Peroxisome proliferator-activated receptor-γ in induced sputum is correlated with MMP-9/TIMP-1 imbalance and formation of emphysema in COPD patients
Introduction
Chronic obstructive pulmonary disease (COPD) is an inflammatory airway disease with irreversible progression due to the exposure to harmful matter, especially the cigarette smoke (CS) (1). This airway inflammation as the response to the CS exposure can lead to injury of lung parenchymal destruction and the subsequent formation of emphysema (1,2). Strong evidence suggests that proteases critically contribute to the pathological processes detected in COPD (3,4). The disturbance of the balance of matrix metalloproteinases (MMPs) and counter-acting tissue inhibitors of metalloproteinases (TIMPs) is one of the key mechanisms for the development of COPD and emphysema. MMPs, including gelatinases, elastases, and collagenases, and cleave extracellular matrix proteins (ECM). TIMPs, binding to and inactivate active MMPs, are endogenous antagonists of the metalloproteinase network, while MMPs are involved in the degeneration of the lung parenchyma and the emphysema formation (3). The search for key factors responsible for maintaining the dynamic balancing and shifting between MMPs and TIMPs is critical for the identification of potential targets in COPD treatment.
Peroxisome proliferator-activated receptors (PPARs), as one ligand-activated nuclear hormone receptor, engage in cell function (5). PPARγ is located and expressed in the antigen-presenting cell and takes part in numerous anti-inflammation functions and repair of tissue (6-8). It is demonstrated that PPARγ deletion from alveolar macrophages led to the inflammatory changes of airway in vivo (9), whereas the susceptibility to emphysema induced by CS was increased in vivo specifically deleting PPARγ expression in the airway epithelial cells (9,10). In our previous study, it is found that the exposure to CS could decrease PPARγ level in macrophages and bronchial epithelial cells of rats. Besides, the oral administration of the PPARγ agonist rosiglitazone attenuated CS-induced metalloproteinase activity (11,12). These results indicate that PPARγ as one of the formation of emphysema the imbalance of MMPs and TIMPs.
In our study, we explored the association between PPARγ expression and MMP-9/TIMP-1 imbalance in the induced sputum of stable COPD patients with smoking history, thus to discuss the role of PPARγ in emphysema progression in COPD.
Methods
Patients
Between June 2012 and July 2013, based on the criteria and classification of the Global Initiative for Chronic Obstructive Lung Disease (GOLD) guidelines (13), 66 patients with stable COPD at the First Affiliated Hospital of China Medical University were recruited consecutively and followed up at the pulmonary medicine outpatient clinics. The control group included 12 healthy non-smoking outpatients without any known diseases who were seen at the clinics for routine health examinations.
The inclusion criteria were as follows: (I) stable COPD; (II) patients who have received the treatment of standard inhaled corticosteroids, bronchodilators, and if required, supplemental oxygen; and (III) patients with a smoking history of more than 10 pack-years.
The exclusion criteria were as follows: (I) except for the treatment of COPD, patients had medication taking history for other diseases; (II) patients with exacerbation or utilization of systemic corticosteroids (oral or intravenous injection therapy) within the past 6 months; (III) patients requiring antibiotics for signs of a bacterial infection; (IV) COPD patients admitted with complications or comorbidities such as pulmonary embolism, pneumonia, heart failure, asthma, lung carcinoma, tuberculosis or bronchiectasis; and (V) patients with respiratory failure requiring mechanical ventilation or oxygen supplementation >15 h per day.
This study was approved by the ethics review board of The First Affiliated Hospital, China Medical University (No. 201105). Each eligible patient was enrolled after written informed consent was obtained.
