fields such as minimally invasive surgery, gastroenterology and otorhinolaryngology. Central airway tumors are not uniform in appearance or location. Each tool possesses unique properties and can be applied safely and effectively to manage malignant airway tumors when these characteristics are understood by the physician wielding the instrument.
Mechanical debulking
Rigid bronchoscopy
The rigid bronchoscope is often described as the cornerstone of therapeutic bronchoscopic management of malignant CAO (Figure 5). Although some interventions can be performed with only flexible bronchoscopy, the large lumen rigid bronchoscope and associated instruments should always be readily available. In addition to allowing the use of larger instruments and high volume suction catheters which can be inserted through the rigid bronchoscope, the tip of the rigid scope itself is an effective tool for tumor debulking through the use of the beveled edge which can ‘core’ out an endoluminal lesion. A rigid dilating tracheal tube or serial rigid bronchoscopic dilators can also be used for mechanical dilation. The use of a rigid bronchoscope is also mandatory for the insertion and removal of silicone airway stents which will be discussed in detail in a later section.
Microdebrider
The microdebrider is a versatile tool for tumor debulking in the trachea and proximal mainstem bronchi. The device consists of a rotating cutting blade contained within a rigid metal suction catheter which can be inserted though the rigid bronchoscope. The amount of tissue shaved is proportional to the pressure applied at the target and inversely proportional to the rotation speed of the blade. To avoid inadvertent injury to the airway wall, we recommend utilizing suction to pull the tissue into the cutting orifice of the instrument rather than applying pressure to the target tissue. Since the instrument couples mechanical debulking with suction, blood and tissue debris can be simultaneously removed from the field to provide visualization of the target. As a non-thermal modality, it allows for rapid debulking without the need for any reduction in the fraction of inspired oxygen (FiO2).
The microdebrider is an efficacious tool in skilled hands (44-47). In the largest study evaluating this instrument for both malignant and benign central obstruction (48) the operators were able to consistently recanalize the airway lumen resulting in a mean residual airway obstruction of only 10%. Although complications are infrequent caution must be exercised while using this device as mishaps from inadvertently resecting airway wall and perforation of surrounding vascular structures can be catastrophic (49). Currently available models all require the rigid bronchoscope for use and are limited to accessing lesions located in the trachea and mainstem bronchi.
Thermal therapies
Thermal therapies utilize the biological effects of heat on cells to produce tissue destruction. Based on the temperature that is generated at the tissue level, a range of histopathological effects have been described (Table 1). All thermal tools have the potential to produce any of these effects depending upon factors such as the type of tissue, characteristics of the tool and operator-dependent application (50,52). The recommended initial power settings for airway procedures are 20–40 W and the FiO2 should be less than 40% to prevent airway fire.
Laser
Lasers (light amplification by stimulated emission of radiation) utilize the unique properties of spatial and temporal coherence and collimation to deliver a precise beam of thermal energy to a targeted area. The effect of the laser on tissue depends upon the wavelength of the light beam emitted, and the color of the target tissue. There are multiple lasers available for use within the tracheobronchial tree (Table 2).
The Nd:YAG laser is most frequently used laser for the treatment of malignant CAO (32,54-56). Due to its deep penetration and absorption by all proteinaceous tissue, it has superior coagulative and tissue ablative effect which is essential for debulking of endobronchial tumor. The Nd:YAP laser, with a wavelength of 1,340 nm, has a higher absorption coefficient in water than Nd:YAG and may provide similar coagulative and ablative effects although with a much more modest depth of penetration, which can be an advantage as risks of airway perforation and damage to surrounding structures are reduced. The Ho:YAG laser has a very high absorption coefficient in water which improves its ability to cut. Yet, it retains its coagulative properties and has been used to successfully treat malignant and benign airway obstruction (57). CO2 lasers, with a wavelength of 10,600 nm, provide very precise ‘cutting’ abilities. In the past, the CO2 laser required a rigid fiber which could not be inserted through a flexible bronchoscope. Recently, flexible CO2 fibers have been developed for flexible endoscopic use (58). A major limitation of the CO2 laser however, remains, its poor coagulative effects which limits its use in endobronchial malignancies with a propensity for bleeding.
Laser can be employed through a rigid or flexible bronchoscope (Figure 6). The laser should be directed parallel to the airway wall to reduce the risk of perforation and bronchovascular fistula. Power settings range from 20 to 50 W with effects changing from coagulative to ablative as the wattage increases. Depending upon the laser used, the entire range of histological effects can be produced and therefore, lasers can be used as a single modality for coagulation, hemostasis, cutting and vaporization. The depth of penetration depends upon the wavelength, distance of probe tip from the target lesion and optical depth (absorbance) of the tissue. With the Nd:YAG laser, keeping the tip of the probe at least 1cm from the tumor allows can minimize the risk of excessive penetration. A major advantage of laser when compared to other thermal therapies is that it does not interfere with pacemaker or defibrillator function.
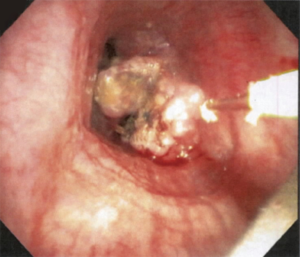
Laser therapy, alone or in conjunction with other tools, have been shown to be effective in managing malignant airway obstruction. The clinical effects are immediate and predictable. Retrospective studies have shown that laser can successfully establish airway patency (especially if tracheal or bronchial obstruction), improve the radiographic appearance of post-obstructive pneumonia and atelectasis, improve symptoms and control hemorrhage (32,55,59-61).
Two large, single-center retrospective studies have detailed their experiences with the use of laser in malignant CAO. Cavaliere et al. performed 2,610 laser resections in 1,838 patients over a 13-year period and were able to restore airway patency in 93% with a mortality of less than 0.4% (32). Moghissi et al. reviewed 1,159 patients with >50% obstruction of the bronchial lumen who underwent 2,235 procedures with Nd:YAG laser over a 21-year period. They found a 48% increase in the caliber of the bronchial lumen, 15% increase in the forced expiratory volume in one second and a low mortality rate (0.17%) (62).
Data supports the combined use of laser therapy with radiation therapy which has a delayed effect. In one study, the survival of patients who underwent emergent palliative laser photoresection and external beam radiation therapy was significantly better than historical cohorts who underwent emergent external beam radiation therapy alone (63). Additionally, a small, randomized controlled trial of 29 patients compared the combined efficacy of Nd:YAG laser therapy and high dose rate brachytherapy with Nd:YAG laser alone. These patients were all previously treated for non-small cell lung cancer and had central airway involvement, but were not eligible for further surgical, chemotherapeutic or external beam radiation treatment. In the group that underwent combined treatment, the symptom free survival was higher (median 8.5 vs. 2.8 months), progression free survival was higher (median 7.5 vs. 2.2 months) and number of repeated endoscopic procedures was lower (median 3 vs. 15) (64).
