Comparison of continuous compression with regular ventilations versus 30:2 compressions-ventilations strategy during mechanical cardiopulmonary resuscitation in a porcine model of cardiac arrest
Introduction
Appropriate compression-ventilation (C:V) ratio is an important component of cardiopulmonary resuscitation (CPR), which plays a major role in the chain of survival for cardiac arrest (CA) victims (1). Since 2005, a C:V ratio of 30:2 is recommended by the American Heart Association (AHA) for adult CPR without advanced airway, with the purpose of improving hemodynamics by decreasing “hands-off” time. Animal experiments and computer modeling have demonstrated that vital organ perfusion and resuscitation outcomes are superior with 30:2 ratio (2-5).
However, continuous chest compression (CCC) with or without ventilations was recently recommended by the AHA guidelines because of the improvement in resuscitation outcomes by minimizing interruption and encouraging more bystander CPR. Animal experiments and clinical studies have proven that resuscitation outcomes were similar or better in compression-only CPR when compared with conventional manual CPR (6-11). Furthermore, the latest guidelines also emphasize CCC combined with regular ventilations in CA patients when an advanced airway exists.
Currently, the ideal C:V ratio and the necessity of ventilation during CPR is still under question. However, little research has been developed to focus on this controversy during mechanical CPR. Previously, our group has demonstrated that CCC with miniaturized mechanical chest compressor combined with regular mechanical ventilations improved hemodynamic efficacy and the success of CPR, resulting in better post-resuscitation myocardial and neurological recovery (12). However, regulate ventilations during CCC with miniaturized mechanical chest compressor might increase the incidence of rib fractures or lung injury because of the asynchronism between compression and ventilation. Therefore, we investigated the effects of 30:2 mechanical CPR on the outcomes and incidence of CPR related complications when compared with CCC combined with regular ventilations in a porcine CA model.
Methods
The study was approved by the Institutional Animal Care and Use Committee of the Tang Wanchun Laboratories of Emergency & Critical Care Medicine at Sun Yat-sen Memorial Hospital, Sun Yat-sen University (IACUC-2016P1609).
Animal preparation
The study was performed on 16 male domestic pigs (weight, 39±2 kg). All animals were fasted overnight but free to water. Anaesthesia was induced with intramuscular injection of ketamine (20 mg/kg) and then followed by an intravascular injection of sodium pentobarbital (30 mg/kg). While the animals showed any signs of restlessness or awakened an additional dose of sodium pentobarbital (8 mg/kg) was administrated, or at intervals of 1 h to maintain anaesthesia if needed. The animals were intubated with a cuffed endotracheal tube and then mechanically ventilated with a VELA ventilator (CareFusion, California, US) at tidal volume of 10 mL/kg, peak flow of 35 L/min, and FiO2 of 0.21. End-tidal carbon dioxide (ETCO2) was monitored with the capnometer module of a BeneView T5 patient monitor (Mindray, Shenzhen, China). Regulation of respiratory frequency was according to maintain ETCO2 between 35–40 mmHg before CA. Three adhesive electrodes were used to the shaved skin of the proximal right-and-left, upper-and-lower limbs for recording electrocardiogram (ECG). A 6-F catheter was inserted into the thoracic aorta through the right femoral artery for the collection of blood samples and the measurement of aortic pressure (AP). For the measurements of core blood temperature and right atrial pressure (RAP), a 7-F Swan-GanzTM catheter (Edwards Lifesciences LLC, US) was advanced from the right femoral vein into the right atrium. For the measurement of extravascular lung water (EVLW) and cardiac output (CO), a 5-F thermistor-tipped catheter (PV-2014L16, Pulsion Medical Systems, Munich, Germany), connected to a PICCO® computer (Mindray, Ltd., Shenzhen, China), was advanced into the descending aorta via the left femoral artery. Saline containing 5 IU bovine heparin per mL were intermittently flushed into all catheters. A 5-F pacing catheter (EP Technologies Inc., Mountain View, CA) was placed through the right external jugular vein into the right ventricle for inducing ventricular fibrillation (VF). The positions of the catheters were confirmed by typical pressure morphology. For measurement of carotid blood flow (CBF), a flow probe (Ultrasonic Blood Flow Meter, T420, Transonic Systems Inc., Ithaca, NY) was positioned around the right common carotid artery. Hard gel adult defibrillation pads (stat-padz, Zoll Medical Corporation, Chelmsford, MA, USA) were applied in an anterior to lateral placement. The compressor piston was placed in the midcourt line at the level of the fifth interspace. Core temperature was adjusted to 37.5±0.5 °C with a warm/cooling blanket (HGT-200II, Hokai medical instruments corporation, Zhuhai, China) throughout the whole experiment.