Patient questionnaires
Dyspnea severity was determined by the modified Medical Research Council (mMRC) dyspnea scale, with a 5 point scale to assess the severity (14). Status of health was assessed by the questionnaire of COPD Assessment Test (CAT) as well as St George’s Respiratory Questionnaire (SGRQ). The SGRQ focuses on three major health-related quality of life (HRQoL) domains (15), namely symptoms, activity, and impacts. Scores from these three domains are combined into a total score, ranging from 0 to 100. The scores on the CAT (16) is a 50-point scale, with a score of 0 as the lowest level and no impairment. The CAT, SGRQ, and mMRC were self-administered under staff supervision.
Pulmonary function tests
Pulmonary function test and plethysmography was performed in all participants following inhalation of salbutamol sulfate according to guidelines from the American Thoracic Society/European Respiratory Society (ATS/ERS) Task Force (17). COPD was diagnosed according to GOLD guidelines with the forced expiratory volume in one second (FEV1)/forced vital capacity (FVC) ratio <70% after post-bronchodilator usage, and the COPD severity was categorized by the current GOLD criteria (13).
Body-mass index, airflow obstruction, dyspnea and exercise index (BODE index)
BODE index is composed by four parts and calculated by summing of the scores of its components together (18): post-bronchodilator FEV1% predicted (FEV1%/Pred), body-mass index, walking distance of the 6-minute walking test (6MWT), and dyspnea scale of mMRC.
Chest computed tomography (CT) and measurement of emphysema
At enrollment, a high resolution chest computed tomography (HRCT) was done for every patient. Chest HRCT images were acquired by a CT scanner (Toshiba Medical Corporation, Tokyo, Japan) according to the following instructions: 1 mm collimation with the range from sternal notch to the diaphragm as the lowest end at a 0.5 mm interval in a supine position during one breath-holding after deep inspiration, at 135 kV and 170 mA with no contrast medium. The window width of the lung image was 1,000 HU and the window level was −700 HU. Emphysema severity was quantified as the percentage of low attenuation area (LAA%) in the lung below the fixed threshold, −950 HU, which was calculated by Lung CAD1.2 software (Neusoft, Shengyang, China). The COPD patients were further classified into the emphysema group and non-emphysema group based on LAA% (−950 HU) ≥15% and <15%, respectively (19).
Processing, cell counts and differentiating of the induced sputum
Induced sputum was processed according to the methods previously reported (20). All subjects were guided to do the mouth wash with water thoroughly. Three percent saline was inhaled by the subjects from an ultrasonic nebulizer. Then the patients were told to cough deeply at 3 min intervals. Sputum plugs were picked from the phlegm. Induced sputum supernatants was refrigerated after homogenization and operated within 60 min of accumulation. For the protein quantification assays, the supernatants were stored at −80 °C. Differential cell counts (≥400 non-squamous cells) were processed on the cytospin slides. Total cell counts were performed manually by a hemocytometer. Sputum sample adequacy was evaluated according to results previously published (21,22). Cell viability was confirmed by trypan blue staining method.
Differential cell count of inflammatory cells in the induced sputum
The pelleted cells of the induced sputum in PBS were resuspended first. Certain number of the cells (1×105 cells) was centrifugated and tossed to cytospin slides, fixed by methanol, and stained by May-Grünwald-Giemsa solution. Then the differential cell count was performed under the light microscope.
MMP-9, TIMP-1, and PPARã concentrations of the induced sputum measured by ELISA
MMP-9, TIMP-1, and PPARγ concentrations were quantified in the supernatants of the sputum using commercial sandwich ELISA kits following the manufacturer’s instructions (Human PPARγ ELISA Kit, NeoScientific, NEO Group Inc., USA; Human MMP-9 ELISA Kit and TIMP-1 ELISA System, GE Healthcare, UK Limited).
Statistical analyses
Continuous data are shown as the mean ± standard deviation (SD). Comparisons of different groups were performed by Mann-Whitney U-test. Correlation coefficient between each variable was analyzed by the Spearman’s rank correlation analyses. Statistical analyses were processed by SPSS 17.0 software (SPSS, Inc., Chicago, IL, USA). The statistical significance was confirmed when a P value <0.05.
