Lung densitometry: why, how and when
Definition
Lung densitometry, namely measurement of lung density, is based on the property of the pulmonary tissue to variably attenuate the X-rays and entails an array of technological and methodological issues aimed to make such a measurement as accurate and precise as possible.
Although it can be applied to lung specimens (1), lung densitometry is far more commonly performed in the living subjects. In the latter case, since chest wall and mediastinal structures interfere with such a measurement, lung densitometry is exclusive domain of computed tomography (CT) and reflects both degree of inflation and structural abnormalities of the lungs. Structural abnormalities can imply decreased attenuation, as typically occurs in emphysema or cystic lung diseases, or increased attenuation, as in pulmonary fibrosis. In this review we shall deal with in vivo lung densitometry in humans.
While lung densitometry is based on density value of each pulmonary voxel alone, texture analysis also considers the spatial relationships among density values of adjacent voxels (2). For this fundamental difference and its relevant background and methodological implications an in depth account of texture analysis is beyond our scope and will not be given.
Why to use lung densitometry?
Qualitative visual evaluation of the axial and reconstructed coronal or sagittal CT sections is the base for detection and classification of the type of lung structural abnormalities underlying increased or decreased pulmonary density. In the clinical practice this can be supplemented by computation of visual scales for semi-quantitative rating of the extent or severity of the diffuse lung alterations associated with decreased or increased lung density (3-13).
Qualitative visual assessment of the lung must always be performed before densitometry (“eye-first” rule), since it is fundamental for diagnostic purposes in the single patient and decreases the risk of false interpretation of the lung density values (14). However, lung densitometry can replace semi-quantitative visual rating of severity and extension of lung changes.
In fact, lung densitometry has five advantages over visual semi-quantitative assessment of diffuse lung alterations.
First, visual assessment is subjective and in general shows slight to moderate inter-observer reproducibility for emphysema (15), pulmonary fibrosis including honeycombing (16-18) and lymphangioleiomyomatosis (LAM) (3), as well in the assessment of air trapping due to small airways disease (9).
In particular, assessment of the extent of pulmonary emphysema in whole lung CT using a visual scale by four chest radiologists (two defined as experts having previously performed more than 500 visual scoring of emphysema on CT examinations) showed a slight to moderate (Cohen k value ranging from 0.16 to 0.41 for pairs of raters) inter-operator and a fair to moderate (Cohen k value ranging from 0.39 to 0.47) intra-operator reproducibility (15). Notably, inter- and intra-operator variability increased with the visual score magnitude, namely with increase of emphysema severity (15). On the contrary, no difference between expert and non-expert chest radiologists’ agreement was observed.
As well, agreement with a reference standard of the score for honeycombing presence by 43 observers, including radiologists with various subspeciality, chest physicians and chest radiologists, was fair to moderate (Cohen weighted k value ranging from 0.40, for radiologists, to 0.58, for chest radiologists) and not significantly different among the categories of observers (18). Similar results were obtained in another study that involved 18 readers with heterogeneous background and reported better agreement for honeycombing (average Cohen kappa value =0.44) and disease extent (average Cohen kappa value =0.47) than for traction bronchiectasis (average Cohen kappa =0.24) with no differences among general radiologists, thoracic radiologists, respiratory physicians, and radiology residents (16).
Direct comparison of CT densitometry with visual score in patients with emphysema (19,20), pulmonary fibrosis (21) and LAM (3) indicated that the former is more reproducible (the imperfect reproducibility of densitometry being due to variability in the manual correction of segmentation). Notably, the better reproducibility of CT densitometry could intrinsically afford a more sensitive assessment of diffuse lung changes than visual semi-quantitative assessment. In fact, it can be assumed that the lower the variability of the measurement, the smaller the variation which can reliably reflect a change of the condition being evaluated.
Second, lung densitometry enables an automatic or semi-automatic complete, namely without spatial gaps, evaluation of all (typically 300–350 according to the subject’s size) thin (1 mm thick) contiguous or overlapped sections of the whole lung parenchyma that are now in few seconds obtained in a single breath-hold with multi-detector CT scanners and spiral technology. In contrast, due to the time required, the reader usually performs visual semi-quantitative assessment on a discrete, typically one every ten sections (15,20,21) and not all CT sections, Notably, the skip of the majority of the sections (and of the volume) of the lung implies a substantial sampling bias of visual assessment that might be detrimental especially in longitudinal studies (22).
Third, thanks to the automatic or semiautomatic segmentation of lung tissue and to the negligible software computation time of its density, the time required for lung densitometry is generally shorter as compared to visual scoring.
Fourth, lung density measurements in an inspiratory scan show a better correlation than visual assessment with pathology quantification of pulmonary emphysema (19,23). So far, this comparative evaluation has not been performed in other diffuse lung diseases including LAM and pulmonary fibrosis.
Fifth, as shown in Table 1, lung density measurements generally correlate with results of pulmonary function tests (PFT) (including spirometry, determination of static lung volumes and lung diffusing capacity for carbon monoxide), dyspnea and quality of life measurements and the multi-parametric BODE index in patients with chronic obstructive pulmonary disease (COPD) (7,20,24-33,47), with results of PFT in smokers and smokers at risk of emphysema (34-37), with results of PFT in patients with LAM (3,38) and with results of PFT, exercise testing, and quality of life measurements in patients with lung fibrosis due to systemic sclerosis (21,39,40). Not surprisingly, because of the intrinsically continuous nature of the densitometry indexes shared with PFT, as opposed to the categorical nature of visual scores, in some studies of the above conditions the degree of correlation of densitometric parameters exceeded that of visual semi-quantitative rating (20,21). However, the degree of correlation of lung density measurements with PFT in idiopathic pulmonary fibrosis (IPF) is generally lower and similar to that of visual score (41-46).
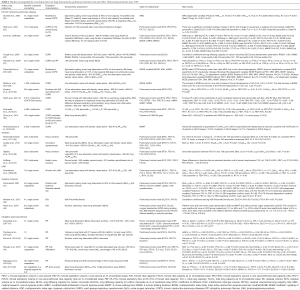
Full table
Spirometry and PFT are quantitative and reproducible techniques that are cheaper than CT which, in addition, utilizes potentially harmful ionizing radiations. However, PFT provide a global assessment of the lung damage. The regional information provided by CT, especially if assessed quantitatively with densitometry, along with its capability to differentiate and to monitor the components underlying obstructive, cystic, or fibrotic lung disease is a distinct advantage (14).
How to perform lung densitometry?
Lung densitometry is a quantitative technique and, as such, requires an accurate setting of parameters and a strict conduct in both CT scan acquisition and post-processing.
The Radiological Society of North America has promoted standardization of lung densitometry for COPD within the frame of Quantitative Imaging Biomarkers Alliance® (QIBA®).
The main technical and methodological aspects, crucial for carrying out a reliable analysis, are listed in Table 2. In the following sections, we will detail the image post-processing procedures for carrying out lung densitometry and discuss the main factors that influence such measurements.
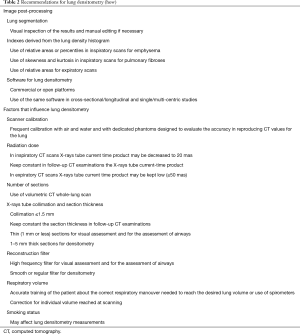
Full table
Image post-processing for defining indexes of emphysema or fibrosis extent
Lung segmentation
The 3-D lung segmentation is an image post-processing procedure that is preliminary to the computation of lung densitometry indexes. It is generally fully automatic and based on a combination of both morphological operations and intensity-based methods. A visual inspection of the results of the segmentation procedure (i.e., the lung mask) is mandatory and, in case of failure, a manual correction is essential in order to prevent the inclusion in the lung mask of non-lung regions or the exclusion of lung regions, e.g., because of image artefacts. Lung densitometry can be performed on a circumscribed lung area (48,49), but evaluation of diffuse lung diseases is usually performed in inspiratory scans on the entire cross-sectional area of the lung in selected sections or on the whole lung. In the latter case, once the lung mask is considered valid, the lung volume can be computed. This can be used to check the quality of the respiratory maneuver performed by the patient, enables application of volume correction techniques before densitometry measurements (50-54), but also represents a valuable information per se in many diffuse lung diseases, especially in longitudinal studies (14).
Indexes derived from the lung density histogram
Mean lung attenuation (MLA) is the simplest measurement which is utilized especially for pulmonary fibrosis (21,40-44,46), but also for estimation of emphysema extent (24,26,28,32,35). MLA is not a sensitive index and other histogram-based indexes are typically used for quantification with densitometry of emphysema and pulmonary fibrosis. Emphysema is assessed mainly with relative areas (RAs) and percentiles (PI), whereas fibrosis are assessed mainly with skewness and kurtosis (Figures 1-3).