Experimental procedures
Baseline measurements were obtained 15-min before inducing VF. The animals were then randomly assigned to receive CCC combined with regular ventilations (CCC group) or 30:2 CPR (VC group). VF was electrically induced with a 1mA alternating current through the pacing catheter. After the onset of VF, mechanical ventilation was discontinued. CPR was initiated after 7 min of untreated VF. The miniaturized mechanical chest compressor (Weil SCCTM, SunLife Inc., Shanghai, China) was set up to perform 100 compressions per minute. The compression depth was set to decrease the anterior-posterior diameter of the chest by 25%. At the same time of beginning of precordial compression, the animals were mechanically ventilated with a tidal volume of 10 mL/kg and FiO2 of 1.0 and a rate of 10 breaths per minute in the CCC group or 30:2 C:V in the VC group. Administration of epinephrine (20 µg/kg) was via the femoral vein after 2 min of CPR. Direct current shock (150 J biphasic energy) was delivered after 5 min of CPR by a Zoll E-Series defibrillator (Zoll Medical Corporation, Chelmsford, MA). If an organized rhythm with a mean aortic pressure (MAP) more than 50 mmHg maintaining for an interval of 5 min or more, the animal was regarded as the restoration of spontaneous circulation (ROSC). If failed to ROSC, CPR was instantly resumed for 2 min before the delivery of another single shock. A maximum of 5 cycles was repeated for the protocol. Additional doses of epinephrine were administrated every 3 min. If ROSC was not achieved, resuscitation protocol was terminated. Another 150-J countershock was attempted if recurrent VF occurred within 30 min after successful resuscitation. After ROSC, the observation was continued for 360 min. Animals were mechanically ventilated with FiO2 of 100% for the first 30 min, 50% for the next 30 min and 21% thereafter. For evaluation of the CPR-related injuries, bedside chest radiograph was performed at all the animals after ROSC. All catheters were withdrawn and wounds were surgically sutured after 360 min post-resuscitation. The animals were then back to the cages and monitored for an additional 72 h. At 24, 48 and 72 h post-resuscitation, neurological function was evaluated by two independent investigators based on the Neurological Alertness Score (NAS) (13). After 72 h of observation, the euthanasia of animals was administrated with 150 mg/kg pentobarbital.
Measurements
ECG, hemodynamic, CBF and ETCO2 were measured continuously and recorded on a PC-based data acquisition system supported by CODAS hardware/software (Computer Acquisition System, Cambridge, MA). Coronary perfusion pressure (CPP) was digitally computed from the differences in time-coincident diastolic AP and RAP and displayed in real time. Arterial blood gases were tested by a Radiometer ABL FLEX™ 80 (Radiometer, Copenhagen, Denmark) at baseline, VF 6 min, CPR 4 min and 30, 120 and 360 min post-resuscitation. Pulse index continuous cardiac output (PiCCO) measurements were assessed at baseline (BL), 30, 60, 120, 240 and 360 min after resuscitation.
Ten mL of 8 °C saline was injected through the central vein into the right atrium. CO and EVLW were obtained by transpulmonary thermodilution method (14). And EVLW was then divided by body weight to compute the extravascular lung water index (EVLWI). The measurement was repeated thrice to obtain a mean value.
Statistical analysis
Continuous variables were shown as mean ± SD if data was normally distributed. If data was not normally distributed, a median (25th, 75th percentiles) were shown. Normal distribution was confirmed by the Kolmogorov-Smirnov test. ANOVA with Scheffe’s method for multiple comparisons was used to analyse measurements between both groups. For the comparison of categorical variables such as rate of ROSC and survival, the Fisher’s exact test was used. A value of P<0.05 was regarded significant.
Results
Heart rate, MAP, RAP, ETCO2, CBF, arterial pH, PaO2, lactate, CO and EVLWI in baseline did not differ obviously between both groups (Figures 1,2, Table 1).