Results
Demographics of the patients
The distribution of population number, age, and gender of the subjects enrolled is listed in Table 1 along with other characteristics. The healthy control and COPD groups were matched according to the age. Among the COPD patients, 4 subjects in GOLD stage I, 22 subjects in GOLD stage II, 32 in GOLD stage III, and 7 in GOLD stage IV. The BMI, FEV1, FEV1/Pred and FEV1/FVC values in the COPD patients were decreased when compared with those in the healthy control (Table 1).
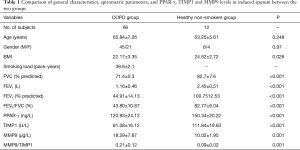
Full table
Comparison of the expression of MMP-9, TIMP-1 and PPARã in the induced sputum between healthy controls and COPD patients
The level of MMP-9 in the induced sputum was higher in COPD individuals than in healthy controls (Table 1), while the concentration of PPARγ and TIMP-1 was less in the COPD patients than those in the healthy population. In contrast, the concentration of MMP-9 and the ratio of MMP9/TIMP1 were higher in COPD patients when compared to controls.
Patients with different levels of pulmonary function impairment also exhibited variations in the expression of PPARγ, MMP-9, and TIMP-1in the induced sputum (Table 2). Among the COPD patients, those with worse lung function presented with increased MMP-9 expression, decreased TIMP-1 and PPARγ expression in the induced sputum.

Full table
Comparison of the concentrations of MMP-9, TIMP-1 and PPARã in the induced sputum of COPD patients with or without emphysema
A comparison of the clinical characteristics of these two groups revealed increased residual volume (RV), decreased exercise capacity (CAT and SGRQ scales), and worse mMRC dyspnea and BODE index scores. The PPARγ and TIMP-1 levels in the induced sputum were reduced in the emphysema group compared with those in the non-emphysema group of COPD patients, whereas the concentration of MMP-9 and the MMP9/TIMP1 ratio were higher in the emphysema group compared with those in the non-emphysema group (Table 3).
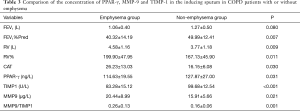
Full table
Factors correlating with the level of PPARã in the induced sputum
The factor-correlation analysis with concentration of PPARγ in the induced sputum was correlated with the FEV1%positively and the expression of TIMP-1 and negatively correlated with the expression of MMP-9, LAA%, RV, and RV/total lung capacity (TLC) (Table 4).
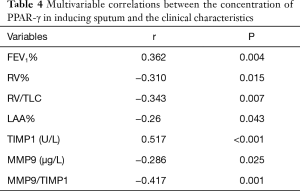
Full table
Discussion
In our study, we found decreased expression of PPARγ and MMP-9/TIMP-1 imbalance in the induced sputum of COPD individuals, distinctively in the emphysema subgroup. Reduced expression of PPARγ in the induced sputum correlated with metalloproteinase/anti-metalloproteinase imbalance, lung function injury and emphysema changes in COPD. Although our study is a cross-sectional observation, a role of PPARγ in the progression of emphysema was indicated from these data potentially. These results also demonstrated that increased expression of PPARγ significantly diminished the lung inflammation in COPD and emphysema.
PPARγ takes part in varieties of biological activities, such as inflammatory responses, metabolism, and cellular proliferation (23,24). In the lungs particularly, PPARγ was proved to be expressed in the macrophages, cells of airway smooth muscles, and epithelial cells (10,23,25,26). It has been proved that PPARγ played the anti-inflammatory role in monocytes/macrophages via the regulation of cytokine production (27). PPARγ activation inhibits airway epithelial cells and macrophages to release the proinflammatory cytokine (6,10,27). Macrophages take a great part in the pulmonary inflammatory response induced by chronic CS exposure in mice and are also the main component of bronchoalveolar lavage fluid (BALF) cells. Induced sputum is composed of inflammatory cells from the airway; therefore, we sought to investigate the expression of PPARγ in induced sputum. In our study, the level of PPARγ in the induced sputum was impoverished in COPD. In addition, the decrease of macrophage count in the induced sputum indicates the relationship between the anti-inflammatory effect of PPARγ and alveolar macrophages. A previous study exploring the effect of tiotropium observed a significant increase in PPARγ expression in sputum cells both after the long acting bronchodilator, tiotropium, and the combination of the long acting bronchodilator and inhaled corticosteroid, formoterol/inhaled corticosteroid, in COPD patients (28). These results indicate that decreased PPARγ expression correlates with exacerbation of airway inflammation and reduced lung function.