Contact electrocautery
Electrocautery is a form of thermal tissue destruction which utilizes the biological effects of electricity which is converted to heat energy to exert biological effects. The clinical effects can be unpredictable and factors influencing the effect include the type of current, the temperature at the tissue, tissue impedance and duration of contact. All electrosurgical generators use alternating current since it does not depolarize tissue. The waveforms from alternating current can be modulated to produce different tissue effects. A ‘cutting current’ is the basic sinusoidal non-modulated waveform and produces greater average power than alternating waveforms at a given voltage. This higher power translates into a smooth cutting action with minimal thermal damage. By modulating the waveform so that there are intermittent bursts of sine waves with high peak voltages, ‘coagulation’ current is produced. The high peak voltages result in higher temperatures at the tissue level producing thermal damage which is necessary for coagulation. A ‘blended current’ can also be used which produced alternating ‘cutting’ and ‘coagulating’ currents. Electrocautery tools can be monopolar or bipolar. In a monopolar mode, the electrical current flows through a single, active electrode into the tissue towards a grounding pad attached to the patient. In bipolar electrocautery, the electrical loop is closed and is present within the tissue.
The specific histological effects of electrocautery on the airways have been evaluated in animal (piglet) models and in vivo (65,66). Van Boxem et al. studied the effects of electrocautery in patients undergoing lung resection for non-small cell lung cancer, and showed the depth of necrosis seen on histopathology examination correlated well with the visual appearance on bronchoscopy and was directly proportional to the duration of application, causing damage to cartilage when applied for 3 or 5 s (66).
Electrocautery has been used within the airways since the 1930s (67). Its advantages include rapid and precise tissue cutting and excellent coagulative properties which can be used both the prophylactically prevent bleeding prior to debulking and to treat active mucosal hemorrhage. There are a multitude of rigid and flexible tools available for the delivery of electrocautery current (Figure 7). The reusable rigid electrocautery probe combines suctioning capabilities with electrocautery and can be used for both hemostasis and tissue destruction through the rigid bronchoscope. The flexible blunt probe can provide similar coagulative and desiccative effects through a flexible bronchoscope however without the added effect of simultaneous suction available with the rigid instrument. Exophytic lesions with a pedunculated stalk can be severed from the base with a flexible polypectomy snare which can be lassoed around the lesion. Reusable flexible electrocautery knives are a very effective tool. They allow the bronchoscopist to make controlled radial cuts along stenotic segments and reduce the risk of uncontrolled mucosal tears with subsequent balloon dilation. Finally, electrocautery forceps can be used to grasp tissue with the added function of coagulation. Although initially considered an exciting technology, thermal forceps are rarely of clinical use today due to the thermal damage in the biopsy specimens that limit pathologic assessment.
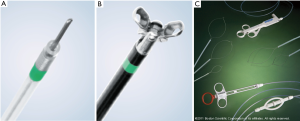
Although general anesthesia provides better operator comfort, electrocautery has been successfully used with local anesthesia and sedation (68,69). The efficacy and safety of electrocautery in the airways has been established in the management of benign and malignant CAO and early radiographically occult cancer (66,70,71). The quality of the data, which are typically confined to case reports and case series, is poor. However, from a clinical perspective, it is clearly an effective tool. In the largest descriptive study on the application of electrocautery in 94 patients with benign (30%) and malignant (70%) airway obstruction, electrocautery, when combined with other modalities such as balloon dilatation and airway stents produced substantial endoscopic improvement in 94% of cases and symptom improvement in 71% cases (70). An additional advantage of electrocautery is its relatively modest cost in comparison to competing technologies and it has been shown to produce comparable efficacy in re-establishing airway patency to Nd:YAG in a cost effective manner (72). Complications with electrocautery are rare but include hemorrhage, airway perforation, airway fire and scarring/stenosis (73). The risks of perforation and inflammation are minimized with the soft coagulation mode of electrocautery (74).
Argon plasma coagulation (APC)
APC is a form of noncontact electrosurgery. The APC system consists of an electrosurgical generator, argon gas cylinder and a transmitting probe (Figures 8,9). Argon is a colorless, odorless and chemically inert gas which passes through the transmitting probe and is ionized into argon plasma when exposed to electrical energy generated by a distal electrode. Argon plasma follows the path of least electrical resistance and moves in any direction towards the closest conductive tissue. Since APC is a form of monopolar electrosurgery, a grounding pad is necessary to complete the transmission of the electrical arc. The effect of APC on tissue can be modulated by adjusting the flow rate of the gas, power setting (watts) and the mode of energy delivery (forced, pulsed, precise). Argon flow should be set to the minimum rate necessary to produce desired tissue effects.
There are a variety of APC probes available with modified tips designed to direct the flow of argon plasma (forward, side or circumferential). Argon plasma produces a relatively superficial tissue effect with depth of penetration of only 1–3 mm. By “painting” the surface of the target tissue, the bronchoscopist can rapidly coagulate large areas of tissue. Another unique feature is that the depth of penetration is limited by the increase in resistance offered by coagulated and desiccated tissue. It is extremely useful to control hemorrhage (75) and can be used to destroy tissue in malignant tumors (76), benign strictures (77), respiratory papillomatosis (78), hereditary hemorrhagic telangiectasia (79) and granulation tissue (80,81). Similar to electrocautery, the data supporting the use of APC is limited to case reports and series (75-77,80,81). However, from a clinical perspective, it is a widely used, essential tool to control hemorrhage and debulk tumor in clinical practice.
APC is a thermal modality and thus has similar airway fire risks as other thermal modalities. The risk of airway perforation is theoretically much lower than electrocautery and laser. A unique safety concern related to APC is the risk of fatal gas embolism (82-85). This complication can be mitigated by using low flow rates (0.5 to 0.8 L/min), reducing the pulse durations at a single site, using optimal power settings (20–40 W) and by “painting” lesions rather than applying continued argon gas to a single site (86).
Cold therapy
Cryotherapy: probe and spray
Cryotherapy is a method of tissue destruction that consists of exposed tissue is subjected to repeated cycles of extremely cold temperatures (below −40 °C). Cryotherapy works through the Joules-Thomson effect in which certain gases (such as carbon dioxide and nitrous oxide N2O) when moved from high pressure to low pressure, expand rapidly resulting in temperature drops which can rapidly freeze target tissue. Low temperatures result in intracellular and extracellular freezing of water which then recrystallizes when thawed resulting in cellular dehydration. Cryotherapy also induces tissue ischemia through local vasoconstriction, increased blood viscosity and platelet aggregation leading to microthrombi formation and finally, cell death through apoptosis.
Cell death depends upon several factors. In vivo animal experiments have shown that the lethal temperatures for single cool-thaw cycles vary based on the type of tissue treated (87). Overall, destruction of tumor occurs only at a very low temperature (at minimum −40 °C). A faster rate of cooling does produce more extensive cellular damage and destruction. However, it is not critical for cellular destruction as lethal freezing has been shown to occur at slower cooling rates as well. Exposing cells to varying temperatures for varying durations also increases cellular destruction. A slow thaw rate, permitting complete thawing of frozen tissue, is a very important factor affecting cell death since recrystallization of the water, which occurs during thawing, results in mechanical damage to cells. Finally, repeat cool-thaw cycles increase cellular damage and promote larger areas of necrosis (88,89).