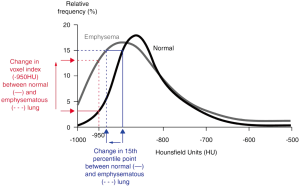
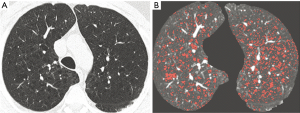
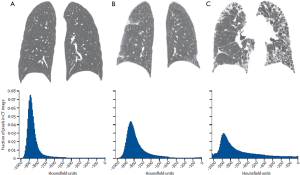
RA is defined as the percentage of the lung with density values below a given threshold (Figures 1,2). Thus, when emphysema extent increases, the RA value increases as well. For pulmonary emphysema, in inspiratory scans, RA at −970 (RA970) (23), −960 (RA960) (23), −950 (RA950) (19) (Figure 2) and −910 (RA910) (57) Hounsfield units (HU) significantly correlated with microscopic and/or macroscopic measurements of pulmonary emphysema and are currently utilized in clinical studies (14).
The percentile index (Perc) is defined as the value in HU below which that given percentage of all voxels is distributed (Figure 1). When emphysema extent increases, the density histogram shifts towards lower HU values and the given Perc index decreases. Several percentile indexes in inspiratory scans significantly correlated with microscopic and macroscopic measurements of emphysema, with Perc at 1% (Perc1) showing the strongest correlation (23,58). For pulmonary emphysema, Perc1 and Perc at 15% (Perc15) are commonly used indexes (14). In particular, Perc15 is less affected by image noise and truncation effect as compared to Perc1, can be volume-corrected using a physiological sponge model (59) and has been utilized in longitudinal studies of emphysema (25,55,60-62).
Skewness and kurtosis of the density histogram have been employed for quantifying pulmonary fibrosis (4,21,63-66). Skewness is a measure of the lack of symmetry of the density histogram, whereas kurtosis is a measure to which the distribution is peaked relative to a normal distribution. In lung fibrosis, both skewness and kurtosis typically decrease (Figure 3).
Other indexes derived from the histogram of lung density and/or the size of the low attenuation areas (LAA) have also been proposed for inspiratory scans including the bullae index (67), the specific gas volume, i.e., the volume of gas per weight of lung tissue (68), as well as fractal dimension of the cumulative distribution function of LAA clusters size (69).
For expiratory scans, thresholds of −856 HU (RA856) or −850 HU (RA850), corresponding to the attenuation values of a normal lung inflated by air (7), have been proposed in order to identify areas of air trapping to be distinguished from areas of emphysema (see below) (14).
Software for lung densitometry
Although several commercial software is available for lung densitometry, several open platforms have been developed as well, making access and use of lung densitometry relatively easy and potentially free. Software generally provides separate density measurements for left and right lungs and lobes. Unfortunately, a detailed description of lung segmentation methods is not available for all software, hindering a methodological comparison among them.
Available software includes Airway Inspector based on 3D SLICER for both the morphometric analysis of airways and lung parenchyma (70), Apollo (VIDA Diagnostics Inc., Coralville, Iowa, USA), CALIPER (Computer-Aided Lung Informatics for Pathology Evaluation and Ratings) (71), Imbio (Minneapolis, MN, USA), LowATT tool in Aquarius software (TeraRecon, Foster City, California, USA), MeVis PULMO3D (Fraunhofer MeVis Research, Bremen, Germany) (72), Osirix MD (Pixmeo, Geneva, Switzerland), PASS (Pulmonary Analysis Software Suite) (73), Pulmo3D tool in Syngo software (Siemens Healthcare, Germany) and YACTA (Yet another CT analyzer) (74).
A comparison among five of these fully automatic software showed that the inter-software variability of the failure rate in CT image processing and of RA950 were high (75). This poor inter-software reproducibility indicates that the same software for lung densitometry should be employed in cross-sectional/longitudinal and single/multi-centric studies.
Factors that influence lung densitometry
An accurate quality control should be applied to all steps of lung densitometry, from CT acquisition to image post-processing, including scanner calibration, adherence to recommended acquisition and reconstruction CT protocol, visual evaluation of image quality (absence of artifacts, check of fully coverage of the lung within the field of view, etc.) and of the lung mask, and assessment of the level of inspiration or expiration reached by the subject at scanning.
Scanner calibration
Scanner calibration plays a crucial role in assuring reliable density values in quantitative CT. Beyond periodic quality controls performed by CT scanner manufacturer, more frequent calibration procedures with air and water and with dedicated phantoms designed to evaluate the accuracy in reproducing CT values for the lung are recommended (76). In particular, in multi-centric studies, the quality of different CT scanners and their stability over time is essential. To this aim, a procedure for quality control in longitudinal studies using a dedicated foam phantom able to simulate CT density of lung emphysema has been proposed (54).
Radiation dose
Radiation dose is directly related to the mA setting of the CT scanner X-rays tube (77) and higher dose is associated with better signal to noise ratio. Higher dose is beneficial for visual assessment of diffuse lung diseases and is mandatory for small airway assessment, because multiplanar reconstructions obtained from submillimeter sections are necessary to ensure precise cross-sectional measurements (14).
Dose comparatively affects less the results of lung densitometry (58,66), although the increased image noise at lower CT doses may cause relative increase in threshold-based measures of emphysema (14). As a matter of fact, the CT radiation dose in inspiratory CT scans for emphysema may be decreased to values of tube current-time product as low as 20 mAs without any significant compromise of lung density assessment (58). It is however recommended to keep constant the tube current-time product (and section thickness) in follow-up CT examinations (78).
In the clinical practice low dose scanning is recommended for assessment of air trapping in expiratory CT scans, and in such a case the tube current-time product may also be ≤50 mAs (14).
Typically, low dose whole lung CT scans at end inspiration as those utilized in lung cancer screening are associated to radiation doses below 1 mSv, whereas full radiation dose radiation whole lung CT scans at end inspiration require several units of mSv (78).
The effects on lung densitometry of additional options to contain radiation dose including iterative reconstruction algorithms and automatic tube-current modulation according to the body size have yet to be established (14).
Number of sections
With the CT units available until early ‘80 that employed a sequential scanning technology it was possible to acquire only one section for each breath-hold. Consequently, lung densitometry was initially performed on few, typically three, sections at predefined anatomic levels or one every ten sections from the apex to the base of the lung. With the advent of multi-detector spiral CT units, whole lung volume is acquired in a few seconds and automatic or semi-automatic segmentation makes whole-lung volume and densitometry readily available (14).
Interestingly, densitometry assessment of lung emphysema in a small volume acquired on the lower lung fields during CT scanning for evaluation of coronary artery disease has been reported to provide a reproducible and valid assessment of emphysema (79). This may be important taking into consideration that emphysema and coronary artery disease are co-morbidities related to smoking habitude.
X-rays tube collimation and section thickness
The X-rays tube collimation, the reconstruction parameters including section thickness and matrix and the reconstruction filter are major determinants of spatial resolution in a CT image. A range of collimation and section reconstructions between 0.75 and 10 mm were used in CT acquisitions for lung densitometry, but now the collimation is 1.5 mm or below. Section thickness has a significant effect on lung densitometry in the case of pulmonary emphysema evaluated in inspiratory scans with thicker sections implying underestimation (58,80). This fact has an important consequence: data obtained with different section thickness cannot be merged in cross-sectional or longitudinal studies (58,80).
Reconstruction filter
The type of reconstruction filter has a marked effect on lung densitometry results in the case of emphysema (80,81). In particular, the high frequency (“high resolution” or “edge enhancement”) reconstruction filters that are generally applied for visual assessment of structural changes of the lung on CT lead to a significant shift of pulmonary density values, implying an overestimation of emphysema (80). Hence, the reconstruction filters should be kept constant in both cross-sectional and longitudinal studies.
Currently, a double reconstruction of the raw data obtained with volumetric thin collimation acquisitions is feasible and recommended: (I) thin (1 mm or less) sections with high resolution filter for visual assessment and for the assessment of airways; (II) 1–5 mm thick sections with smooth or regular filters for densitometry (14,20).
Alternatively, the application of a Gaussian smoothing to reduce the noise in sections reconstructed using high resolution filter has also been employed (50,51).
Respiratory volume
Lung densitometry is usually performed on a CT scan obtained at end inspiration. Notably, lung volume at end inspiration is most reproducible as compared to volumes attained in expiration, either at forced respiratory capacity (FRC) or at residual volume (RV), corresponding to end expiration and forced expiration, respectively (14,82).
In principle, to get rid of possible confounding effects of lung volumes on lung density values, two approaches can be followed: (I) an accurate coaching of the patient by the radiographer about the correct respiratory manouver needed to reach the desired lung volume (83) or (II) the use of spirometers (with or without CT gating) for real-time measurement of the lung volume (21,24,64,76). However, spirometers are not used in the clinical practice.