In this study, the decreased levels of PPARγ correlated with emphysema severity, as well as MMP-9/TIMP-1 imbalance. In our previous study, the activation of PPARγ decreased the expression of MMP-9 in lung tissue and reduced the histopathological characteristics of lung emphysema in a CS-induced emphysema model (11). We previously demonstrated in vitro that CS exposure diminishes PPARγ expression with the increase of inflammatory cytokine in isolated macrophages (12). In vitro experiments showed that emphysema patients with smoking history have not only reduced PPARγ expression in the myeloid dendritic cells from lungs, but also inhibited the activity of endogenous agonist of PPARγ (29). Knockout of PPARγ in antigen-presenting cells in mice led to lung inflammation spontaneously with the formation of emphysema, which looks like the manifestations of CS-exposed mice (29). In another study, deletion of PPARγ in airway epithelial cells shown the escalating formation of emphysema induced by CS with superfluous aggregation of macrophage and the release of chemokines, such as Cxcl15, Ccl5, and Cxcl10, through a direct interplay between PPARγ and NF-κB, including PPARγ-mediated impact on IκBɑ degeneration, nuclear translocation of p65, and the activation of IKK (10). These results revealed the critical role of PPARγ in the anti-inflammation in COPD, distinctly emphysema, and is likely released and regulated by macrophages.
An imbalance of TIMPs and MMPs is crucial in COPD (3). Although the concentration of MMP-9 in lung is reduced in COPD patients with smoking history (30-33), levels of MMP-9 are greater in both smokers and COPD patients than non-smokers (33-36). Alveolar macrophages from COPD patients produced more MMP-9 (37,38) and show higher enzymatic activity compared with non-smokers and healthy smokers (37). The putative source of MMP-9 could be both neutrophils and alveolar macrophages given that these cells isolated from COPD patients expressed MMP-9 (39). Airway epithelial cells could contribute to the MMP-9 levels, because compared with cells from healthy population without smoking history, these cells from both asymptomatic smokers and COPD patients’ exhibit increased MMP-9 mRNA expression (31). Increased MMP-9 expression might also be caused by increased release of macrophages. An increase in macrophages and the expression of PPARγ together with a change downward in neutrophils and MMP-9 expression in induced sputum might exhibit counter-regulatory functions in terms of protection from COPD. The counter-regulatory relationship between PPARγ and MMP-9 was also observed in some other lung diseases. In vitro, PPARγ agonists reduced the IL-8 release and MMP-9 from epithelial cells of airway as a reaction to the stimulation of TNF-α/IL-1β or PAO1 in cystic fibrosis model, by the partial interaction with NF-κB (40). It has been previously demonstrated that in bronchial epithelial cell lines derived from human beings (41). TNF-α and PMA-induced gelatinolytic activity of MMP-9 was reduced significantly by PPARγ agonists in a concentration dependent manner.
Regarding TIMP-1, the healthy non-smoker group exhibited significantly higher TIMP-1 expression than that in the COPD and healthy smoker groups (33,42). CS exposure could impair the interaction between TIMP-1 and MMP-9 in vivo. TIMP-1 acetylation could be induced by CS stimulation and disrupt its connection with MMP-9, thus to compromise its inhibition activity of MMPs (43). Thus in this study, we examined TIMP-1 to elucidate the relationship between PPARγ and MMP-9/TIMP-1 imbalance.