The cryotherapy system consists of a cryogen gas stored under high pressure and the cryosurgery device (Figure 10). Nitrous oxide gas is the most commonly used gas for cryotherapy. The cryosurgery device consists of a cryoprobe, a line to connect the cryoprobe to the gas container and a power console which regulates the flow of the cryogen. The cryoprobes are long, hollow tubes that carry the cryogen to the target tissue when activated by the power console. The flexible cryoprobe (available in 1.9 and 2.3 mm in diameter) can be passed through the working channel of a flexible bronchoscope for application. Rigid and semi-rigid cryoprobes which are inserted through the rigid bronchoscope are also available but rarely used as they do not provide significant advantage over the flexible probe.
The techniques of application of cryotherapy vary based on the indication. Cryo-recanalization is the broad term applied to the use of cryotherapy to establish patency of an occluded airway. In this case, a cryoprobe is placed in contact with the target tissue, malignant or benign, and activated for a short duration to create an “ice ball” of tissue at the tip of the probe. The duration of activation to allow tissue adhesion with the probe has been reported from 2 to 20 s (90-92). We recommend activation until adequate adhesion is visualized by the formation of an “ice ball” involving the target tissue only. The probe and bronchoscope is then pulled away from the lesion to remove attached tissue. The bronchoscope, probe and tissue must be carefully withdrawn from the airway en-bloc without touching the airway walls. The tissue and probe are placed in saline to allow thawing and separation of tissue from the probe. This method is fast and effective. When cryotherapy is used to treat early stage lung cancer or benign endobronchial lesions such as papillomatosis, several cycles of cooling and thawing are applied to the target lesion. These cycles are performed by placing the probe on the lesion of interest, activating for 30 s and then allowing the target to thaw completely before repeating this maneuver usually with three freeze-thaw cycles. Another method of application is spray cryotherapy in which liquid nitrogen is sprayed through a flexible catheter on the target lesion producing temperatures as low as −196 °C without contact. Since barotrauma, including pneumothorax and pneumomediastinum resulting from the inability of the rapidly expanding gas to escape through a closed system, can occur, careful planning using an open ventilating system to permit escape of gas during use is necessary (93).
Cryotherapy has multiple applications. It has been used in the multimodality approach to relieve benign and malignant CAO (90,94-96). Dysplastic lesions and early, low-grade malignancies of the airways have been treated with cryotherapy with acceptable long-term control (97). As an adjunct tool, it is often used to remove blood clots and necrotic tumor from the airways. There have been multiple studies have retrospectively reviewed the success of cryotherapy for malignant airway obstruction with the largest studies reviewed and summarized in Table 3. Similar to the microdebrider, cryotherapy can be used in patients with high oxygen requirements and without the need to reduced inspired FiO2.
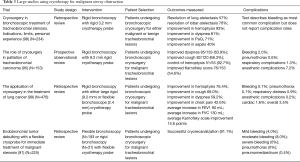
Full table
Airway stent
Airway stents are prosthetic devices used to maintain patency of the airway lumen. A stent buttresses the airway wall against tumor ingrowth or extrinsic compression once patency of the airway has been partially or completed established. Currently, stents are broadly classified based the composed material as either silastic or self-expanding metallic. The Montgomery T-tube is a T-shaped silicone tube developed for the airways in 1965 and is still used for the treatment of subglottic and proximal tracheal obstruction. The main disadvantage of the t-tube is the need for a tracheostomy to secure the horizontal limb. The first purely endoluminal silicone stents were introduced by Jean-François Dumon in 1990. Silicone stents typically are produced with studs along the anterior wall to reduce the risk of migration and contact from mucosa and can be straight tubes (tracheal or bronchial) or bifurcated (Y stents). Additionally, the silicone stents can be easily cut with standard scissors allowing length of the limbs to be modified prior to placement. Multiple variations (including custom stents) are available through a variety of manufactures (Table 4, Figure 11). Self-expanding metallic stents (SEMS) are typically composed of the metal alloy nitinol (nickel and titanium). Nitinol possesses favorable characteristics of super-elasticity and shape memory which allows the stent to be deformed during deployment and regain its original shape when heated to its transformation temperature within the airways. SEMS can be bare or covered (partially or completely) with silicone or polyurethane to prevent ingrowth of tumor and debris. Major advantages of SEMS compared to silastic stents include easy of deployment, higher inner to outer diameter ratio and adaptability in tortuous airways. A detailed description of each stent is beyond the scope of this review.
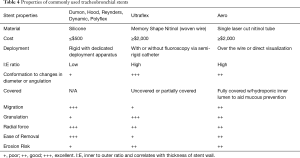
Full table
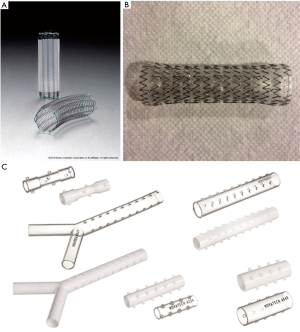
Deployment of airway stents requires sound clinical judgement, an appreciable level of skill, thoughtful consideration and anticipation of complications which can occur during or after placement. There are several considerations which must be addressed when contemplating stent placement. Stenting does not treat the tumor; so, the objective of an airway stent is either entirely palliative or for treatment and prevention of symptoms of CAO to allow an individual to receive systemic therapy. As stents are foreign bodies, which can worsen certain symptoms such as cough and place the patient at risk for late complication, one must be relatively confident that symptoms are primarily attributable to the airway obstruction. The risk of tumor growth or recurrence and options for systemic therapy are additional factors that influence decision making. For extrinsic compression, airway stenting is the only bronchoscopic modality available which can result in prolonged airway patency. In purely endoluminal disease stenting is usually not a primary modality however can be considered following debulking for treatment of residual obstruction or to obstruct tumor regrowth.
Choosing the optimal stent for a lesion begins with integration of knowledge of the normal anatomy and integrating this with details obtained from imaging and white light bronchoscopy (100). A perfectly sized stent fits firmly along the airway wall and is neither too large (causes granulation and mucosal ischemia) nor small (promotes migration) and extends approximately 5mm proximal and distal to the obstruction (100).