Due to the sensitivity of pulmonary density to lung volume (Figure 4) (56), correction for individual volume reached at scanning has been established as a fundamental methodological requisite in cross-sectional and longitudinal densitometry assessment of emphysema in inspiratory scans (50-54).
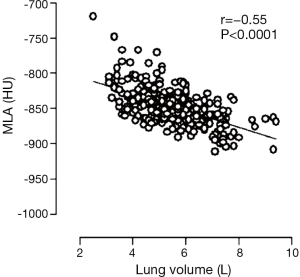
In selected cases (see below) lung densitometry can also be applied on a CT scan obtained at end or forced expiration for assessment of areas of air trapping.
Notably, patients with severe COPD and hyper-inflated lungs can have variable difficulty in reaching desired expiratory volumes. This represents a strong confounding variable for the assessment of lung density in expiratory scans in these patients.
Smoking status
Intriguingly, lung density measurements may also be affected by the smoking status of the subjects. In fact, current smoking status per se, presumably because of soot and tar deposition or inflammation, can determine increased lung density irrespective of emphysema presence and evolution (61,84).
When to perform lung densitometry?
Indications of lung densitometry are summarized in Table 3.
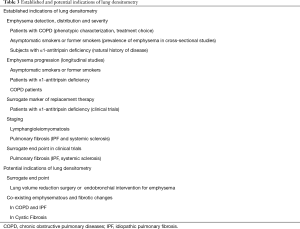
Full table
In principle, due to the reasons previously outlined, lung densitometry might substitute visual semi-quantitative scales in all instances in which they are applied.
However, visual semi-quantitative scales have been for a long time the standard in the clinical practice and “conservatism” (and some possible individual reluctance towards numbers) might militate against adoption of lung densitometry.
On the other hand, there is growing agreement that lung densitometry is useful in pathophysiology research and is to be preferred as endpoint in pharmacological or surgical trials (Table 4) (22,85). In particular, 25 of 94 studies using CT in COPD patients and registered at ClinicalTrials.gov (https://clinicaltrials.gov), as accessed on march 24, 2017, specifically mentioned adoption of quantification of lung features including density, volume or texture analysis. The same applies to 9 of 75 studies using CT in pulmonary fibrosis.
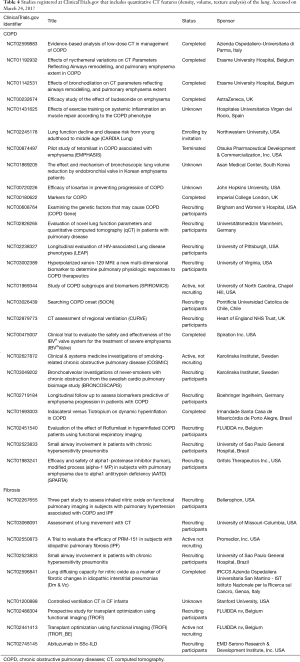
Full table
Some Authors suggest a complementary role of visual semi-quantitative scales and lung densitometry (6,14).
Lung densitometry has predominantly been used for assessment of emphysema, whereas it has been comparatively less applied to the evaluation to lung fibrosis. Recently, the possible role of lung densitometry in fibrotic interstitial lung diseases in clinical practice and treatment trials has been addressed in a position paper of the Fleischner Society (6).
Measurement of emphysema and air trapping for phenotypic characterization and choice of treatment in the patient with COPD
Quantification with PFT of airflow limitation, in particular a post-bronchodilator FEV1/FVC ratio <70% (86), is fundamental to diagnose and grade COPD in patients with dyspnea, cough, and/or sputum production (87). According to the 2017 GOLD recommendations, in the diagnostic phase of COPD, chest X-ray and CT are useful only to rule out other conditions contributing to respiratory symptoms or in patients failing to respond to treatment (87).
However, with such an approach, under the COPD label are comprised different combinations in the single patient of two pathological and clinical conditions, namely emphysema and chronic bronchitis, for which the need of a different therapeutic strategy is advocated by some Authors (88-92) and already recommended in case of severe COPD (see below) (87).
In particular, schematically, the pathophysiological basis of expiratory airflow obstruction measured by PFT is represented by inflammatory changes and remodelling of the conductive airways (first 14–15 generations of bronchi) in COPD patients with chronic bronchitis phenotype, and by destruction of the lung parenchyma where gas exchange takes place in COPD patients with emphysema phenotype (88). It has been argued that discarding this heterogeneity might account for the deceiving results in pharmacological trials that recruited COPD patients based on the results of spirometry alone (93). Moreover, only patients with CT evidence of emphysema at upper lobes and decreased exercise capacity had benefit from lung volume reduction surgery (LVRS) (see below) (94). The above evidence in someone’s opinion supports the view that future therapeutic strategies should be targeted on the COPD patient’s specific pathophysiological bases of expiratory air flow obstruction (95).
Presently, visual assessment of lung density in an inspiratory CT scan is considered by the GOLD initiative Authors to be sufficient for selection of treatment options in patients with advanced COPD (87). These include surgery (bullectomy), LVRS or endobronchial procedures to treat patients showing large bullae and heterogenous or homogeneous emphysema with hyperinflation, and lung transplantation to treat patients without the above CT findings. However, the capability of lung densitometry to more objectively assess the amount of pulmonary emphysema before treatment choice is established (25). In particular, Perc15 was applied to assessment of effect of LVRS for emphysema (25). The surgical procedure significantly increased lung density by 5.0±10.9 g/L. Improvement in the diffusing capacity of the lung for carbon monoxide and in RV significantly correlated with an increase in lung density, supporting the value of Perc15 as a surrogate marker for detection of both the extent and reduction of emphysema. A similar application can be anticipated for assessment of efficacy of bronchial valve placement in COPD patients and is under investigation (Table 4).
In addition, software for automatic morphometric analysis of the airway luminal diameter and airway wall thickness until to bronchi with 3.5 mm external diameter (third or fourth sub-segmentary division) on CT are now available and enable direct measurement of the chronic bronchitis component (30,96). The quantitative assessment in a single inspiratory CT scan of emphysema and chronic bronchitis (Figure 5) contributing to the overall airway obstruction demonstrated and quantified by PFT, is potentially of paramount pathophysiological, clinical and therapeutic interest (30,36,96).
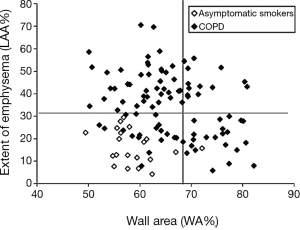
Importantly, lung densitometry using RA856 or RA850 may be used to assess gas air trapping in expiratory scans which is assumed to reflect obstruction of the more distal airways that may be prevalent in single patients and is considered by some Authors as the primary momentum of emphysema development (97). Although, overall, densitometry of air trapping is less standardized as compared to densitometry of emphysema in inspiratory scans, especially due to the variable degree of expiratory volume reached by the subject, it is now recognized that expiratory scans might contribute to identify in the single patient gas trapping as a third determinant of airflow obstruction (14,31).
Additional composite volume and density measurements can be obtained from paired inspiratory/expiratory scans (98).
From the above it emerges that using densitometry and small airway assessment it is possible to obtain a complete phenotype of the single COPD patient with measurement of the respective contribute of emphysema and airway changes (in inspiratory scan) and as trapping (in expiratory scan) to the overall airway obstruction shown by spirometry.
Ascertainment of pauci or a-symptomatic emphysema in the single subject and studies of prevalence and progression of pulmonary emphysema
Emphysema is associated predominantly with cigarette smoking but also with fire (cooking) smoke, inhalative substances (occupational exposure) and air pollution (87).
Chronic respiratory symptoms associated with acute respiratory events may precede the development of airflow limitation measurable with spirometry (99). In addition, also smokers without airflow limitation on spirometry may have structural evidence of lung disease manifested by the presence of emphysema, airway wall thickening, and gas trapping (99,100).
Since, detection of mild emphysema is difficult on chest X-rays (14), lung CT has become the preferred tool to establish presence and severity of pulmonary emphysema in the single subject with no or mild symptoms and in cohorts of subjects at variable risk defined according to different criteria, typically smoking history and age (34,37,56,101). For such a purpose, visual score and densitometry can be applied. For both approaches, it is necessary to establish the threshold for presence of “significant” emphysema. Accordingly, emphysematous areas extending from 5% to 25% of the lung cross-sectional area can qualify presence of mild emphysema on visual assessment (51,102). Similarly, a threshold of 6% value of the average RA950 in an inspiratory scan was recommended for densitometry definition of presence “significant” of emphysema (103,104).
