Matrix-assisted laser desorption ionization time-of-flight mass spectrometry: a powerful tool for identification of Corynebacterium species
Introduction
Corynebacterium spp. commonly colonizes the skin and mucous membranes of humans, which rarely account for clinical infections. However, several recent studies reported that Corynebacterium spp. would result in opportunistic infection including artificial joint infections, endocarditis, pneumonia and catheter-related bloodstream infections (CRBSI) (1,2). Correct and rapid identification of Corynebacterium spp. is a crucial to identify the real source of infection and install the appropriate treatment for the infection. Current bacterial identification methods such as API Cory (bioMerieux) and BD Phoenix Automated Microbiology System (Becton Dickinson, Sparks, MD, USA) are generally based on conventional phenotypic and biochemical methods. Most of these tests are laborious, time-consuming processes and do not always give reliable identification at the species level. Therefore, accurate and rapid identification of Corynebacterium spp. help clinicians to identify isolates of pathogens or contaminating bacteria. Recently, matrix-assisted laser desorption ionization time-of-flight mass spectrometry (MALDI-TOF MS), based on the protein composition of microbial cells, has emerged as a promising technique which is a rapid, reliable diagnostic tool for the identification of most microorganism including Corynebacterium spp. (3-5). There is limited data evaluating the use of this tool for the identification of Corynebacterium spp., and these studies have been done mostly using the Bruker system rather than VITEK MS (bioMérieux). The aims of our study were to evaluate the VITEK MS in quickly identification of Corynebacterium spp. by compared with API Coryne and Phoenix. All strains were confirmed by rpoB sequencing as the gold standard.
Methods
Bacterial isolates
All Corynebacterium isolates were collected from routine examination of human clinical specimen in Zhongshan Hospital of Fudan University from 2012 to 2013, which contained blood, central venous catheter, ascites, hydrothorax, urine, pus and excreta. These strains obtained from the sterile site such as blood and central venous catheter were identified as pathogenic bacteria, while isolates obtained from other sites contained both pathogenic and contamination bacteria. All isolates were recultured after incubation at 35 °C on sheep blood agar (bioMerieux, France) and stored at −20 °C for later use.
Biochemical identification
After gram staining, all trains were identified by API Coryne strips (bioMerieux, Marcy I’Etoile, France), Phoenix (BD, Sparks, MD, USA) and VITEK MS (bioMerieux, Marcy l’Etoile, France), respectively. BD Phoenix Automated Microbiology System was applied to identify the bacteria with Bacteria identification strips PMIC/ID (BD PhoenixPID, panel448505, Franklin Lakes, USA). API Coryne strips was also used for identification in accordance with the standard procedure recommended by the manufacturer.
Molecular identification
For partial rpoB gene sequencing, DNA was first extracted by heat and then stored at −70 °C. Polymerase chain reaction (PCR) was operated according to instructions by Khamis A (6). PCR analysis included 35 cycles of denaturation at 94 °C for 30 s, primer annealing at 57 °C for 30 s, and extension at 72 °C for 2 min, after a denaturation step of 2 min. PCR amplification products were delivered to the Liuhe Bejing Genomics Institute of Science and Technology Co., Ltd. for sequencing. The gene sequences were then compared using the BLAST website (http://blast.ncbi.nlm.nih.gov/Blast.cgi) with more than 97% similarity considered as cut-off for reliable species identification.
MALDI-TOF mass spectrometry
For VITEK MS, all strains were subcultured on sheep blood agar plates (bioMérieux) at 37 °C for 24 to 48 h and identified by the VITEK MS system using a single deposit directly from bacterial colonies according to the manufacturer’s guidelines. Briefly, a single colony or multiple small colonies of each isolate were smeared onto the VITEK MS-DS target slide (bioMérieux), supplied in a 48-well microscope slide format, followed by overlaying with 1 µL of α-cyano-4-hydroxycinnamic acid (CHCA) matrix solution (bioMérieux) and dried at room temperature for later target interrogation by the VITEK MS mass spectrometer (bioMérieux). The system reported the best identification with confidence values from 0 to 99.9%. Escherichia coli ATCC 8739 was used as internal identification control during the whole analysis process (7,8).
Data analysis
The VITEK MS identification system is based on comparison of the characteristics of the obtained spectra with those of the VITEK MS v2.0 database (7,8). All of the statistical analyses were performed with SPSS 19.0. All P values were based on two-sided tests and a P value of less than 0.05 was considered statistically significant.
Results
Source of specimen and bacteria species
A total of 75 corynebacterium spp. isolates were collected from various clinical specimens, which mainly contains blood (28.0%), pus (26.7%), hydrothorax (16.0%), central venous catheter (5.3%) and urine (18.7%) (Table 1). According to rpoB gene sequencing, isolates were identified into 12 groups. Corynebacterium striatum (58.7%) was the most common species in clinical isolates, followed by Corynebacterium jeikeium (16.0%), Corynebacterium amycolatum (5.3%) and Corynebacterium urealyticum (5.3%) (Table 2).