Silicone stent deployment is complex and requires rigid bronchoscopy and general anesthesia. They can be placed by either the push or pull technique, though the pull technique is often favored. Stents are typically deployed into the airways by loading a lubricated stent into the barrel of an introducer with a specialized stent loader. The introducer is then placed into the lumen of a rigid bronchoscope that is placed into the desired location in the airways. The stent is pushed out of the introducer as the rigid bronchoscope is withdrawn slightly. Slight distal placement is preferable (pull technique) as it is easier to withdrawal the stent proximally than to push it distally. After stent introduction, manipulation with rigid forceps under direct visualization is usually required for ideal placement. While it can be challenging to employ these stents, they have distinct advantages. Silicone stents are easy to remove and reposition and they can be customized on site for a better fit. On the other hand, depending upon the manufacturer, SEMS have several methods of deployment. They can be placed using a flexible bronchoscope with or without fluoroscopic guidance and a guide wire. SEMS cannot be easily repositioned if placed proximally and removal of SEMS can be difficult. Stents hamper normal mucociliary clearance and respiratory toilet with hypertonic saline nebulization with or without a bronchodilator several times a day to clear secretions is advisable.
Multiple studies have retrospectively reviewed the safety and efficacy of silicone stents and SEMS (12) (Table 5). Several large studies describing the use of stents in CAO show significant variability in the patient population and include both benign and malignant disease (101-103). Additionally, some describe their experience with both silicone and metallic stents (102,103). Despite this heterogeneity, some clinically meaningful conclusions can be drawn. These studies reveal that clinically significant CAO requiring an intervention is infrequent, that it is possible to successfully and safely improved airway patency and alleviate symptoms and that placement of stents require a level of expertise more often available at centers of excellence. These studies also reinforce the need for caution in airway stent placement due to the occurrence of complications such as migration, obstruction from secretions and granulation and less commonly, ulcerations, perforations and infections.
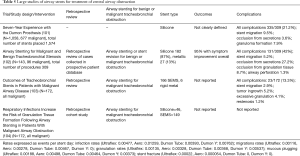
Full table
While there continues to be considerable interest in the development of drug eluting airway stents to increase the efficacy of airway stenting and reduce complications, there is not much evidence to support the routine clinical use of such stents (105).
Most literature related to airway stent complications focus on rates of complications. Ost et al. conducted the largest study of airway stent related complications in malignant disease (104). In this retrospective review of 195 stent procedures performed in 172 patients, 73 patients developed stent related respiratory tract infections with a median time to infection of 1 month after stent placement and these infections resulted in significant sequelae with the majority of patients requiring hospitalization and 23% of patients dying within 14 days of infection. Stent migration, which can be a catastrophic event resulting in asphyxiation when it occurs within the trachea, occurred in 27/163 patients with a median time to event of 1.43 months. When silicone Y-stents, which very rarely migrate, where excluded from analysis, the highest migration rate was seen in tube silicone stents.
Delayed bronchoscopic methods
Photodynamic therapy (PDT)
PDT is the term applied for the use of a specific wavelength of light to activate a systemically or topically administered photosensitizing agent that selectively accumulates in tumor cells. PDT works by activation of a pre-administered photosensitizer tumor cells. An effective photosensitizer should preferentially accumulate within tumor cells compared to healthy cells, be stable and possess appropriate extinction coefficients. Photosensitizers are categorized as porphyrins (first, second and third generation) and non-porphyrins. At this time, none of the available photosensitizers possess completely favorable characteristics but the development of nanocarriers with improved permeability and retention within tumor cells may be lead to a new era in PDT (106). The most widely used photosensitizer in the United States is Photofrin which is activated when exposed to a light source of 630 nm wavelength (107).
When exposed to light, the photosensitizer absorbs photons and releases the energy excess onto the surrounding structures. As energy is transferred, free radicals are generated to produce reactive oxygen species (ROS). This is a Type I reaction. Alternatively, a type II reaction can occur with energy transferred to molecular oxygen forming highly reactive singlet oxygen. Both of these activated oxygen molecules have short half-lives which means that the toxic effects are relatively localized (107). The toxic effects of photodynamic therapy on tumor cells are three-fold: a direct cytotoxic effect of high levels of ROS within tumor cells, damage to the surrounding microvasculature feeding the tumor and recruitment of a local immune response which results in both immediate and delayed effects.
A photosensitizing agent needs to be administered intravenously prior to bronchoscopy. Optimally administration should occur between 40 to 50 h prior to bronchoscopy to allow the photosensitizer to wash out of the normal mucosa, mitigating damage to healthy tissue. Subsequently, flexible bronchoscopy is performed in which a light source (usually a laser fiber emitting nonthermal light) emitting the optimal wavelength for the photosensitizer is introduced through the working channel and activated near the target tissue. It is recommended to apply 200 Joules per cm treated and the energy dose can be modified based on the size and location of the tumor. This can be repeated for a total of 3 sessions 6 weeks apart. A bronchoscopy needs to be performed 3 days after the first treatment session to examine the mucosa and clear the airways of sloughed mucosa. A second activation of residual photosensitizer in the tumor can be performed if deemed necessary during the bronchoscopy to clear the airway up to five days after initial administration.
PDT has been used for palliation in patients who have inoperable CAO. Response rates range between 41% to 100% (108). Although there is significant heterogeneity in the clinical outcomes assessed, most studies report improvements in dyspnea, cough and hemoptysis (61,109,110). PDT combined with external beam radiation therapy has been compared to external beam radiation therapy alone in a randomized trial of 41 patients with inoperable and obstructing central airway tumors (109). Patients who had PDT and external beam radiation were more likely to have complete clearance of airway obstruction on bronchoscopy (70% vs. 9.5%) and lower recurrence rates, however, there was no difference in overall survival. PDT also was compared to Nd:YAG laser in a randomized trial of 211 patients with advanced lung cancer and endobronchial obstruction. Although overall survival was similar in both groups with PDT demonstrating a better clinical response rate at one month compared to Nd:YAG laser (55% vs. 30%) (110).
The most common side effect of PDT is photosensitivity, and patients must take precautions to avoid exposure to light for at least 2 weeks after systemic administration of the photosensitizer (111). Specific to PDT, mucosal sloughing which may cause respiratory failure is expected and may require multiple bronchoscopies for clearance of necrotic tissue. Other complications reported with treatment include hemoptysis, bronchitis, pneumonias and severe endotracheal candidiasis (108).
The obvious advantages of PDT are that is can be employed in patients who are not surgical candidate, who cannot tolerate general anesthesia and when oxygen requirements are too high to use thermal ablative techniques. However, as with many technologies adapted for endobronchial therapy, there is limited data to identify which patients with CAO would benefit from PDT over alternative ablative techniques. Patients with CAO have increased morbidity and mortality and are often nearing the end of their lives. This technique obligates certain lifestyle modifications to prevent complications (avoiding exposure to light for between two and six weeks after administration of the photosensitizer to prevent photosensitive reactions) and requires a series of bronchoscopic procedures.
Endoluminal brachytherapy
Brachytherapy is the delivery of radiation to a tumor from a source located very close to the tumor allowing high dose radiation while minimizing the radiation exposure to heathy tissue. Although there are several methods of placing the source of radiation near the tumor (endoluminal, interstitial and percutaneous), endoluminal brachytherapy is predominantly used for curative and palliative treatment of endobronchial tumors. In this method, a blind-tipped catheter is placed close to the tumor under bronchoscopic guidance through the nose or artificial airway and secured. The after loading technique allows the radiation oncologist to deploy beads of iridium-192 through the catheter after it is placed and minimizes radiation exposure to technical staff. The radiation can be delivered by a low-dose rate (treatment is continuous in one session over 20–60 h), intermediate-dose rate and high-dose rate. The latter two requires multiple bronchoscopies for placement of the catheter since these sessions are repeated several times (112).