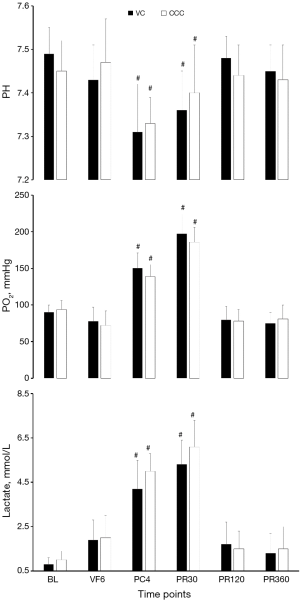
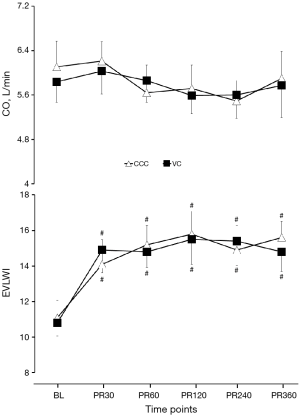
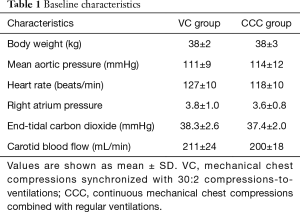
Full table
CPP, ETCO2 and CBF in the VC group was similar to those achieved in the CCC group during CPR (Figure 3). Similar compression depths were performed by the SCC in two groups, whereas chest compression fraction was lower in the VC group compared with the CCC group (Table 2).
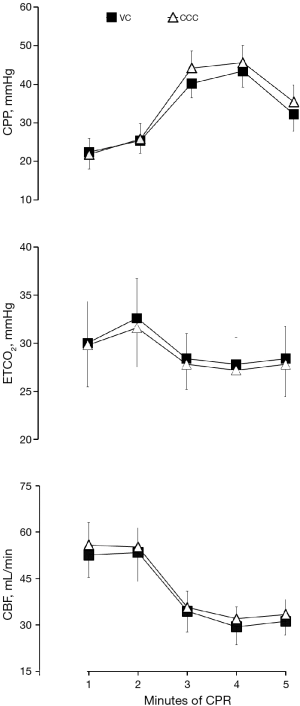
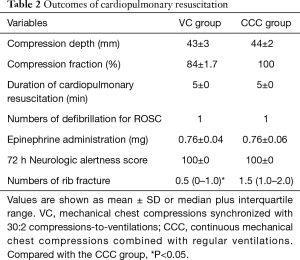
Full table
In comparison with baseline value, significant decrease in PH and increases in PO2 and lactate were observed in all the animals during CPR and at PR30. However, no obvious differences were observed in arterial blood gas parameters between both groups after 5 min of CPR and at 30, 120 and 360 min post-resuscitation (Figure 1). Although EVLWI of animals in both groups significantly increased after resuscitation, no distinct differences in CO and EVLWI were found in these two groups.
All animals were successfully resuscitated and survived for 72 h with favourable neurologic outcomes in these two groups. No obvious differences in the requirements of epinephrine doses, CPR duration and defibrillation were observed in both groups. However, significantly more numbers of rib fracture were found in CCC animals in comparison with VC animals (Table 2).
Discussion
The present study demonstrated that there were no differences in hemodynamic efficacy, gas exchanges and PiCCO measurements between 30:2 mechanical CPR and CCC combined with regular ventilations in a porcine model. Moreover, equivalent 72 h survival with good neurological function was observed in both groups. However, significant incidences of rib fractures were found in CCC group.
Chest compressions generate critical blood flow, oxygen and energy delivery to the heart and brain during CPR. For adequate vital tissue oxygenation, it is important to minimize interruptions and maximize the amount of chest compressions (1-13). Some experimental and clinical studies have indicated that better hemodynamic efficacy was achieved by the CCC strategy when compared to 30:2 CPR (11,15,16). However, the results of our study demonstrated that similar CPP, ETCO2, and CBF were achieved by CCC and 30:2 CPR. The reason for this difference may be because compression interruption for ventilation was about 4 s in our protocol whereas it was 16 s or more in the aforementioned experimental and clinical studies. Previous studies have demonstrated that the increasing interval of compression interruption compromises hemodynamic efficacy and therefore the outcome of CPR (17,18). Furthermore, with prolonged interruptions of compression, perfusion is non-existent for longer periods, as resumption time of chest compressions is increased due to a longer hands-off period (19).
Compression alone was recently indicated to cause profound hypoxemia, respiratory acidosis and significantly worse 24 h neurologic outcomes in comparison to CCC with regular ventilations in porcine models (20). A lack of positive pressure ventilation during CA and CPR obviously increases the amount of atelectasis, resulting in impairment of gas exchanges and hemodynamic once a critical amount of atelectasis is exceeded (21). However, in the present study, no significant differences in arterial gas changes were found between 30:2 CPR and CCC synchronized with regular ventilations. Consistent tidal volume and oxygen concentrations may be a rational explanation for similar results of blood gas analysis, even though different breaths were given every minute in each group (10 breaths in the CCC group vs. 4–6 breaths in the VC group).