Our study suggested one potential mechanism for the formation of emphysema to COPD. There are two limitations as follows: firstly, given that smoke exposure itself could impose some effects on the changes of PPARγ and the harmonious arrangement between MMP-9 and TIMP-1, we only used healthy non-smokers in the control group instead of healthy population with smoking history; secondly, this is a one-center trial with a limited number of sample cases to explore the hypothesis we obtained from our animal and cell experiments.
Conclusions
In summary, decreased PPARγ expression in the induced sputum associated with reduced lung function and emphysema changes in COPD populations. Our results further reveal the protective role of PPARγ in the maintenance of the dynamic balance of MMP-9/TIMP-1 in COPD, distinctively in the emphysema subgroup, thus providing evidence for potential treatment targets in COPD.
Acknowledgements
Funding: This research was supported by three project grants (Ministry of Science and Technology of the People’s Republic of China, 2012BAI05B01; Science and Technology Department of Liaoning Province of the People’s Republic of China, L2013310; the Technology Department of Shenyang of the People’s Republic of China, F13-316-1-72).
Footnote
Conflicts of Interest: The authors have no conflicts of interest to declare.
Ethical Statement: This study was approved by the ethics review board of The First Affiliated Hospital, China Medical University (No. 201105). Each eligible patient was enrolled after written informed consent was obtained.
References
- Zuo L, He F, Sergakis GG, et al. Interrelated role of cigarette smoking, oxidative stress, and immune response in COPD and corresponding treatments. Am J Physiol Lung Cell Mol Physiol 2014;307:L205-18. [Crossref] [PubMed]
- Angelis N, Porpodis K, Zarogoulidis P, et al. Airway inflammation in chronic obstructive pulmonary disease. J Thorac Dis 2014;6 Suppl 1:S167-72. [PubMed]
- Navratilova Z, Kolek V, Petrek M. Matrix metalloproteinases and their inhibitors in chronic obstructive pulmonary disease. Arch Immunol Ther Exp (Warsz) 2016;64:177-93. [Crossref] [PubMed]
- Demkow U, van Overveld FJ. Role of elastases in the pathogenesis of chronic obstructive pulmonary disease: implications for treatment. Eur J Med Res 2010;15 Suppl 2:27-35. [PubMed]
- Berger J, Moller DE. The mechanisms of action of PPARs. Annu Rev Med 2002;53:409-35. [Crossref] [PubMed]
- Reddy RC. Immunomodulatory role of PPAR-gamma in alveolar macrophages. J Investig Med 2008;56:522-7. [Crossref] [PubMed]
- Denning GM, Stoll LL. Peroxisome proliferator-activated receptors: potential therapeutic targets in lung disease? Pediatr Pulmonol 2006;41:23-34. [Crossref] [PubMed]
- Belvisi MG, Mitchell JA. Targeting PPAR receptors in the airway for the treatment of inflammatory lung disease. Br J Pharmacol 2009;158:994-1003. [Crossref] [PubMed]
- Gautier EL, Chow A, Spanbroek R, et al. Systemic analysis of PPARgamma in mouse macrophage populations reveals marked diversity in expression with critical roles in resolution of inflammation and airway immunity. J Immunol 2012;189:2614-24. [Crossref] [PubMed]
- Solleti SK, Simon DM, Srisuma S, et al. Airway epithelial cell PPARgamma modulates cigarette smoke-induced chemokine expression and emphysema susceptibility in mice. Am J Physiol Lung Cell Mol Physiol 2015;309:L293-304. [Crossref] [PubMed]
- Hou G, Yin Y, Han D, et al. Rosiglitazone attenuates the metalloprotease/anti-metalloprotease imbalance in emphysema induced by cigarette smoke: involvement of extracellular signal-regulated kinase and NFkappaB signaling. Int J Chron Obstruct Pulmon Dis 2015;10:715-24. [PubMed]
- Yin Y, Hou G, Li E, et al. PPARgamma agonists regulate tobacco smoke-induced toll like receptor 4 expression in alveolar macrophages. Respir Res 2014;15:28. [Crossref] [PubMed]
- Global Initiative for Chronic Obstructive Lung Disease (GOLD). Global strategy for the diagnosis, management, and prevention of chronic obstructive lung disease. Global Initiative for Chronic Obstructive Lung Disease, Inc; Revised 2011; Available from: http://www.goldcopd.org/uploads/users/files/GOLD_Report_2011_Feb21.pdf
- Bestall JC, Paul EA, Garrod R, et al. Usefulness of the Medical Research Council (MRC) dyspnoea scale as a measure of disability in patients with chronic obstructive pulmonary disease. Thorax 1999;54:581-6. [Crossref] [PubMed]
- Jones PW, Quirk FH, Baveystock CM. The St George's Respiratory Questionnaire. Respir Med 1991;85 Suppl B:25-31; discussion 33-7.