Studies evaluating the use of endobronchial brachytherapy are quite heterogenous but generally report significant improvement in symptoms of dyspnea, cough, hemoptysis and radiographic findings of atelectasis (17,64,113-115). At this time, there is no evidence to direct patient selection, support a particular dose-rate method or predict tumor responsiveness other than size of the tumor. It is reasonable to consider brachytherapy for endobronchial lesions arising in the segmental bronchi and extending peribronchially which are inaccessible to other ablative technology. The development of severe radiation bronchitis fistulas, abscesses, hemorrhage (even fatal) and infection have been observed (116-119). The decision to use endoluminal brachytherapy must be made based on the patient’s tumor, severity of symptoms, need for urgent recanalization and capabilities of the institution. Since it has a delayed effect, it is not an efficient method to treat severely symptomatic malignant CAO.
Conclusions
Malignant CAO can significantly impact a patient’s quality of life as well as their eligibility for systemic therapies. In trained hands, bronchoscopic interventions can provide a minimally invasive option for restoring patency and provide rapid symptomatic improvement even in the critically ill patients. Multiple tools are available to treat intrinsic, extrinsic and mixed airway obstruction. Currently available modalities for rapidly restoring airway patency include mechanical debulking, thermal tools, cryotherapy and airway stents. Additionally, delayed bronchoscopic methods such as photodynamic therapy and brachytherapy, although less commonly employed, can also be utilized in select patients with non-emergent obstruction. Each modality possesses unique characteristics and can be invaluable when employed in the optimal setting. Assessment of potential benefits and risks of interventions in each individual patient during the decision-making process forms the basis of the management of malignant CAO.
Acknowledgements
None.
Footnote
Conflicts of Interest: The authors have no conflicts of interest to declare.
References
- Cox JD, Yesner RA. Causes of treatment failure and death in carcinoma of the lung. Yale J Biol Med 1981;54:201-7. [PubMed]
- Marchioni A, Lasagni A, Busca A, et al. Endobronchial metastasis: an epidemiologic and clinicopathologic study of 174 consecutive cases. Lung Cancer 2014;84:222-8. [Crossref] [PubMed]
- Macchiarini P. Primary tracheal tumours. Lancet Oncol 2006;7:83-91. [Crossref] [PubMed]
- Harpole DH, Feldman JM, Buchanan S, et al. Bronchial carcinoid tumors: a retrospective analysis of 126 patients. Ann Thorac Surg 1992;54:50-4; discussion 4-5. [Crossref] [PubMed]
- Skuladottir H, Hirsch FR, Hansen HH, et al. Pulmonary neuroendocrine tumors: incidence and prognosis of histological subtypes. A population-based study in Denmark. Lung Cancer 2002;37:127-35. [Crossref] [PubMed]
- Braman SS, Whitcomb ME. Endobronchial metastasis. Arch Intern Med 1975;135:543-7. [Crossref] [PubMed]
- Abers MS, Sandvall BP, Sampath R, et al. Postobstructive Pneumonia: An Underdescribed Syndrome. Clin Infect Dis 2016;62:957-61. [Crossref] [PubMed]
- Jabbardarjani H, Herth F, Kiani A, et al. Central Airway Obstruction Masquerading as Difficult-to-Treat Asthma: A Retrospective Study. J Bronchology Interv Pulmonol 2009;16:6-9. [Crossref] [PubMed]
- Henschke CI, Lee IJ, Wu N, et al. CT screening for lung cancer: prevalence and incidence of mediastinal masses. Radiology 2006;239:586-90. [Crossref] [PubMed]
- Ducrocq X, Thomas P, Massard G, et al. Operative risk and prognostic factors of typical bronchial carcinoid tumors. Ann Thorac Surg 1998;65:1410-4. [Crossref] [PubMed]
- Ernst A, Feller-Kopman D, Becker HD, et al. Central airway obstruction. Am J Respir Crit Care Med 2004;169:1278-97. [Crossref] [PubMed]
- Wahidi MM, Herth FJ, Ernst A. State of the art: interventional pulmonology. Chest 2007;131:261-74. [Crossref] [PubMed]
- Kwong JS, Adler BD, Padley SP, et al. Diagnosis of diseases of the trachea and main bronchi: chest radiography vs CT. AJR Am J Roentgenol 1993;161:519-22. [Crossref] [PubMed]
- Sorantin E, Geiger B, Lindbichler F, et al. CT-based virtual tracheobronchoscopy in children--comparison with axial CT and multiplanar reconstruction: preliminary results. Pediatr Radiol 2002;32:8-15. [Crossref] [PubMed]
- Harris K, Alraiyes AH, Attwood K, et al. Reporting of central airway obstruction on radiology reports and impact on bronchoscopic airway interventions and patient outcomes. Ther Adv Respir Dis 2016;10:105-12. [Crossref] [PubMed]
- Walker CM, Abbott GF, Greene RE, et al. Imaging pulmonary infection: classic signs and patterns. AJR Am J Roentgenol 2014;202:479-92. [Crossref] [PubMed]
- Lee P, Kupeli E, Mehta AC. Therapeutic bronchoscopy in lung cancer. Laser therapy, electrocautery, brachytherapy, stents, and photodynamic therapy. Clin Chest Med 2002;23:241-56. [Crossref] [PubMed]
- Miller RD, Hyatt RE. Obstructing lesions of the larynx and trachea: clinical and physiologic characteristics. Mayo Clin Proc 1969;44:145-61. [PubMed]
- Miller RD, Hyatt R. Evaluation of obstructing lesions of the trachea and larynx by flow-volume loops. Am Rev Respir Dis 1973;108:475-81. [PubMed]
- Gascoigne AD, Corris PA, Dark JH, et al. The biphasic spirogram: a clue to unilateral narrowing of a mainstem bronchus. Thorax 1990;45:637-8. [Crossref] [PubMed]