Prevalence of “significant” emphysema in healthy smokers and former-smokers, as measured with lung densitometry and different RA values, ranged between 26% and 58% (56,101). On the other hand, the median percent emphysema defined as the percentage of lung voxels below −950 HU in 854 healthy never-smokers was 1.1%, but it was higher among men compared with women and lower among African Americans, Hispanics, and Asians compared with whites (37). Moreover, percent emphysema was positively related to age and height and inversely related to body mass index.
A potentially confounding factor for the above estimates is ageing that is characterised by lung changes which are apparently similar to those of emphysema (105). However, they are associated to distal airspace enlargement rather than the alveolar wall destruction associated to emphysema. Zach et al. (106) reported that CT attenuation remains relatively similar within the age range from 45 to 80 years in 92 healthy non-smokers. Moreover, according to Bellia et al. (107), differentiation between senile lung and pulmonary emphysema is possible using lung densitometry and the usual density threshold. However, Copley et al. (35) observed that both MLD, RA950 and RA910 were correlated with age in non-smoking healthy volunteers, whereas fractal dimension, an index of tissue complexity extracted with texture analysis, was significantly higher in younger subjects as compared to elderly subjects.
CT follow-up for emphysema is not routinely done nor recommended by the Respiratory Societies and there is paucity of information about the factors associated with progression of pulmonary emphysema or lack of thereof. Hence there is interest in measuring progression of emphysema in asymptomatic smokers and former smokers or COPD patients as well as in subjects with alpha-1-antitrypsin (AAT) deficiency.
Lung densitometry has been applied to investigate progression of smoking-related emphysema in subjects recruited in lung cancer screening studies with low dose CT (51,108,109). Unfortunately, due to heterogeneity of the lung densitometry procedure, data from these studies are rather fragmentary. Bellomi et al. (108) reported that in the COSMOS study the median percentage increase in emphysema over a 2-year period was significantly higher in current than in former smokers and that the risk of worsening emphysema (by 30% in 2 years) in current smokers increased with smoking duration. In the ITALUNG study (51) 15 (14.5%) of 103 subjects showed a Perc15 corrected for lung volume change between the 2 examinations that exceeded the lower 95% limit of agreement, consistent with progression of emphysema with a mean difference in lung density of 14.7%±2.6%. In the NELSON trial 3,670 male smokers underwent low dose CT at baseline and after 1 and 3 years follow-up (109). At baseline, mean lung volume <−950 HU (%) was 8.8 and mean perc15 was −935 HU. Former smokers had an annual rate of progression of emphysema of 1.07%, compared with 1.12% in current smokers. The progression rate was greater in those with more severe COPD at baseline.
In a longitudinal study outside screening, Coxson et al. (84) found an average annual decline in lung density of 1.13 g/L after correction for lung volume in a group of 1,928 current and former smokers. The decline was more rapid in women than in men, and in current smokers than in former smokers.
As mentioned above, history of smoking and variation of smoking habits should be considered in cross-sectional and longitudinal studies of emphysema defined by CT lung density.
AAT deficiency is an uncommon hereditary condition characterized by development of pulmonary emphysema that is typically distributed to the lung bases and is due to deficiency of this glycoprotein that is mainly synthesized in the liver and protects lung tissue from destruction by neutrophil elastase. Because of the assumed improved sensitivity as compared to spirometry in revealing early and small alterations in lung parenchyma, since 1992 CT has been used to evaluate lung disease in AAT-deficient individuals (110). Importantly, lung densitometry was the best independent predictor of mortality in AAT deficiency (111). In a sample of 32-year-old asymptomatic subjects with AAT-deficiency of varying severity discovered at neonatal screening no lung density change consistent with emphysema was measured as compared to age-matched control subjects (112). These data are in line with the observation that AAT deficit determines clinically detectable emphysema and COPD in the affected subjects after 40 years of age (113). In a cohort of 22 patients with moderate emphysema due to marked AAT deficiency who were followed for 2–4 years with an annual CT, lung densitometry demonstrated a marked decline in HU in low-density areas, corresponding to a mean annual loss of lung tissue of 2.1 g/L lung volume (114). Bakker et al. (62) assessed with densitometry regional progression of emphysema in 50 subjects with emphysema due to AAT deficiency and 16 subjects with smoking related emphysema who underwent CT at baseline and after 30 months. As expected, in AAT deficiency subjects, emphysema was predominantly distributed basally and progression was found to be more pronounced in the basal area consistent with the hypothesis that emphysema due to AAT deficiency spreads out from affected areas. This was not the case in subjects with smoking-related emphysema.
Surrogate markers of replacement therapy in AAT deficiency emphysema
Replacement therapy of AAT deficiency has been introduced since ‘80 (115). The assumption was that by augmenting the circulating and hence lung levels of AAT, the normal inhibitory capacity of AAT in the lungs would be restored with retardation of the destructive process.
Since AAT deficiency is a rare disease, recruitment of the large number of subjects required for a controlled trial using PFT as end-point is cumbersome. Hence, lung densitometry has been included as surrogate marker and primary end-point in two multi-center randomized trials investigating efficacy of AAT replacement therapy (60,116). Pooled analysis of the two trials (85) indicated that after a mean follow-up of approximately 2.5 years, a mean change in lung density from baseline to last CT scan of −4.0 g/L for AAT and of −6.3 g/L for placebo was observed with a treatment related statistically significant difference. These results demonstrated that intravenous AAT augmentation therapy significantly reduces the decline in lung density and may have an impact on the mortality in patients with AAT deficiency related emphysema.
LAM
Densitometry has been applied to quantification of lesion burden in LAM using two approaches. Initially, a double (−300 and −900 HU) tresholding technique proved capable to separate abnormal tissue in LAM patients from control subjects and demonstrated correlation between the quantitative CT index and both PFT and exercise measurements (117). Measurement of the volume of the cystic changes as percentage of total lung volume by means of segmentation and computation of RA910 and Perc15 demonstrated strongest correlation with PFT as compared to each densitometric index alone (38).
In LAM patients, texture analysis demonstrated existence of damage also in tissue nearby cysts and this improved the correlation with lung function. These data indicate that cystic changes alone may not define the extent of lung destruction in this disease (118).
Staging and end point in clinical trials of pulmonary fibrosis
One general problem in patients with different form of lung fibrosis is staging of the disease at clinical presentation. This has relevance both on the prognosis of the single patient and on the opportunity to selectively enrol in clinical trials those subjects who are likely to have a progression of pulmonary fibrosis (6,21).
Staging is usually performed with a combination of clinical, PFT, histopathological, biomarkers and CT features. The latter are usually determined with visual scales for qualitative or semi-quantitative assessment of reticular pattern, traction bronchiectasis, ground glass opacities and honeycombing. Lung densitometry on whole lung (46), selected sections (42) or on regions of interest with creation of local histograms of lung density distribution (46,48,49) (Figure 6) has been developed and tested for staging IPF. Both lung densitometry and visual semi-quantitative scales were capable to predict mortality, but kurtosis alone was identified as a significant predictor of mortality on multivariate analysis in a study (42). Two of these studies also demonstrated the capability of lung densitometry to track progression of pulmonary fibrosis (4,49). However, in a study of 57 patients with IPF, one classifier-model-derived score (Quantitative Lung Fibrosis), based on a set of texture features, but not histogram kurtosis was associated with baseline functional disease severity and was also a sensitive measure of change over a 7 months interval in terms of forced vital capacity (FVC) and carbon monoxide diffusion capacity (43).
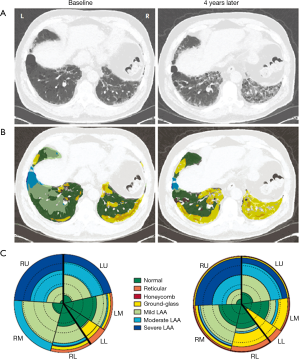
Similarly, lung densitometry proved to be able to track progression of lung fibrosis in a cohort of patients with systemic sclerosis (39).
Importantly, as indicated in Table 4, lung densitometry of serial CT has been incorporated along with other markers as end point in clinical trials of new drugs for IPF (6) and of new therapeutic interventions as autologous stem cell transplantation for pulmonary fibrosis in systemic sclerosis (119). In these contexts, taking into account the inherent longitudinal character of the trials, the overcome of subjectivity of visual rating afforded by lung densitometry and its increased sensitivity appear particularly advantageous.
Additional potential indications of densitometry
Lung densitometry in the single patient might have a role for assessment of the fibrotic component that is associated to emphysema in up to 10% COPD patients (120) and vice versa of the emphysema that can be observed in patients with cystic fibrosis (121) and with IPF (122).
Limitations of lung densitometry
The following limitations of lung densitometry can be recognized.
As any precision measurement, lung densitometry is prone to systematic errors. It is dependent on several variables pertinent to the acquisition and processing of CT data and on heterogeneity of software. Software can have some costs and undergo technological turn-over.