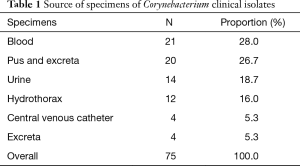
Full table
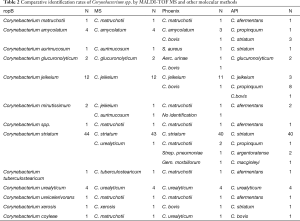
Full table
All strains were identified with MS to the genus level and 69 of which were correctly identified to the species level. The other six strains were identified as C. jeikeium, C. aurimucosum and C. matruchotii. In 49 of 75 strains (65.3%), biochemical identification by API Coryne yielded identical results as rpoB gene sequencing. Twenty-six strains showed unreliable or ambiguous API results and were only identified to genus level (Corynebacterium spp.).
According to rpoB sequencing, there was no significant difference in the identification of corynebacterium to genus level by MS (100%, 75/75) or Phoenix (93.3%, 70/75) (P=0.058). 92.0% (69/75) strains were successfully identified to species by MS but just 78.7% and 65.3% strains were identified by Phoenix and API, respectively. Compared with gene sequencing, the coincidence rate of identification by MS was significantly higher than Phoenix (P=0.036) and API (P<0.001) in the species level. The turn-around time of each strain by MS was about 3–5 min.
Identification of Corynebacterium spp.
Sixty-nine bacterial results are consistent with the sequencing results for MS, the remaining six were identified as C. jeikeium, C. aurimucosum and C. matruchotii. Phoenix and API were successfully identified 59 and 49 Corynebacterium, and the rest were identified as Corynebacterium species and other Gram-positive bacteria (Table 2).
Validation of the different methods for identifying Corynebacterium spp.
The results of VITEK MS and Phoenix at genus level was 100% and 93.3%, both two methods shown no significant difference (P=0.058). Among all clinical isolates, 69 strains were identified by MS, and the results were consistent with the sequencing results, and the rate was 92%, significantly higher than that of Phoenix (P=0.036) and API (P<0.001). There was no statistical significance (P=0.101) between Phoenix and API, as shown in Table 3.
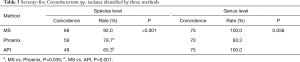
Full table
Turnaround time for identification
There was no significant difference for preparation time of three methods. However, conventional biochemical identification methods such as API require 16–24 h, and about 15 h for automated bacterial identification instrument (Phoenix). Remarkably, per plant bacterial identification time is just 3–5 min for MS on average, as shown in Table 4.
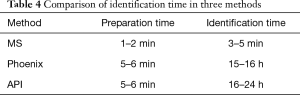
Full table
Discussion
There are more than 120 species in the genus Corynebacterium, which contains clinically relevant species as well as opportunistic commensals (9). The most widely known pathogenic species is C. diphtheriae causing diphtheria, a potentially fatal disease, by toxigenic strains. Over recent years, non-diphtheriae Corynebacterium has been recognized as opportunistic pathogens which cause various types of healthcare-associated infections (1,2,10,11). However, they are always considered as contaminants and have not received a great deal of attention when recovered from clinical specimens. For many years, the identification of microorganisms in clinical microbiology laboratories has been mainly relied on phenotypic methods such as biochemical reactions, molecular techniques including PCR and sequencing. API Coryne, commercially available manual identification test strips, and automated systems include Vitek 2 (bioMérieux) and Phoenix (Becton Dickinson, Sparks, MD, USA) are commonly used in many laboratories when identification of Corynebacterium are needed. However, they usually rely on active metabolic processes of the microorganisms and therefore long periods are needed sometimes and usually provide the user with several possible species. As a result, additional tests should be done to discriminate these choices. PCR technology has been used as an alternative approach, but because of its costly and complicated, it is not suitable for routine use in every laboratory.
Therefore, a fast and reliable identification of Corynebacterium species is crucial.
MALDI-TOF MS, nowadays, for its speed, low per-sample cost and accurate approach, has been increasingly applied in clinical microbial laboratory as a new technology for species identification (1,12).
Most published studies reporting identification of Corynebacterium strains using MS focused on the potentially toxigenic species C. diphtheria, C. ulcerans and C. Pseudotuberculosis (3). In our study, 75 Corynebacterium isolates recovered from clinical specimen were identified to 12 species, which represent common species isolated in the routine work of our clinical microbiology laboratory. Although the most widely known pathogenic species of Corynebacterium is C. diphtheriae, there was no C. diphtheria having been found. The dominant isolates in our study were C. striatum and C. jeikeium, which have also been reported as the cause of outbreaks of healthcare-associated infections, remarkably (13-16).