- Gelb AF, Tashkin DP, Epstein JD, et al. Nd-YAG laser surgery for severe tracheal stenosis physiologically and clinically masked by severe diffuse obstructive pulmonary disease. Chest 1987;91:166-70. [Crossref] [PubMed]
- Rotman HH, Liss HP, Weg JG. Diagnosis of upper airway obstruction by pulmonary function testing. Chest 1975;68:796-9. [Crossref] [PubMed]
- Miller MR, Pincock AC, Oates GD, et al. Upper airway obstruction due to goitre: detection, prevalence and results of surgical management. Q J Med 1990;74:177-88. [PubMed]
- Raposo LB, Bugalho A, Gomes MJ. Contribution of flow-volume curves to the detection of central airway obstruction. J Bras Pneumol 2013;39:447-54. [Crossref] [PubMed]
- Modrykamien AM, Gudavalli R, McCarthy K, et al. Detection of upper airway obstruction with spirometry results and the flow-volume loop: a comparison of quantitative and visual inspection criteria. Respir Care 2009;54:474-9. [PubMed]
- Guntupalli KK, Reddy RM, Loutfi RH, et al. Evaluation of obstructive lung disease with vibration response imaging. J Asthma 2008;45:923-30. [Crossref] [PubMed]
- Becker HD, Slawik M, Miyazawa T, et al. Vibration response imaging as a new tool for interventional-bronchoscopy outcome assessment: a prospective pilot study. Respiration 2009;77:179-94. [Crossref] [PubMed]
- Pornsuriyasak P, Ploysongsang Y. Impulse Oscillometry System in Diagnosis of Central Airway Obstruction in Adults: Comparison with Spirometry and Body Plethysmography. Chest 2009;136:123S. [Crossref]
- Handa H, Huang J, Murgu SD, et al. Assessment of central airway obstruction using impulse oscillometry before and after interventional bronchoscopy. Respir Care 2014;59:231-40. [Crossref] [PubMed]
- Erdös G, Tzanova I. Perioperative anaesthetic management of mediastinal mass in adults. Eur J Anaesthesiol 2009;26:627-32. [Crossref] [PubMed]
- Mathisen DJ, Grillo HC. Endoscopic relief of malignant airway obstruction. Ann Thorac Surg 1989;48:469-73; discussion 73-5. [Crossref] [PubMed]
- Cavaliere S, Venuta F, Foccoli P, et al. Endoscopic treatment of malignant airway obstructions in 2,008 patients. Chest 1996;110:1536-42. [Crossref] [PubMed]
- Ost DE, Ernst A, Grosu HB, et al. Therapeutic bronchoscopy for malignant central airway obstruction: success rates and impact on dyspnea and quality of life. Chest 2015;147:1282-98. [Crossref] [PubMed]
- Hespanhol V, Magalhães A, Marques A. Neoplastic severe central airways obstruction, interventional bronchoscopy: a decision-making analysis. J Thorac Cardiovasc Surg 2013;145:926-32. [Crossref] [PubMed]
- Perrin G, Colt HG, Martin C, et al. Safety of interventional rigid bronchoscopy using intravenous anesthesia and spontaneous assisted ventilation. A prospective study. Chest 1992;102:1526-30. [Crossref] [PubMed]
- Ernst A, Simoff M, Ost D, et al. Prospective risk-adjusted morbidity and mortality outcome analysis after therapeutic bronchoscopic procedures: results of a multi-institutional outcomes database. Chest 2008;134:514-9. [Crossref] [PubMed]
- Ost DE, Ernst A, Grosu HB, et al. Complications Following Therapeutic Bronchoscopy for Malignant Central Airway Obstruction: Results of the AQuIRE Registry. Chest 2015;148:450-71. [Crossref] [PubMed]
- Schwarz C, Schönfeld N, Bittner RC, et al. Value of flexible bronchoscopy in the pre-operative work-up of solitary pulmonary nodules. Eur Respir J 2013;41:177-82. [Crossref] [PubMed]
- Lindfors KK, Meyer JE, Busse PM, et al. CT evaluation of local and regional breast cancer recurrence. AJR Am J Roentgenol 1985;145:833-7. [Crossref] [PubMed]
- Chhajed PN, Baty F, Pless M, et al. Outcome of treated advanced non-small cell lung cancer with and without central airway obstruction. Chest 2006;130:1803-7. [Crossref] [PubMed]
- Mahmood K, Wahidi MM, Thomas S, et al. Therapeutic bronchoscopy improves spirometry, quality of life, and survival in central airway obstruction. Respiration 2015;89:404-13. [Crossref] [PubMed]
- Amjadi K, Voduc N, Cruysberghs Y, et al. Impact of interventional bronchoscopy on quality of life in malignant airway obstruction. Respiration 2008;76:421-8. [Crossref] [PubMed]
- Oviatt PL, Stather DR, Michaud G, et al. Exercise capacity, lung function, and quality of life after interventional bronchoscopy. J Thorac Oncol 2011;6:38-42. [Crossref] [PubMed]
- Lunn W, Garland R, Ashiku S, et al. Microdebrider bronchoscopy: a new tool for the interventional bronchoscopist. Ann Thorac Surg 2005;80:1485-8. [Crossref] [PubMed]
- Melendez J, Cornwell L, Green L, et al. Treatment of large subglottic tracheal schwannoma with microdebrider bronchoscopy. J Thorac Cardiovasc Surg 2012;144:510-2. [Crossref] [PubMed]
- Wahla AS, Khan I, Bellinger C, et al. Use of microdebrider bronchoscopy for the treatment of endobronchial leiomyoma. Clin Respir J 2011;5:e4-7. [Crossref] [PubMed]
- Kennedy MP, Morice RC, Jimenez CA, et al. Treatment of bronchial airway obstruction using a rotating tip microdebrider: a case report. J Cardiothorac Surg 2007;2:16. [Crossref] [PubMed]
- Casal RF, Iribarren J, Eapen G, et al. Safety and effectiveness of microdebrider bronchoscopy for the management of central airway obstruction. Respirology 2013;18:1011-5. [Crossref] [PubMed]
- Kühnel T, Hosemann W, Rothammer R. Evaluation of powered instrumentation in out-patient revisional sinus surgery. Rhinology 2001;39:215-9. [PubMed]
- Thomsen S. Pathologic analysis of photothermal and photomechanical effects of laser-tissue interactions. Photochem Photobiol 1991;53:825-35. [Crossref] [PubMed]
- Berlien HP. Thermal effects on biological tissues. Applied laser medicine. Berlin: Springer; 2003.