In the present study, successful resuscitation of all animals in both groups resulted from a CPP greater than 15 mmHg during CPR, which regarded as the best predictor in the successful defibrillation and resuscitation. Furthermore, though chest compression fraction was larger in the CCC group when compared to the VC group (100% vs. 84%), both were more than 80%; which is associated with a high rate of ROSC (22). Additionally, identical survival rates with intact neurologic function in both groups were supported by favorable hemodynamic efficacy and CBF levels, which are indicators of myocardial and cerebral perfusion during CPR.
CCC with regular ventilations are suggested by the current AHA guidelines to perform on patients with an advanced airway, but 30:2 mechanical CPR should be taken into account based on the similar outcomes of the two different CPR strategies, as seen in this study. In addition, lower ventilation rates in 30:2 CPR could prevent hyperventilation, which may impede filling of the right heart and thereby limit CO generated by the compressions. Moreover, CPR related injuries including rib fracture or impairments in airway and lung during CPR due to positive ventilations in the phases of compression could be prevented in 30:2 CPR. In the present study, we demonstrated that the numbers of rib fracture in the CCC animals were obviously higher that in VC animals.
Limitations
There were several limitations in our study. Firstly, the healthy animals might not accord with the real condition of patients in a clinical setting. Successful resuscitation from CPR would not be easy to achieve unless the underlying cause is treated, such as revascularization of coronary occlusions. Secondly, interruption of chest compression for two breaths took 4 s in our protocol, less than the average time required by lay persons which was 13–16 s (16,23). However, it is possible to perform two breaths in 4 s with bag mask by skilful rescuers or synchronized with the ventilator during CPR. Finally, gasping was allowed during VF and CPR, which could generate not only ventilation but also forward blood flow which thereby may confound the hemodynamic efficacy and gas changes (24,25). However, both groups were treated identically to minimize the bias and gasping that occur in humans.
Conclusions
There were no differences in hemodynamic efficacy, gas exchanges, CO and EVLWI, therefore identical 72 h survival with intact neurologic function was observed between 30:2 mechanical CPR and CCC combined with regular ventilations. However, the incidence of rib fracture increases during the mechanical CPR strategy of CCC combined with regular ventilations.
Acknowledgements
Funding: This study was supported by research grant from the project of Leading Talents in Pearl River Talent Plan of Guangdong Province (No. 81000-42020004).
Footnote
Conflicts of Interest: The authors have no conflicts of interest to declare.
Ethical Statement: The study was approved by the Institutional Animal Care and Use Committee of the Tang Wanchun Laboratories of Emergency & Critical Care Medicine at Sun Yat-sen Memorial Hospital, Sun Yat-sen University (IACUC-2016P1609).
References
- Kleinman ME, Brennan EE, Goldberger ZD, et al. Part 5: Adult Basic Life Support and Cardiopulmonary Resuscitation Quality: 2015 American Heart Association Guidelines Update for Cardiopulmonary Resuscitation and Emergency Cardiovascular Care. Circulation 2015;132:S414-35. [Crossref] [PubMed]
- Babbs CF, Kern KB. Optimum compression to ventilation ratios in CPR under realistic, practical conditions: a physiological and mathematical analysis. Resuscitation. 2002;54:147-57. [Crossref] [PubMed]
- Yannopoulos D, Aufderheide TP, Gabrielli A, et al. Clinical and hemodynamic comparison of 15:2 and 30:2 compression-to-ventilation ratios for cardiopulmonary resuscitation. Crit Care Med 2006;34:1444-9. [Crossref] [PubMed]
- Babbs CF. Effects of an impedance threshold valve upon hemodynamics in Standard CPR: studies in a refined computational model. Resuscitation 2005;66:335-45. [Crossref] [PubMed]
- Lurie KG, Zielinski T, McKnite S. The use of an inspiratory impedance valve improves neurologically intact survival in a porcine model of ventricular fibrillation. Circulation 2002;105:124-9. [Crossref] [PubMed]