In our experience, MS and ropB gene sequencing showed identical results for 69 (92.0%) of all 75 tested Corynebacterium strains in species level, which is almost similar as reported by Alibi et al. (94.84%) (17) and Theel ES et al. (92.3%) (18) using the Bruker Biotyper MALDI TOF MS system (Billerica, MA, USA), while Phoenix and API reliably identified 59 (78.7%) and 49 (65.3%) strains respectively. Although the agreement between API Coryne and MS has been reported in 87% cases by Alatoom et al. (5) and 88.7% by Alibi et al. (17), our data show a lower agreement (65.3%) between two methods. Twenty-six strains showed unreliable or ambiguous API results and were only identified to genus level (Corynebacterium spp.). Phoenix showed a better agreement (78.7%) than API but five strains were failed to identify to genus level. The reason of the lower agreement maybe as follows: (I) some strains maybe misidentified or contaminated during the testing; (II) there are not enough number of substrates in panels to differentiate all Corynebacterium spp.; (III) the databases have only been infrequently updated and do not included newly described species. There was one strain identified to species level by MS but only to genus level by molecular methods. As partial rpoB sequencing is an efficient mean for identification of corynebacteria, some research showed that there are not completely congruent with by rpoB gene and 16sRNA (19). It was reported that even when sent to a reference laboratory, 30–50% of coryneform bacteria isolates cannot be reliably identified at the species level. Nevertheless, further study could be taken to focus on these strains.
As MALDI-TOF MS is reported to be widely used in routine laboratories (20-23). The most prominent advantages are its speed and low running costs provided that a quality-controlled database of reference spectra including all relevant microorganisms is available (3). As showed in our research, it takes at least 16 hours to get the final results whether use API Coryne or Phoenix. RpoB gene sequencing seems to take up to several days until a result is available and requires trained staff. However, teaching the use of MS to laboratory technician personal requires only about 1 h, and it only takes about 5 min on identification by MS. Moreover, MS could be applied to detect in large quantities while API strips could only be done one by one. Without regard to equipment costs, single sample testing by VITEK MS costs less than Phoenix and API testing in reagent cost. Obviously, these features and advantages in workflow also reduce both laboratory technician hands-on time and material costs. Furthermore, this convenient technology could not only change the concept that Corynebacteria always be contamination and do not need attention in current laboratory but also provide evidence for clinical to differentiate pathogenic or contaminated bacteria as soon as possible.
Our study also has some limitations. First, we had limited numbers of Corynebacterium isolates and species. The potentially toxigenic Corynebacterium species, C. diphtheriae, C. ulcerans and C. pseudotuberculosis and some special species, C. pseudodiphtheriticum and C. propinquum had not been found. However, clinically significant Corynebacterium species recovered in our laboratory were included in this study. Second, we only made a simple comparison of the cost of reagents in three methods without considering the initial cost. Previous evaluations have compared the accuracy. Compared with standard protocols, the MALDI protocol provided identifications 1.45 days earlier on average and can reduce reagent and labor costs of identification by $102,424 or 56.9% within 12 months in K. E. Tan’s study (24).
Conclusions
For supporting clinical decisions to distinguish Corynebacterium spp. from colonization bacteria or opportunistic pathogens, MALDI-TOF MS is needed and will undoubtedly change the approach used by clinical laboratories for the identification in routine work because of its speed, ease of use, cost-efficient and reliable approach for Corynebacterium identification.
Acknowledgements
None.
Footnote
Conflicts of Interest: The authors have no conflicts of interest to declare.