Finally, lung densitometry is based on exposition to ionizing radiations that may be harmful especially in the context of longitudinal assessment.
Perspectives of lung densitometry
Normal values of lung densitometry
In analogy to PFT, using the recommendations reported above, reasonable standardization of lung densitometry can now be achieved. Despite some attempts to define range of normal lung density values (27,106,123), a decisive step for implementation in the daily clinical practice of lung densitometry would be availability of shared databases with normal values of lung density and greater consensus about the indexes of choice for detection and tracking of emphysema and other diffuse lung diseases (124).
Simulated phantoms of emphysema
An attempt has been proposed to model lung parenchyma by mimicking the cluster size distribution of LAA on CT imaging of subjects with pulmonary emphysema (69,124-127). In particular, a set of 40 digital phantoms of LAA images in CT imaging with different grade of emphysema severity has been generated using a finite element model (125). Both RA and the exponent D of the cumulative distribution function of LAA clusters size (69) computed on the digital phantoms were underestimated as compared to those calculated on the models output (which simulates the parenchymal tissue and thus can be considered as reference), suggesting that the accuracy in the assessment of real emphysema extent of such indexes may be low. The generation of larger databases of standard test images and also the design of physical phantoms of LAA images are desirable to evaluate accuracy and compare results among densitometry indexes. These analyses may be complementary to validation with histology data.
Voxel-wise inspiratory and expiratory joint analysis
Recently, joint analyses of inspiratory and expiratory CT scans allowed to extract functional information from CT images. For this purpose, Murphy et al. proposed a quantification of voxel-wise ventilation measurements in automatically spatially aligned inspiration-to-expiration CT sections (128). Several CT-derived measurements showed high correlation (coefficients between 0.85 and 0.90) with spirometry results in a sample of 216 patients with COPD. Also, using these CT-derived measurements, a k-nearest neighbors’ classifier correctly anticipated the GOLD stage in 67% of subjects.
Another technique, originally developed for neuroimaging applications, termed parametric response map (PRM), has been proposed to quantify regional air trapping and emphysema (129,130). This technique applies a voxel-wise approach to the usual thresholds for emphysema index (RA950 in inspiratory scans) and air trapping (RA856 in expiratory scans) on co-registered inspiratory and expiration images. The joint thresholding classifies individual voxels of lung parenchyma as normal, non-emphysematous airflow obstruction (functional small airways disease) and emphysema. The result of this analysis is displayed as a color coded map representing the voxel-wise labels (Figure 7) as well as the percentage of voxels belonging to each class. Such information may be used for studying the regional (e.g., cranio-caudal) distribution of air trapping/emphysema, their possible interplay and progression over time and their correlation with PFTs and clinical data. Using PRM Boes et al. (131) were capable to document a 1-year progression of functional small airways disease (air trapping areas) in 11 of 76 patients with COPD, whereas emphysema areas increased only slightly (from 19 to 21%). These results indicate the capability of PRM to monitor disease status and support the view that small airways disease may be a transitional phase from normal parenchyma to emphysema.
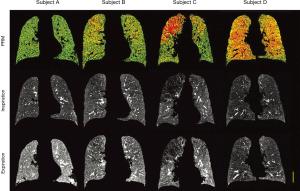
Conclusions
Lung densitometry is not a “stand-alone” quantitative procedure and must always be performed after visual (“qualitative”) assessment of the morphological or density changes of the pulmonary tissue in the CT sections on which it is applied (“eye-first” rule). Lung densitometry is less operator-dependent and faster than visual semi-quantitative scores and enables a complete assessment of extension and severity of diffuse pulmonary structural abnormalities implying decreased or increased pulmonary density. In case of inspiratory scans, it is also better correlated with pathologically measured extension of emphysema. Finally, it provides better or equal correlation with PFT. Commercially and open platform software are available making access and use of lung densitometry relatively easy and potentially free. Correction for lung volumes on lung density measurement is mandatory. Quality control on the CT scanner and adherence to technical and methodological recommendations are fundamental.
The main indication for lung densitometry in the clinical setting is measurement of emphysema component in an inspiratory scan of the single patient with COPD. Additional emerging applications of lung densitometry include the evaluation of air trapping in COPD patients and in subjects at risk of emphysema and the staging in patients with IPF and in patients with LAM. Lung densitometry has been applied in pathophysiology research to assess prevalence of smoking related emphysema and to monitor progression of smoking-related emphysema, AAT deficiency emphysema, IPF and systemic sclerosis pulmonary fibrosis. Finally, it is used in patients undergoing surgical or bronchoscopic treatment of emphysema and is recommended as efficacy end-point or surrogate marker in trials replacement therapy in AAT deficiency emphysema and lung fibrosis in IPF and systemic sclerosis.
Acknowledgements
The authors wish to thank Maurizio Zompatori and Fabio Falaschi for constructive criticisms.
Funding: This work was supported by the Italian Ministry of Health, by Tuscany Region (Italy) (RF-2010-2321362) and by Ente Cassa di Risparmio di Firenze (2013.0464).
Footnote
Conflicts of Interest: The authors have no conflicts of interest to declare.
References
- Scotton CJ, Hayes B, Alexander R, et al. Ex vivo micro-computed tomography analysis of bleomycin-induced lung fibrosis for preclinical drug evaluation. Eur Respir J 2013;42:1633-45. [Crossref] [PubMed]
- Ginsburg SB, Lynch DA, Bowler RP, et al. Automated texture-based quantification of centrilobular nodularity and centrilobular emphysema in chest CT images. Acad Radiol 2012;19:1241-51. [Crossref] [PubMed]
- Avila NA, Kelly JA, Dwyer AJ, et al. Lymphangioleiomyomatosis: correlation of qualitative and quantitative thin-section CT with pulmonary function tests and assessment of dependence on pleurodesis. Radiology 2002;223:189-97. [Crossref] [PubMed]
- Best AC, Meng J, Lynch AM, et al. Idiopathic pulmonary fibrosis: physiologic tests, quantitative CT indexes, and CT visual scores as predictors of mortality. Radiology 2008;246:935-40. [Crossref] [PubMed]
- Goddard PR, Nicholson EM, Laszlo G, et al. Computed tomography in pulmonary emphysema. Clin Radiol 1982;33:379-87. [Crossref] [PubMed]
- Hansell DM, Goldin JG, King TE Jr, et al. CT staging and monitoring of fibrotic interstitial lung diseases in clinical practice and treatment trials: a position paper from the Fleischner Society. Lancet Respir Med 2015;3:483-96. [Crossref] [PubMed]
- Hersh CP, Washko GR, Estépar RS, et al. Paired inspiratory-expiratory chest CT scans to assess for small airways disease in COPD. Respir Res 2013;14:42. [Crossref] [PubMed]
- Lee JS, Im JG, Ahn JM, et al. Fibrosing alveolitis: prognostic implication of ground-glass attenuation at high-resolution CT. Radiology 1992;184:451-4. [Crossref] [PubMed]
- Ng CS, Desai SR, Rubens MB, et al. Visual quantitation and observer variation of signs of small airways disease at inspiratory and expiratory CT. J Thorac Imaging 1999;14:279-85. [Crossref] [PubMed]
- Sakai F, Gamsu G, Im JG, et al. Pulmonary function abnormalities in patients with CT-determined emphysema. J Comput Assist Tomogr 1987;11:963-8. [Crossref] [PubMed]