- Berg RA, Kern KB, Hilwig RW, et al. Assisted ventilation during “bystander” CPR in a swine acute myocardial infarction model does not improve outcome. Circulation 1997;96:4364-71. [Crossref] [PubMed]
- Berg RA, Kern KB, Hilwig RW, et al. Assisted ventilation does not improve outcome in a porcine model of single-rescuer bystander cardiopulmonary resuscitation. Circulation 1997;95:1635-41. [Crossref] [PubMed]
- Ong ME, Ng FS, Anushia P, et al. Comparison of chest compression only and standard cardiopulmonary resuscitation for out-of-hospital cardiac arrest in Singapore. Resuscitation 2008;78:119-126. [Crossref] [PubMed]
- Bohm K, Rosenqvist M, Herlitz J, et al. Survival is similar after standard treatment and chest compression only in out-of-hospital bystander cardiopulmonary resuscitation. Circulation 2007;116:2908-12. [Crossref] [PubMed]
- Iwami T, Kawamura T, Hiraide A, et al. Effectiveness of bystander-initiated cardiac-only resuscitation for patients with out-of-hospital cardiac arrest. Circulation 2007;116:2900-7. [Crossref] [PubMed]
- SOS-KANTO Study Group. Cardiopulmonary resuscitation by bystanders with chest compression only (SOS-KANTO): an observational study. Lancet 2007;369:920-6. [Crossref] [PubMed]
- Chen W, Weng Y, Wu X, et al. The effects of a newly developed miniaturized mechanical chest compressor on outcomes of cardiopulmonary resuscitation in a porcine model. Crit Care Med 2012;40:3007-12. [Crossref] [PubMed]
- Tang W, Weil MH, Schock RB, et al. Phased chest and abdominal compression-decompression: a new option for cardiopulmonary resuscitation. Circulation 1997;95:1335-40. [Crossref] [PubMed]
- García-Delgado M, Touma-Fernandez A, Chamorro-Marin V, et al. Alveolar fluid clearance in healthy pigs and influence of positive end-expiratory pressure. Critical care (London, England) 2010;14:R36. [Crossref] [PubMed]
- Wang S, Wu JY, Guo ZJ, et al. Effect of rescue breathing during cardiopulmonary resuscitation on lung function after restoration of spontaneous circulation in a porcine model of prolonged cardiac arrest. Crit Care Med 2013;41:102-10. [Crossref] [PubMed]
- Ewy GA, Zuercher M, Hilwig RW, et al. Improved neurological outcome with continuous chest compressions compared with 30:2 compressions-to-ventilations cardiopulmonary resuscitation in a realistic swine model of out-of-hospital cardiac arrest. Circulation 2007;116:2525-30. [Crossref] [PubMed]
- Sato Y, Weil MH, Sun S, et al. Adverse effects of interrupting precordial compression during cardiopulmonary resuscitation. Crit Care Med 1997;25:733-6. [Crossref] [PubMed]
- Yu T, Weil MH, Tang W, et al. Adverse outcomes of interrupted precordial compression during automated defibrillation. Circulation 2002;106:368-72. [Crossref] [PubMed]
- Cunningham LM, Mattu A, O'Connor RE, Brady WJ. Cardiopulmonary resuscitation for cardiac arrest: the importance of uninterrupted chest compressions in cardiac arrest resuscitation. Am J Emerg Med 2012;30:1630-8. [Crossref] [PubMed]
- Yannopoulos D, Matsuura T, McKnite S, et al. No assisted ventilation cardiopulmonary resuscitation and 24-hour neurological outcomes in a porcine model of cardiac arrest. Crit Care Med 2010;38:254-60. [Crossref] [PubMed]
- Markstaller K, Rudolph A, Karmrodt J, et al. Effect of chest compressions only during experimental basic life support on alveolar collapse and recruitment. Resuscitation 2008;79:125-32. [Crossref] [PubMed]
- Vaillancourt C, Everson-Stewart S, Christenson J, et al. The impact of increased chest compression fraction on return of spontaneous circulation for out-of-hospital cardiac arrest patients not in ventricular fibrillation. Resuscitation. 2011;82:1501-7. [Crossref] [PubMed]
- Kern KB, Hilwig RW, Berg RA, et al. Importance of continuous chest compressions during cardiopulmonary resuscitation: improved outcome during a simulated single lay-rescuer scenario. Circulation 2002;105:645-9. [Crossref] [PubMed]
- Yang L, Weil MH, Noc M, et al. Spontaneous gasping increases the ability to resuscitate during experimental cardiopulmonary resuscitation. Crit Care Med. 1994;22:879-83. [Crossref] [PubMed]
- Noc M, Weil MH, Sun S, et al. Spontaneous gasping during cardiopulmonary resuscitation without mechanical ventilation. Am J Respir Crit Care Med 1994;150:861-4. [Crossref] [PubMed]