- Kelly JL, Bamsey O, Smith C, et al. Health status assessment in routine clinical practice: the chronic obstructive pulmonary disease assessment test score in outpatients. Respiration 2012;84:193-9. [Crossref] [PubMed]
- Laszlo G. Standardisation of lung function testing: helpful guidance from the ATS/ERS Task Force. Thorax 2006;61:744-6. [Crossref] [PubMed]
- Celli BR, Cote CG, Marin JM, et al. The body-mass index, airflow obstruction, dyspnea, and exercise capacity index in chronic obstructive pulmonary disease. N Engl J Med 2004;350:1005-12. [Crossref] [PubMed]
- Blechschmidt RA, Werthschutzky R, Lorcher U. Automated CT image evaluation of the lung: a morphology-based concept. IEEE Trans Med Imaging 2001;20:434-42. [Crossref] [PubMed]
- Holz O, Tal-Singer R, Kanniess F, et al. Validation of the human ozone challenge model as a tool for assessing anti-inflammatory drugs in early development. J Clin Pharmacol 2005;45:498-503. [Crossref] [PubMed]
- Efthimiadis A, Spanevello A, Hamid Q, et al. Methods of sputum processing for cell counts, immunocytochemistry and in situ hybridisation. Eur Respir J Suppl 2002;37:19s-23s. [PubMed]
- Bartoli ML, Bacci E, Carnevali S, et al. Quality evaluation of samples obtained by spontaneous or induced sputum: comparison between two methods of processing and relationship with clinical and functional findings. J Asthma 2002;39:479-86. [Crossref] [PubMed]
- Becker J, Delayre-Orthez C, Frossard N, et al. Regulation of inflammation by PPARs: a future approach to treat lung inflammatory diseases? Fundam Clin Pharmacol 2006;20:429-47. [Crossref] [PubMed]
- Heikkinen S, Auwerx J, Argmann CA. PPARgamma in human and mouse physiology. Biochim Biophys Acta 2007;1771:999-1013. [Crossref] [PubMed]
- Kliewer SA, Forman BM, Blumberg B, et al. Differential expression and activation of a family of murine peroxisome proliferator-activated receptors. Proc Natl Acad Sci USA 1994;91:7355-9. [Crossref] [PubMed]
- Reddy RC, Keshamouni VG, Jaigirdar SH, et al. Deactivation of murine alveolar macrophages by peroxisome proliferator-activated receptor-gamma ligands. Am J Physiol Lung Cell Mol Physiol 2004;286:L613-9. [Crossref] [PubMed]
- Clark RB. The role of PPARs in inflammation and immunity. J Leukoc Biol 2002;71:388-400. [PubMed]
- Holownia A, Mroz RM, Noparlik J, et al. Expression of CREB-binding protein and peroxisome proliferator-activated receptor gamma during formoterol or formoterol and corticosteroid therapy of chronic obstructive pulmonary disease. J Physiol Pharmacol 2008;59 Suppl 6:303-9. [PubMed]
- Shan M, You R, Yuan X, et al. Agonistic induction of PPARgamma reverses cigarette smoke-induced emphysema. J Clin Invest 2014;124:1371-81. [Crossref] [PubMed]