- Schmidt SL, Sundaram B, Flaherty KR. Diagnosing fibrotic lung disease: when is high-resolution computed tomography sufficient to make a diagnosis of idiopathic pulmonary fibrosis? Respirology 2009;14:934-9. [Crossref] [PubMed]
- Shin KM, Lee KS, Chung MP, et al. Prognostic determinants among clinical, thin-section CT, and histopathologic findings for fibrotic idiopathic interstitial pneumonias: tertiary hospital study. Radiology 2008;249:328-37. [Crossref] [PubMed]
- Warrick JH, Bhalla M, Schabel SI, et al. High resolution computed tomography in early scleroderma lung disease. J Rheumatol 1991;18:1520-8. [PubMed]
- Lynch DA. Progress in Imaging COPD, 2004-2014. Chronic Obstr Pulm Dis 2014;1:73-82. [Crossref] [PubMed]
- Mascalchi M, Diciotti S, Sverzellati N, et al. Low agreement of visual rating for detailed quantification of pulmonary emphysema in whole-lung CT. Acta Radiol 2012;53:53-60. [Crossref] [PubMed]
- Siemienowicz ML, Kruger SJ, Goh NS, et al. Agreement and mortality prediction in high-resolution CT of diffuse fibrotic lung disease. J Med Imaging Radiat Oncol 2015;59:555-63. [Crossref] [PubMed]
- Walsh SL, Sverzellati N, Devaraj A, et al. Connective tissue disease related fibrotic lung disease: high resolution computed tomographic and pulmonary function indices as prognostic determinants. Thorax 2014;69:216-22. [Crossref] [PubMed]
- Watadani T, Sakai F, Johkoh T, et al. Interobserver variability in the CT assessment of honeycombing in the lungs. Radiology 2013;266:936-44. [Crossref] [PubMed]
- Bankier AA, De Maertelaer V, Keyzer C, et al. Pulmonary emphysema: subjective visual grading versus objective quantification with macroscopic morphometry and thin-section CT densitometry. Radiology 1999;211:851-8. [Crossref] [PubMed]
- Cavigli E, Camiciottoli G, Diciotti S, et al. Whole-lung densitometry versus visual assessment of emphysema. Eur Radiol 2009;19:1686-92. [Crossref] [PubMed]
- Camiciottoli G, Orlandi I, Bartolucci M, et al. Lung CT densitometry in systemic sclerosis: correlation with lung function, exercise testing, and quality of life. Chest 2007;131:672-81. [Crossref] [PubMed]
- Goldin JG. Computed tomography as a biomarker in clinical trials imaging. J Thorac Imaging 2013;28:291-7. [Crossref] [PubMed]
- Madani A, Zanen J, de Maertelaer V, et al. Pulmonary emphysema: objective quantification at multi-detector row CT--comparison with macroscopic and microscopic morphometry. Radiology 2006;238:1036-43. [Crossref] [PubMed]
- Giuntini C, Camiciottoli G, Maluccio NM, et al. Chronic effort dyspnea explained by lung function tests and by HRCT and CRX radiographic patterns in COPD: a post-hoc analysis in 51 patients. COPD 2007;4:169-76. [Crossref] [PubMed]
- Stolk J, Versteegh MI, Montenij LJ, et al. Densitometry for assessment of effect of lung volume reduction surgery for emphysema. Eur Respir J 2007;29:1138-43. [Crossref] [PubMed]
- Lee YK, Oh YM, Lee JH, et al. Quantitative assessment of emphysema, air trapping, and airway thickening on computed tomography. Lung 2008;186:157-65. [Crossref] [PubMed]
- Heussel CP, Herth FJ, Kappes J, et al. Fully automatic quantitative assessment of emphysema in computed tomography: comparison with pulmonary function testing and normal values. Eur Radiol 2009;19:2391-402. [Crossref] [PubMed]
- Camiciottoli G, Bigazzi F, Bartolucci M, et al. BODE-index, modified BODE-index and ADO-score in chronic obstructive pulmonary disease: relationship with COPD phenotypes and CT lung density changes. COPD 2012;9:297-304. [Crossref] [PubMed]
- Martinez CH, Chen YH, Westgate PM, et al. Relationship between quantitative CT metrics and health status and BODE in chronic obstructive pulmonary disease. Thorax 2012;67:399-406. [Crossref] [PubMed]
- Koyama H, Ohno Y, Nishio M, et al. Three-dimensional airway lumen volumetry: comparison with bronchial wall area and parenchymal densitometry in assessment of airway obstruction in pulmonary emphysema. Br J Radiol 2012;85:1525-32. [Crossref] [PubMed]
- Schroeder JD, McKenzie AS, Zach JA, et al. Relationships between airflow obstruction and quantitative CT measurements of emphysema, air trapping, and airways in subjects with and without chronic obstructive pulmonary disease. AJR Am J Roentgenol 2013;201:W460-70. [Crossref] [PubMed]
- Choo JY, Lee KY, Shin C, et al. Quantitative analysis of lungs and airways with CT in subjects with the chronic obstructive pulmonary disease (COPD) candidate FAM13A gene: case control study for CT quantification in COPD risk gene. J Comput Assist Tomogr 2014;38:597-603. [Crossref] [PubMed]
- Andrianopoulos V, Celli BR, Franssen FM, et al. Determinants of exercise-induced oxygen desaturation including pulmonary emphysema in COPD: Results from the ECLIPSE study. Respir Med 2016;119:87-95. [Crossref] [PubMed]
- Mohamed Hoesein FA, Zanen P, van Ginneken B, et al. Association of the transfer coefficient of the lung for carbon monoxide with emphysema progression in male smokers. Eur Respir J 2011;38:1012-8. [Crossref] [PubMed]
- Copley SJ, Giannarou S, Schmid VJ, et al. Effect of aging on lung structure in vivo: assessment with densitometric and fractal analysis of high-resolution computed tomography data. J Thorac Imaging 2012;27:366-71. [Crossref] [PubMed]
- Dijkstra AE, Postma DS, ten Hacken N, et al. Low-dose CT measurements of airway dimensions and emphysema associated with airflow limitation in heavy smokers: a cross sectional study. Respir Res 2013;14:11. [Crossref] [PubMed]
- Hoffman EA, Ahmed FS, Baumhauer H, et al. Variation in the percent of emphysema-like lung in a healthy, nonsmoking multiethnic sample. The MESA lung study. Ann Am Thorac Soc 2014;11:898-907. [Crossref] [PubMed]
- Schmithorst VJ, Altes TA, Young LR, et al. Automated algorithm for quantifying the extent of cystic change on volumetric chest CT: initial results in Lymphangioleiomyomatosis. AJR Am J Roentgenol 2009;192:1037-44. [Crossref] [PubMed]
- Ninaber MK, Stolk J, Smit J, et al. Lung structure and function relation in systemic sclerosis: application of lung densitometry. Eur J Radiol 2015;84:975-9. [Crossref] [PubMed]
- Ariani A, Aiello M, Silva M, et al. Quantitative CT indexes are significantly associated with exercise oxygen desaturation in interstitial lung disease related to systemic sclerosis. Clin Respir J 2016. [Epub ahead of print]. [Crossref] [PubMed]
- Sverzellati N, Calabrò E, Chetta A, et al. Visual score and quantitative CT indices in pulmonary fibrosis: Relationship with physiologic impairment. Radiol Med 2007;112:1160-72. [Crossref] [PubMed]
- Tanizawa K, Handa T, Nagai S, et al. Clinical impact of high-attenuation and cystic areas on computed tomography in fibrotic idiopathic interstitial pneumonias. BMC Pulm Med 2015;15:74. [Crossref] [PubMed]
- Kim HJ, Brown MS, Chong D, et al. Comparison of the quantitative CT imaging biomarkers of idiopathic pulmonary fibrosis at baseline and early change with an interval of 7 months. Acad Radiol 2015;22:70-80. [Crossref] [PubMed]
- Ohkubo H, Kanemitsu Y, Uemura T, et al. Normal Lung Quantification in Usual Interstitial Pneumonia Pattern: The Impact of Threshold-based Volumetric CT Analysis for the Staging of Idiopathic Pulmonary Fibrosis. PLoS One 2016;11:e0152505. [Crossref] [PubMed]
- Nakagawa H, Nagatani Y, Takahashi M, et al. Quantitative CT analysis of honeycombing area in idiopathic pulmonary fibrosis: Correlations with pulmonary function tests. Eur J Radiol 2016;85:125-30. [Crossref] [PubMed]
- Ash SY, Harmouche R, Vallejo DL, et al. Densitometric and local histogram based analysis of computed tomography images in patients with idiopathic pulmonary fibrosis. Respir Res 2017;18:45. [Crossref] [PubMed]
- Camiciottoli G, Bartolucci M, Maluccio NM, et al. Spirometrically gated high-resolution CT findings in COPD: lung attenuation vs lung function and dyspnea severity. Chest 2006;129:558-64. [Crossref] [PubMed]
- Iwasawa T, Asakura A, Sakai F, et al. Assessment of prognosis of patients with idiopathic pulmonary fibrosis by computer-aided analysis of CT images. J Thorac Imaging 2009;24:216-22. [Crossref] [PubMed]