- Cummins L, Nauenberg M. Thermal effects of laser radiation in biological tissue. Biophys J 1983;42:99-102. [Crossref] [PubMed]
- Miller RJ, Murgu SD. Bronchoscopic resection of an exophytic endoluminal tracheal mass. Ann Am Thorac Soc 2013;10:697-700. [Crossref] [PubMed]
- Dumon JF, Reboud E, Garbe L, et al. Treatment of tracheobronchial lesions by laser photoresection. Chest 1982;81:278-84. [Crossref] [PubMed]
- Hetzel MR, Nixon C, Edmondstone WM, et al. Laser therapy in 100 tracheobronchial tumours. Thorax 1985;40:341-5. [Crossref] [PubMed]
- Cavaliere S, Foccoli P, Farina PL. Nd:YAG laser bronchoscopy. A five-year experience with 1,396 applications in 1,000 patients. Chest 1988;94:15-21. [Crossref] [PubMed]
- Squiers JJ, Teeter WA, Hoopman JE, et al. Holmium:YAG laser bronchoscopy ablation of benign and malignant airway obstructions: an 8-year experience. Lasers Med Sci 2014;29:1437-43. [Crossref] [PubMed]
- Brase C, Schwitulla J, Künzel J, et al. First experience with the fiber-enabled CO2 laser in stapes surgery and a comparison with the "one-shot" technique. Otol Neurotol 2013;34:1581-5. [Crossref] [PubMed]
- Kvale PA, Eichenhorn MS, Radke JR, et al. YAG laser photoresection of lesions obstructing the central airways. Chest 1985;87:283-8. [Crossref] [PubMed]
- Brutinel WM, Cortese DA, McDougall JC, et al. A two-year experience with the neodymium-YAG laser in endobronchial obstruction. Chest 1987;91:159-65. [Crossref] [PubMed]
- Moghissi K, Dixon K, Stringer M, et al. The place of bronchoscopic photodynamic therapy in advanced unresectable lung cancer: experience of 100 cases. Eur J Cardiothorac Surg 1999;15:1-6. [Crossref] [PubMed]
- Moghissi K, Dixon K. Bronchoscopic NdYAG laser treatment in lung cancer, 30 years on: an institutional review. Lasers Med Sci 2006;21:186-91. [Crossref] [PubMed]
- Desai SJ, Mehta AC, VanderBrug Medendorp S, et al. Survival experience following Nd:YAG laser photoresection for primary bronchogenic carcinoma. Chest 1988;94:939-44. [Crossref] [PubMed]
- Chella A, Ambrogi MC, Ribechini A, et al. Combined Nd-YAG laser/HDR brachytherapy versus Nd-YAG laser only in malignant central airway involvement: a prospective randomized study. Lung Cancer 2000;27:169-75. [Crossref] [PubMed]
- Verkindre C, Brichet A, Maurage CA, et al. Morphological changes induced by extensive endobronchial electrocautery. Eur Respir J 1999;14:796-9. [Crossref] [PubMed]
- van Boxem TJ, Westerga J, Venmans BJ, et al. Tissue effects of bronchoscopic electrocautery: bronchoscopic appearance and histologic changes of bronchial wall after electrocautery. Chest 2000;117:887-91. [Crossref] [PubMed]
- Gilroy F. Primary malignant tumors of the lower third of the trachea: report of a case with successful treatment by electrofulguration and deep X-rays. Archives of Otolaryngology 1932;16:182-7. [Crossref]
- Sutedja G, van Kralingen K, Schramel FM, et al. Fibreoptic bronchoscopic electrosurgery under local anaesthesia for rapid palliation in patients with central airway malignancies: a preliminary report. Thorax 1994;49:1243-6. [Crossref] [PubMed]
- Sagawa M, Sato M, Takahashi H, et al. Electrosurgery with a fiberoptic bronchoscope and a snare for endotracheal/endobronchial tumors. J Thorac Cardiovasc Surg 1998;116:177-9. [Crossref] [PubMed]
- Wahidi MM, Unroe MA, Adlakha N, et al. The use of electrocautery as the primary ablation modality for malignant and benign airway obstruction. J Thorac Oncol 2011;6:1516-20. [Crossref] [PubMed]
- Vonk-Noordegraaf A, Postmus PE, Sutedja TG. Bronchoscopic treatment of patients with intraluminal microinvasive radiographically occult lung cancer not eligible for surgical resection: a follow-up study. Lung Cancer 2003;39:49-53. [Crossref] [PubMed]
- Boxem T, Muller M, Venmans B, et al. Nd-YAG laser vs bronchoscopic electrocautery for palliation of symptomatic airway obstruction: a cost-effectiveness study. Chest 1999;116:1108-12. [Crossref] [PubMed]
- Horinouchi H, Miyazawa T, Takada K. Safety Study of Endobronchial Electrosurgery for Tracheobronchial Lesions: Multicenter Prospective Study. Journal of Bronchology 2008;15:228-32. [Crossref]
- Tremblay A, Marquette CH. Endobronchial electrocautery and argon plasma coagulation: a practical approach. Can Respir J 2004;11:305-10. [Crossref] [PubMed]
- Morice RC, Ece T, Ece F, et al. Endobronchial argon plasma coagulation for treatment of hemoptysis and neoplastic airway obstruction. Chest 2001;119:781-7. [Crossref] [PubMed]
- Reichle G, Freitag L, Kullmann HJ, et al. Argon plasma coagulation in bronchology: a new method--alternative or complementary? Pneumologie 2000;54:508-16. [Crossref] [PubMed]
- Keller CA, Hinerman R, Singh A, et al. The use of endoscopic argon plasma coagulation in airway complications after solid organ transplantation. Chest 2001;119:1968-75. [Crossref] [PubMed]
- Bergler W, Hönig M, Götte K, et al. Treatment of recurrent respiratory papillomatosis with argon plasma coagulation. J Laryngol Otol 1997;111:381-4. [Crossref] [PubMed]
- Bergler W, Riedel F, Baker-Schreyer A, et al. Argon plasma coagulation for the treatment of hereditary hemorrhagic telangiectasia. Laryngoscope 1999;109:15-20. [Crossref] [PubMed]
- Sato M, Terada Y, Nakagawa T, et al. Successful use of argon plasma coagulation and tranilast to treat granulation tissue obstructing the airway after tracheal anastomosis. Chest 2000;118:1829-31. [Crossref] [PubMed]
- Shu L, Hu Y, Wei R. Argon Plasma Coagulation Combined with a Flexible Electronic Bronchoscope for Treating Foreign Body Granulation Tissues in Children's Deep Bronchi: Nine Case Reports. J Laparoendosc Adv Surg Tech A 2016;26:1039-40. [Crossref] [PubMed]
- Reddy C, Majid A, Michaud G, et al. Gas embolism following bronchoscopic argon plasma coagulation: a case series. Chest 2008;134:1066-9. [Crossref] [PubMed]
- Shaw Y, Yoneda KY, Chan AL. Cerebral gas embolism from bronchoscopic argon plasma coagulation: a case report. Respiration 2012;83:267-70. [Crossref] [PubMed]
- Kizer N, Zighelboim I, Rader JS. Cardiac arrest during laparotomy with argon beam coagulation of metastatic ovarian cancer. Int J Gynecol Cancer 2009;19:237-8. [Crossref] [PubMed]
- Ousmane ML, Fleyfel M, Vallet B. Venous gas embolism during liver surgery with argon-enhanced coagulation. Eur J Anaesthesiol 2002;19:225. [Crossref] [PubMed]