- Russell RE, Culpitt SV, DeMatos C, et al. Release and activity of matrix metalloproteinase-9 and tissue inhibitor of metalloproteinase-1 by alveolar macrophages from patients with chronic obstructive pulmonary disease. Am J Respir Cell Mol Biol 2002;26:602-9. [Crossref] [PubMed]
- Kwiatkowska S, Noweta K, Zieba M, et al. Enhanced exhalation of matrix metalloproteinase-9 and tissue inhibitor of metalloproteinase-1 in patients with COPD exacerbation: a prospective study. Respiration 2012;84:231-41. [Crossref] [PubMed]
- Paone G, Conti V, Vestri A, et al. Analysis of sputum markers in the evaluation of lung inflammation and functional impairment in symptomatic smokers and COPD patients. Dis Markers 2011;31:91-100. [Crossref] [PubMed]
- Mercer PF, Shute JK, Bhowmik A, et al. MMP-9, TIMP-1 and inflammatory cells in sputum from COPD patients during exacerbation. Respir Res 2005;6:151. [Crossref] [PubMed]
- Ilumets H, Mazur W, Toljamo T, et al. Ageing and smoking contribute to plasma surfactant proteins and protease imbalance with correlations to airway obstruction. BMC Pulm Med 2011;11:19. [Crossref] [PubMed]
- Dickens JA, Miller BE, Edwards LD, et al. COPD association and repeatability of blood biomarkers in the ECLIPSE cohort. Respir Res 2011;12:146. [Crossref] [PubMed]
- Ji J, von Scheele I, Bergstrom J, et al. Compartment differences of inflammatory activity in chronic obstructive pulmonary disease. Respir Res 2014;15:104. [Crossref] [PubMed]
- Finlay GA, O'Driscoll LR, Russell KJ, et al. Matrix metalloproteinase expression and production by alveolar macrophages in emphysema. Am J Respir Crit Care Med 1997;156:240-7. [Crossref] [PubMed]
- Lim S, Roche N, Oliver BG, et al. Balance of matrix metalloprotease-9 and tissue inhibitor of metalloprotease-1 from alveolar macrophages in cigarette smokers. Regulation by interleukin-10. Am J Respir Crit Care Med 2000;162:1355-60. [Crossref] [PubMed]
- Vernooy JH, Lindeman JH, Jacobs JA, et al. Increased activity of matrix metalloproteinase-8 and matrix metalloproteinase-9 in induced sputum from patients with COPD. Chest 2004;126:1802-10. [Crossref] [PubMed]
- Perez A, van Heeckeren AM, Nichols D, et al. Peroxisome proliferator-activated receptor-gamma in cystic fibrosis lung epithelium. Am J Physiol Lung Cell Mol Physiol 2008;295:L303-13. [Crossref] [PubMed]
- Hetzel M, Walcher D, Grüb M, et al. Inhibition of MMP-9 expression by PPARgamma activators in human bronchial epithelial cells. Thorax 2003;58:778-83. [Crossref] [PubMed]
- Kang MJ, Oh YM, Lee JC, et al. Lung matrix metalloproteinase-9 correlates with cigarette smoking and obstruction of airflow. J Korean Med Sci 2003;18:821-7. [Crossref] [PubMed]
- Yao H, Hwang JW, Sundar IK, et al. SIRT1 redresses the imbalance of tissue inhibitor of matrix metalloproteinase-1 and matrix metalloproteinase-9 in the development of mouse emphysema and human COPD. Am J Physiol Lung Cell Mol Physiol 2013;305:L615-24. [Crossref] [PubMed]