- Maldonado F, Moua T, Rajagopalan S, et al. Automated quantification of radiological patterns predicts survival in idiopathic pulmonary fibrosis. Eur Respir J 2014;43:204-12. [Crossref] [PubMed]
- Diciotti S, Sverzellati N, Kauczor HU, et al. Defining the intra-subject variability of whole-lung CT densitometry in two lung cancer screening trials. Acad Radiol 2011;18:1403-11. [Crossref] [PubMed]
- Mascalchi M, Sverzellati N, Falchini M, et al. Changes in volume-corrected whole-lung density in smokers and former smokers during the ITALUNG screening trial. J Thorac Imaging 2012;27:255-62. [Crossref] [PubMed]
- Park SJ, Lee CH, Goo JM, et al. Inter-scan repeatability of CT-based lung densitometry in the surveillance of emphysema in a lung cancer screening setting. Eur J Radiol 2012;81:e554-60. [Crossref] [PubMed]
- Shaker SB, Dirksen A, Laursen LC, et al. Volume adjustment of lung density by computed tomography scans in patients with emphysema. Acta Radiol 2004;45:417-23. [Crossref] [PubMed]
- Stoel BC, Putter H, Bakker ME, et al. Volume correction in computed tomography densitometry for follow-up studies on pulmonary emphysema. Proc Am Thorac Soc 2008;5:919-24. [Crossref] [PubMed]
- Parr DG, Dirksen A, Piitulainen E, et al. Exploring the optimum approach to the use of CT densitometry in a randomised placebo-controlled study of augmentation therapy in alpha 1-antitrypsin deficiency. Respir Res 2009;10:75. [Crossref] [PubMed]
- Camiciottoli G, Cavigli E, Grassi L, et al. Prevalence and correlates of pulmonary emphysema in smokers and former smokers. A densitometric study of participants in the ITALUNG trial. Eur Radiol 2009;19:58-66. [Crossref] [PubMed]
- Müller NL, Staples CA, Miller RR, et al. "Density mask". An objective method to quantitate emphysema using computed tomography. Chest 1988;94:782-7. [PubMed]
- Madani A, De Maertelaer V, Zanen J, et al. Pulmonary emphysema: radiation dose and section thickness at multidetector CT quantification--comparison with macroscopic and microscopic morphometry. Radiology 2007;243:250-7. [Crossref] [PubMed]
- Dirksen A. Monitoring the progress of emphysema by repeat computed tomography scans with focus on noise reduction. Proc Am Thorac Soc 2008;5:925-8. [Crossref] [PubMed]
- Dirksen A, Dijkman JH, Madsen F, et al. A randomized clinical trial of alpha(1)-antitrypsin augmentation therapy. Am J Respir Crit Care Med 1999;160:1468-72. [Crossref] [PubMed]
- Ashraf H, Lo P, Shaker SB, et al. Short-term effect of changes in smoking behaviour on emphysema quantification by CT. Thorax 2011;66:55-60. [Crossref] [PubMed]
- Bakker ME, Putter H, Stolk J, et al. Assessment of regional progression of pulmonary emphysema with CT densitometry. Chest 2008;134:931-7. [Crossref] [PubMed]
- Best AC, Lynch AM, Bozic CM, et al. Quantitative CT indexes in idiopathic pulmonary fibrosis: relationship with physiologic impairment. Radiology 2003;228:407-14. [Crossref] [PubMed]
- Camiciottoli G, Diciotti S, Bartolucci M, et al. Whole-lung volume and density in spirometrically-gated inspiratory and expiratory CT in systemic sclerosis: correlation with static volumes at pulmonary function tests. Sarcoidosis Vasc Diffuse Lung Dis 2013;30:17-27. [PubMed]
- Hartley PG, Galvin JR, Hunninghake GW, et al. High-resolution CT-derived measures of lung density are valid indexes of interstitial lung disease. J Appl Physiol (1985) 1994;76:271-7. [PubMed]
- Sverzellati N, Zompatori M, De Luca G, et al. Evaluation of quantitative CT indexes in idiopathic interstitial pneumonitis using a low-dose technique. Eur J Radiol 2005;56:370-5. [Crossref] [PubMed]
- Blechschmidt RA, Werthschützky R, Lörcher U. Automated CT image evaluation of the lung: a morphology-based concept. IEEE Trans Med Imaging 2001;20:434-42. [Crossref] [PubMed]
- Coxson HO, Rogers RM, Whittall KP, et al. A quantification of the lung surface area in emphysema using computed tomography. Am J Respir Crit Care Med 1999;159:851-6. [Crossref] [PubMed]
- Mishima M, Hirai T, Itoh H, et al. Complexity of terminal airspace geometry assessed by lung computed tomography in normal subjects and patients with chronic obstructive pulmonary disease. Proc Natl Acad Sci U S A 1999;96:8829-34. [Crossref] [PubMed]
- San Jose Estépar R, Washko GG, Silverman EK, et al. Airway Inspector: An Open Source Application for Lung Morphometry. First International Workshop on Pulmonary Image ProcessingFirst International Workshop on Pulmonary Image Processing. New York 2008;293-302.
- Zavaletta VA, Bartholmai BJ, Robb RA. High resolution multidetector CT-aided tissue analysis and quantification of lung fibrosis. Acad Radiol 2007;14:772-87. [Crossref] [PubMed]
- Kuhnigk JM, Dicken V, Zidowitz S, et al. Informatics in radiology (infoRAD): new tools for computer assistance in thoracic CT. Part 1. Functional analysis of lungs, lung lobes, and bronchopulmonary segments. Radiographics 2005;25:525-36. [Crossref] [PubMed]
- Guo J, Reinhardt JM, Kitaoka H, et al. Integrated system for CT-based assessment of parenchymal lung disease. In 2002 International Symposium on Biomedical Imaging 2002;871-4.
- Zaporozhan J, Ley S, Eberhardt R, et al. Paired inspiratory/expiratory volumetric thin-slice CT scan for emphysema analysis: comparison of different quantitative evaluations and pulmonary function test. Chest 2005;128:3212-20. [Crossref] [PubMed]
- Wielpütz MO, Bardarova D, Weinheimer O, et al. Variation of densitometry on computed tomography in COPD--influence of different software tools. PLoS One 2014;9:e112898. [Crossref] [PubMed]
- Newell JD Jr, Hogg JC, Snider GL. Report of a workshop: quantitative computed tomography scanning in longitudinal studies of emphysema. Eur Respir J 2004;23:769-75. [Crossref] [PubMed]
- Mayo JR, Aldrich J, Muller NL, et al. Radiation exposure at chest CT: a statement of the Fleischner Society. Radiology 2003;228:15-21. [Crossref] [PubMed]
- Mascalchi M, Belli G, Zappa M, et al. Risk-benefit analysis of X-ray exposure associated with lung cancer screening in the Italung-CT trial. AJR Am J Roentgenol 2006;187:421-9. [Crossref] [PubMed]
- Hoffman EA, Jiang R, Baumhauer H, et al. Reproducibility and validity of lung density measures from cardiac CT Scans--The Multi-Ethnic Study of Atherosclerosis (MESA) Lung Study. Acad Radiol 2009;16:689-99. [Crossref] [PubMed]
- Gierada DS, Bierhals AJ, Choong CK, et al. Effects of CT section thickness and reconstruction kernel on emphysema quantification relationship to the magnitude of the CT emphysema index. Acad Radiol 2010;17:146-56. [Crossref] [PubMed]
- Boedeker KL, McNitt-Gray MF, Rogers SR, et al. Emphysema: effect of reconstruction algorithm on CT imaging measures. Radiology 2004;232:295-301. [Crossref] [PubMed]
- Lamers RJ, Kemerink GJ, Drent M, et al. Reproducibility of spirometrically controlled CT lung densitometry in a clinical setting. Eur Respir J 1998;11:942-5. [Crossref] [PubMed]
- Bankier AA, O'Donnell CR, Boiselle PM. Quality initiatives. Respiratory instructions for CT examinations of the lungs: a hands-on guide. Radiographics 2008;28:919-31. [Crossref] [PubMed]
- Coxson HO, Dirksen A, Edwards LD, et al. The presence and progression of emphysema in COPD as determined by CT scanning and biomarker expression: a prospective analysis from the ECLIPSE study. Lancet Respir Med 2013;1:129-36. [Crossref] [PubMed]
- Stockley RA, Parr DG, Piitulainen E, et al. Therapeutic efficacy of alpha-1 antitrypsin augmentation therapy on the loss of lung tissue: an integrated analysis of 2 randomised clinical trials using computed tomography densitometry. Respir Res 2010;11:136. [Crossref] [PubMed]
- Rabe KF, Hurd S, Anzueto A, et al. Global strategy for the diagnosis, management, and prevention of chronic obstructive pulmonary disease: GOLD executive summary. Am J Respir Crit Care Med 2007;176:532-55. [Crossref] [PubMed]
- Vogelmeier CF, Criner GJ, Martinez FJ, et al. Global Strategy for the Diagnosis, Management, and Prevention of Chronic Obstructive Lung Disease 2017 Report. GOLD Executive Summary. Am J Respir Crit Care Med 2017;195:557-82. [Crossref] [PubMed]