- Feller-Kopman D, Lukanich JM, Shapira G, et al. Gas flow during bronchoscopic ablation therapy causes gas emboli to the heart: a comparative animal study. Chest 2008;133:892-6. [Crossref] [PubMed]
- Gage AA, Baust J. Mechanisms of tissue injury in cryosurgery. Cryobiology 1998;37:171-86. [Crossref] [PubMed]
- Gage AA, Guest K, Montes M, et al. Effect of varying freezing and thawing rates in experimental cryosurgery. Cryobiology 1985;22:175-82. [Crossref] [PubMed]
- Mazur P. The role of intracellular freezing in the death of cells cooled at supraoptimal rates. Cryobiology 1977;14:251-72. [Crossref] [PubMed]
- Hetzel M, Hetzel J, Schumann C, et al. Cryorecanalization: a new approach for the immediate management of acute airway obstruction. J Thorac Cardiovasc Surg 2004;127:1427-31. [Crossref] [PubMed]
- Schumann C, Hetzel M, Babiak AJ, et al. Endobronchial tumor debulking with a flexible cryoprobe for immediate treatment of malignant stenosis. J Thorac Cardiovasc Surg 2010;139:997-1000. [Crossref] [PubMed]
- Franke KJ, Szyrach M, Nilius G, et al. Experimental study on biopsy sampling using new flexible cryoprobes: influence of activation time, probe size, tissue consistency, and contact pressure of the probe on the size of the biopsy specimen. Lung 2009;187:253-9. [Crossref] [PubMed]
- Browning R, Parrish S, Sarkar S, et al. First report of a novel liquid nitrogen adjustable flow spray cryotherapy (SCT) device in the bronchoscopic treatment of disease of the central tracheo-bronchial airways. J Thorac Dis 2013;5:E103-6. [PubMed]
- DiBardino DM, Lanfranco AR, Haas AR. Bronchoscopic Cryotherapy. Clinical Applications of the Cryoprobe, Cryospray, and Cryoadhesion. Ann Am Thorac Soc 2016;13:1405-15. [Crossref] [PubMed]
- Maiwand MO. The role of cryosurgery in palliation of tracheo-bronchial carcinoma. Eur J Cardiothorac Surg 1999;15:764-8. [Crossref] [PubMed]
- Moorjani N, Beeson JE, Evans JM, et al. Cryosurgery for the treatment of benign tracheo-bronchial lesions. Interact Cardiovasc Thorac Surg 2004;3:547-50. [Crossref] [PubMed]
- Colt HG, Murgu SD, Korst RJ, et al. Follow-up and surveillance of the patient with lung cancer after curative-intent therapy: Diagnosis and management of lung cancer, 3rd ed: American College of Chest Physicians evidence-based clinical practice guidelines. Chest 2013;143:e437S-54S.
- Marasso A, Gallo E, Massaglia GM, et al. Cryosurgery in bronchoscopic treatment of tracheobronchial stenosis. Indications, limits, personal experience. Chest 1993;103:472-4. [Crossref] [PubMed]
- Maiwand MO, Evans JM, Beeson JE. The application of cryosurgery in the treatment of lung cancer. Cryobiology 2004;48:55-61. [Crossref] [PubMed]
- Lunn W. Obstruction of the Central Airways: Evaluation and Management. In: Simoff M, Sterman D, Ernst A. editors. Thoracic Endoscopy: Advances in Interventional Pulmonology. Hoboken: Wiley-Blackwell, 2008:323-9.
- Dumon JF. Seven-Year Experience with the Dumon Prosthesis. J Bronchology Interv Pulmonol 1996;3:6-10.
- Wood DE, Liu YH, Vallières E, et al. Airway stenting for malignant and benign tracheobronchial stenosis. Ann Thorac Surg 2003;76:167-72; discussion 173-4. [Crossref] [PubMed]
- Lemaire A, Burfeind WR, Toloza E, et al. Outcomes of tracheobronchial stents in patients with malignant airway disease. Ann Thorac Surg 2005;80:434-7; discussion 7-8. [Crossref] [PubMed]
- Ost DE, Shah AM, Lei X, et al. Respiratory infections increase the risk of granulation tissue formation following airway stenting in patients with malignant airway obstruction. Chest 2012;141:1473-81. [Crossref] [PubMed]
- Hohenforst-Schmidt W, Zarogoulidis P, Pitsiou G, et al. Drug Eluting Stents for Malignant Airway Obstruction: A Critical Review of the Literature. J Cancer 2016;7:377-90. [Crossref] [PubMed]
- Li L, Huh KM. Polymeric nanocarrier systems for photodynamic therapy. Biomater Res 2014;18:19. [Crossref] [PubMed]
- Chiaviello A, Postiglione I, Palumbo G. Targets and mechanisms of photodynamic therapy in lung cancer cells: a brief overview. Cancers (Basel) 2011;3:1014-41. [Crossref] [PubMed]
- Maziak DE, Markman BR, MacKay JA, et al. Photodynamic therapy in nonsmall cell lung cancer: a systematic review. Ann Thorac Surg 2004;77:1484-91. [Crossref] [PubMed]
- Lam S, Kostashuk EC, Coy EP, et al. A randomized comparative study of the safety and efficacy of photodynamic therapy using Photofrin II combined with palliative radiotherapy versus palliative radiotherapy alone in patients with inoperable obstructive non-small cell bronchogenic carcinoma. Photochem Photobiol 1987;46:893-7. [Crossref] [PubMed]
- Wieman TJ, Mang TS, Fingar VH, et al. Effect of photodynamic therapy on blood flow in normal and tumor vessels. Surgery 1988;104:512-7. [PubMed]
- Triesscheijn M, Baas P, Schellens JH, et al. Photodynamic therapy in oncology. Oncologist 2006;11:1034-44. [Crossref] [PubMed]
- Slawson RG, Scott RM. Radiation therapy in bronchogenic carcinoma. Radiology 1979;132:175-6. [Crossref] [PubMed]
- Celebioglu B, Gurkan OU, Erdogan S, et al. High dose rate endobronchial brachytherapy effectively palliates symptoms due to inoperable lung cancer. Jpn J Clin Oncol 2002;32:443-8. [Crossref] [PubMed]
- Kelly JF, Delclos ME, Morice RC, et al. High-dose-rate endobronchial brachytherapy effectively palliates symptoms due to airway tumors: the 10-year M. D. Anderson cancer center experience. Int J Radiat Oncol Biol Phys 2000;48:697-702. [Crossref] [PubMed]
- Huber RM, Fischer R, Haŭtmann H, et al. Palliative endobronchial brachytherapy for central lung tumors. A prospective, randomized comparison of two fractionation schedules. Chest 1995;107:463-70. [Crossref] [PubMed]
- Schray MF, McDougall JC, Martinez A, et al. Management of malignant airway compromise with laser and low dose rate brachytherapy. The Mayo Clinic experience. Chest 1988;93:264-9. [Crossref] [PubMed]
- Nori D, Allison R, Kaplan B, et al. High dose-rate intraluminal irradiation in bronchogenic carcinoma. Technique and results. Chest 1993;104:1006-11. [Crossref] [PubMed]
- Khanavkar B, Stern P, Alberti W, et al. Complications associated with brachytherapy alone or with laser in lung cancer. Chest 1991;99:1062-5. [Crossref] [PubMed]
- Trédaniel J, Hennequin C, Zalcman G, et al. Prolonged survival after high-dose rate endobronchial radiation for malignant airway obstruction. Chest 1994;105:767-72. [Crossref] [PubMed]