References
- Bernard K. The genus corynebacterium and other medically relevant coryneform-like bacteria. J Clin Microbiol 2012;50:3152-8. [Crossref] [PubMed]
- Díez-Aguilar M, Ruiz-Garbajosa P, Fernández-Olmos A, et al. Non-diphtheriae Corynebacterium species: an emerging respiratory pathogen. Eur J Clin Microbiol Infect Dis 2013;32:769-72. [Crossref] [PubMed]
- Konrad R, Berger A, Huber I, et al. Matrix-assisted laser desorption/ionisation time-of-flight (MALDI-TOF) mass spectrometry as a tool for rapid diagnosis of potentially toxigenic Corynebacterium species in the laboratory management of diphtheria-associated bacteria. Euro Surveill 2010;15:19699. [PubMed]
- Bizzini A, Greub G. Matrix-assisted laser desorption ionization time-of-flight mass spectrometry, a revolution in clinical microbial identification. Clin Microbiol Infect 2010;16:1614-9. [Crossref] [PubMed]
- Alatoom AA, Cazanave CJ, Cunningham SA, et al. Identification of non-diphtheriae corynebacterium by use of matrix-assisted laser desorption ionization-time of flight mass spectrometry. J Clin Microbiol 2012;50:160-3. [Crossref] [PubMed]
- Khamis A, Raoult D, La Scola B. rpoB gene sequencing for identification of Corynebacterium species. J Clin Microbiol 2004;42:3925-31. [Crossref] [PubMed]
- Lee W, Kim M, Yong D, et al. Evaluation of VITEK mass spectrometry (MS), a matrix-assisted laser desorption ionization time-of-flight MS system for identification of anaerobic bacteria. Ann Lab Med 2015;35:69-75. [Crossref] [PubMed]
- Rychert J, Burnham CA, Bythrow M, et al. Multicenter evaluation of the Vitek MS matrix-assisted laser desorption ionization-time of flight mass spectrometry system for identification of Gram-positive aerobic bacteria. J Clin Microbiol 2013;51:2225-31. [Crossref] [PubMed]
- Harrington AT, Mizuki S, Bui U, et al. Hardware Infection with Corynebacterium spp.: a Case Report and Review of the Literature. Clinical Microbiology Newsletter 2014;36:9-13. [Crossref]
- Belmares J, Detterline S, Pak JB, et al. Corynebacterium endocarditis species-specific risk factors and outcomes. BMC Infect Dis 2007;7:4. [Crossref] [PubMed]
- Nhan TX, Parienti JJ, Badiou G, et al. Microbiological investigation and clinical significance of Corynebacterium spp. in respiratory specimens. Diagn Microbiol Infect Dis 2012;74:236-41. [Crossref] [PubMed]
- Carbonnelle E, Mesquita C, Bille E, et al. MALDI-TOF mass spectrometry tools for bacterial identification in clinical microbiology laboratory. Clin Biochem 2011;44:104-9. [Crossref] [PubMed]
- Chen FL, Hsueh PR, Teng SO, et al. Corynebacterium striatum bacteremia associated with central venous catheter infection. J Microbiol Immunol Infect 2012;45:255-8. [Crossref] [PubMed]
- Verroken A, Bauraing C, Deplano A, et al. Epidemiological investigation of a nosocomial outbreak of multidrug-resistant Corynebacterium striatum at one Belgian university hospital. Clin Microbiol Infect 2014;20:44-50. [Crossref] [PubMed]
- Mookadam F, Cikes M, Baddour LM, et al. Corynebacterium jeikeium endocarditis: a systematic overview spanning four decades. Eur J Clin Microbiol Infect Dis 2006;25:349-53. [Crossref] [PubMed]
- Ifantidou AM, Diamantidis MD, Tseliki G, et al. Corynebacterium jeikeium bacteremia in a hemodialyzed patient. Int J Infect Dis 2010;14 Suppl 3:e265-8. [Crossref] [PubMed]
- Alibi S, Ferjani A, Gaillot O, et al. Identification of clinically relevant Corynebacterium strains by Api Coryne, MALDI-TOF-mass spectrometry and molecular approaches. Pathol Biol (Paris) 2015;63:153-7. [Crossref] [PubMed]
- Theel ES, Schmitt BH, Hall L, et al. Formic acid-based direct, on-plate testing of yeast and Corynebacterium species by Bruker Biotyper matrix-assisted laser desorption ionization-time of flight mass spectrometry. J Clin Microbiol 2012;50:3093-5. [Crossref] [PubMed]
- Khamis A, Raoult D, La Scola B. Comparison between rpoB and 16S rRNA gene sequencing for molecular identification of 168 clinical isolates of Corynebacterium. J Clin Microbiol 2005;43:1934-6. [Crossref] [PubMed]
- Seng P, Drancourt M, Gouriet F, et al. Ongoing revolution in bacteriology: routine identification of bacteria by matrix-assisted laser desorption ionization time-of-flight mass spectrometry. Clin Infect Dis 2009;49:543-51. [Crossref] [PubMed]
- van Veen SQ, Claas EC, Kuijper EJ. High-throughput identification of bacteria and yeast by matrix-assisted laser desorption ionization-time of flight mass spectrometry in conventional medical microbiology laboratories. J Clin Microbiol 2010;48:900-7. [Crossref] [PubMed]
- Sauer S, Kliem M. Mass spectrometry tools for the classification and identification of bacteria. Nat Rev Microbiol 2010;8:74-82. [Crossref] [PubMed]
- Navas M, Pincus DH, Wilkey K, et al. Identification of aerobic Gram-positive bacilli by use of Vitek MS. J Clin Microbiol 2014;52:1274-7. [Crossref] [PubMed]
- Tan KE, Ellis BC, Lee R, et al. Prospective evaluation of a matrix-assisted laser desorption ionization-time of flight mass spectrometry system in a hospital clinical microbiology laboratory for identification of bacteria and yeasts: a bench-by-bench study for assessing the impact on time to identification and cost-effectiveness. J Clin Microbiol 2012;50:3301-8. [Crossref] [PubMed]