- Burrows B, Fletcher CM, Heard BE, et al. The emphysematous and bronchial types of chronic airways obstruction. A clinicopathological study of patients in London and Chicago. Lancet 1966;1:830-5. [Crossref] [PubMed]
- Gelb AF, Hogg JC, Müller NL, et al. Contribution of emphysema and small airways in COPD. Chest 1996;109:353-9. [Crossref] [PubMed]
- Pistolesi M, Camiciottoli G, Paoletti M, et al. Identification of a predominant COPD phenotype in clinical practice. Respir Med 2008;102:367-76. [Crossref] [PubMed]
- Reilly JJ. COPD and declining FEV1--time to divide and conquer? N Engl J Med 2008;359:1616-8. [Crossref] [PubMed]
- Wedzicha JA. The heterogeneity of chronic obstructive pulmonary disease. Thorax 2000;55:631-2. [Crossref] [PubMed]
- Calverley PM, Rabe KF, Goehring UM, et al. Roflumilast in symptomatic chronic obstructive pulmonary disease: two randomised clinical trials. Lancet 2009;374:685-94. [Crossref] [PubMed]
- Fishman A, Martinez F, Naunheim K, et al. A randomized trial comparing lung-volume-reduction surgery with medical therapy for severe emphysema. N Engl J Med 2003;348:2059-73. [Crossref] [PubMed]
- Hogg JC. Pathophysiology of airflow limitation in chronic obstructive pulmonary disease. Lancet 2004;364:709-21. [Crossref] [PubMed]
- Nakano Y, Müller NL, King GG, et al. Quantitative assessment of airway remodeling using high-resolution CT. Chest 2002;122:271S-275S. [Crossref] [PubMed]
- Hogg JC, McDonough JE, Sanchez PG, et al. Micro-computed tomography measurements of peripheral lung pathology in chronic obstructive pulmonary disease. Proc Am Thorac Soc 2009;6:546-9. [Crossref] [PubMed]
- Nagatani Y, Murata K, Takahashi M, et al. A new quantitative index of lobar air trapping in chronic obstructive pulmonary disease (COPD): comparison with conventional methods. Eur J Radiol 2015;84:963-74. [Crossref] [PubMed]
- Woodruff PG, Barr RG, Bleecker E, et al. Clinical Significance of Symptoms in Smokers with Preserved Pulmonary Function. N Engl J Med 2016;374:1811-21. [Crossref] [PubMed]
- Regan EA, Lynch DA, Curran-Everett D, et al. Clinical and Radiologic Disease in Smokers With Normal Spirometry. JAMA Intern Med 2015;175:1539-49. [Crossref] [PubMed]
- Sashidhar K, Gulati M, Gupta D, et al. Emphysema in heavy smokers with normal chest radiography. Detection and quantification by HCRT. Acta Radiol 2002;43:60-5. [PubMed]
- Hersh CP, Washko GR, Jacobson FL, et al. Interobserver variability in the determination of upper lobe-predominant emphysema. Chest 2007;131:424-31. [Crossref] [PubMed]
- Gevenois PA, De Vuyst P, de Maertelaer V, et al. Comparison of computed density and microscopic morphometry in pulmonary emphysema. Am J Respir Crit Care Med 1996;154:187-92. [Crossref] [PubMed]
- Lynch DA, Austin JH, Hogg JC, et al. CT-Definable Subtypes of Chronic Obstructive Pulmonary Disease: A Statement of the Fleischner Society. Radiology 2015;277:192-205. [Crossref] [PubMed]
- Fukuchi Y. The aging lung and chronic obstructive pulmonary disease: similarity and difference. Proc Am Thorac Soc 2009;6:570-2. [Crossref] [PubMed]
- Zach JA, Newell JD Jr, Schroeder J, et al. Quantitative computed tomography of the lungs and airways in healthy nonsmoking adults. Invest Radiol 2012;47:596-602. [Crossref] [PubMed]
- Bellia M, Benfante A, Menozzii M, et al. Validation of lung densitometry threshold at CT for the distinction between senile lung and emphysema in elderly subjects. Monaldi Arch Chest Dis 2011;75:162-6. [PubMed]
- Bellomi M, Rampinelli C, Veronesi G, et al. Evolution of emphysema in relation to smoking. Eur Radiol 2010;20:286-92. [Crossref] [PubMed]
- Mohamed Hoesein FA, Zanen P, de Jong PA, et al. Rate of progression of CT-quantified emphysema in male current and ex-smokers: a follow-up study. Respir Res 2013;14:55. [Crossref] [PubMed]
- Guest PJ, Hansell DM. High resolution computed tomography (HRCT) in emphysema associated with alpha-1-antitrypsin deficiency. Clin Radiol 1992;45:260-6. [Crossref] [PubMed]
- Dawkins PA, Dowson LJ, Guest PJ, et al. Predictors of mortality in alpha1-antitrypsin deficiency. Thorax 2003;58:1020-6. [Crossref] [PubMed]
- Bernspång E, Diaz S, Stoel B, et al. CT lung densitometry in young adults with alpha-1-antitrypsin deficiency. Respir Med 2011;105:74-9. [Crossref] [PubMed]
- Janus ED, Phillips NT, Carrell RW. Smoking, lung function, and alpha 1-antitrypsin deficiency. Lancet 1985;1:152-4. [Crossref] [PubMed]
- Dirksen A, Friis M, Olesen KP, et al. Progress of emphysema in severe alpha 1-antitrypsin deficiency as assessed by annual CT. Acta Radiol 1997;38:826-32. [PubMed]
- Wewers MD, Casolaro MA, Sellers SE, et al. Replacement therapy for alpha 1-antitrypsin deficiency associated with emphysema. N Engl J Med 1987;316:1055-62. [Crossref] [PubMed]
- Dirksen A, Piitulainen E, Parr DG, et al. Exploring the role of CT densitometry: a randomised study of augmentation therapy in alpha1-antitrypsin deficiency. Eur Respir J 2009;33:1345-53. [Crossref] [PubMed]
- Crausman RS, Lynch DA, Mortenson RL, et al. Quantitative CT predicts the severity of physiologic dysfunction in patients with lymphangioleiomyomatosis. Chest 1996;109:131-7. [Crossref] [PubMed]
- Yao J, Taveira-DaSilva AM, Colby TV, et al. CT grading of lung disease in lymphangioleiomyomatosis. AJR Am J Roentgenol 2012;199:787-93. [Crossref] [PubMed]
- Kloth C, Maximilian Thaiss W, Preibsch H, et al. Quantitative chest CT analysis in patients with systemic sclerosis before and after autologous stem cell transplantation: comparison of results with those of pulmonary function tests and clinical tests. Rheumatology (Oxford) 2016;55:1763-70. [Crossref] [PubMed]
- Washko GR, Hunninghake GM, Fernandez IE, et al. Lung volumes and emphysema in smokers with interstitial lung abnormalities. N Engl J Med 2011;364:897-906. [Crossref] [PubMed]
- Wielpütz MO, Weinheimer O, Eichinger M, et al. Pulmonary emphysema in cystic fibrosis detected by densitometry on chest multidetector computed tomography. PLoS One 2013;8:e73142. [Crossref] [PubMed]
- Mejía M, Carrillo G, Rojas-Serrano J, et al. Idiopathic pulmonary fibrosis and emphysema: decreased survival associated with severe pulmonary arterial hypertension. Chest 2009;136:10-5. [Crossref] [PubMed]
- Smith BM, Barr RG. Establishing normal reference values in quantitative computed tomography of emphysema. J Thorac Imaging 2013;28:280-3. [Crossref] [PubMed]
- Stolk J, Stoel BC. Lung densitometry to assess progression of emphysema in chronic obstructive pulmonary disease: time to apply in the clinic? Am J Respir Crit Care Med 2011;183:1578-80. [Crossref] [PubMed]
- Diciotti S, Nobis A, Ciulli S, et al. Development of digital phantoms based on a finite element model to simulate low-attenuation areas in CT imaging for pulmonary emphysema quantification. Int J Comput Assist Radiol Surg 2016. [Epub ahead of print]. [PubMed]
- Suki B, Lutchen KR, Ingenito EP. On the progressive nature of emphysema: roles of proteases, inflammation, and mechanical forces. Am J Respir Crit Care Med 2003;168:516-21. [Crossref] [PubMed]
- Bates JH, Davis GS, Majumdar A, et al. Linking parenchymal disease progression to changes in lung mechanical function by percolation. Am J Respir Crit Care Med 2007;176:617-23. [Crossref] [PubMed]
- Murphy K, Pluim JP, van Rikxoort EM, et al. Toward automatic regional analysis of pulmonary function using inspiration and expiration thoracic CT. Med Phys 2012;39:1650-62. [Crossref] [PubMed]
- Boes JL, Bule M, Hoff BA, et al. The Impact of Sources of Variability on Parametric Response Mapping of Lung CT Scans. Tomography 2015;1:69-77. [Crossref] [PubMed]
- Galbán CJ, Han MK, Boes JL, et al. Computed tomography-based biomarker provides unique signature for diagnosis of COPD phenotypes and disease progression. Nat Med 2012;18:1711-5. [Crossref] [PubMed]
- Boes JL, Hoff BA, Bule M, et al. Parametric response mapping monitors temporal changes on lung CT scans in the subpopulations and intermediate outcome measures in COPD Study (SPIROMICS). Acad Radiol 2015;22:186-94. [Crossref] [PubMed]