Screening for lung cancer with low-dose computed tomography: a review of current status
Introduction
The rationale for lung cancer screening
Lung cancer caused an estimated 1.4 million deaths in 2008 (1), and is the leading cause of cancer death worldwide. Incidence and mortality closely follow smoking trends with a time-lag of twenty years. This explains why death rates are falling or plateauing in countries such as the US, yet rising in others such as China (2,3). Lung cancer carries a poor prognosis with reported overall five year survival between 8 and 16% in Europe and the USA, and between 6% and 32% in China (4-6).
Currently 25-30% of patients present with localised, potentially curable disease. Five year survival for those with pathological stage IA non-small cell lung cancer (NSCLC) is 73% whereas metastatic disease has a dismal prognosis (13% 5-year survival) (7,8).
Given that lung cancer has a detectable pre-clinical phase, effective treatment, especially surgery, with effective and potentially cost-effective applicable screening methods, it would seem to fulfil the criteria for screening first described by Wilson and Jungner (9) (Box 1). Although early screening studies using plain chest radiography (CXR) had methodological drawbacks (11), it is generally accepted that CXR screening does not confer a mortality benefit, a conclusion reinforced by the Prostate, Lung, Colorectal and Ovarian (PLCO) Cancer Screening Trial (12). In contrast, computed tomography (CT) is a far more sensitive imaging modality which has been studied for its potential utility in lung cancer screening over the past 25 years. Recently, the National Lung Screening Trial (13) showed that low-dose CT (LDCT) screening reduced lung cancer mortality by 20% compared with CXR screening. This was the first demonstration in a randomized clinical trial of a mortality reduction with screening. In response to these findings several expert bodies in the USA issued guidelines for screening high-risk populations and the US Preventive Services Task Force has awarded a Grade B draft recommendation (14-17).
LDCT screening--practical issues and technical considerations
One of the most important issues confronting those who wish to consider implementation of LDCT screening in high-risk populations is the problem of the high rate of positive examinations, primarily pulmonary nodules.
Nodule detection
Pulmonary nodules can be defined as rounded or irregular opacities, well or poorly defined, measuring up to 3 cm in diameter (18). There is inherent subjectivity in identifying nodules, reflected in inter- and intra-reader variability, even amongst experienced radiologists (19,20).
A considerable proportion of nodules may be missed at first reading and identified retrospectively at later scans (21). Nodule detection may be increased by using a second reader (22), image formatting, e.g., to maximum intensity projections (MIPs) (23-25) or by using computer aided detection (CAD) software as a “second reader” (26-28).
Nodule assessment
Nodules are best classified in four important ways: size, attenuation, presence/absence of calcification and, once a follow-up scan has been obtained, interval growth rate.
Size
Nodule size is the most important predictor for malignancy (Figure 1) (29-31). Detailed analysis of baseline NLST results found the positive predictive values (PPV) for malignancy increased significantly from 1.7% for nodules 7-10 mm in diameter to 11.9%, 29.7% and 41.3% for those 11-20, 21-30 and >30 mm diameter respectively (32). However even very small nodules (micronodules) have some risk of malignancy, e.g., 3 of 230 nodules <5 mm diameter (1.3%) at baseline scan followed for one year (33).
Attenuation
Certain calcification patterns and intra-nodular fat reliably indicate benignity (34), however, many nodules are too small to resolve internal features and are simply classified as ‘non-calcified’ nodules (NCNs). NCNs are common and detected in 25-50% of LDCT scans.
The majority of NCNs are of ‘solid’ (soft-tissue) radiological attenuation. The remainder are classified as non-solid nodules (NSNs) and subdivided into pure ground-glass (pGGO) or mixed (part-solid) attenuation nodules (solid and ground-glass components; psGGO). Synonyms vary between studies (Figure 2). The significance of GGOs is contentious as discussed below.
Ground glass opacities
The ELCAP study reported positive findings in 233/1,000 baseline scans. 19% of lesions were pGGO or psGGO (prevalence 4.4%; slice thickness 10 mm). Twenty-seven cancers were detected. After adjusting for size, the malignancy rate was 63% for psGGO, 32% for solid nodules and 13% for pGGOs (35). Other studies highlight the importance of a new or increasing solid component within NSNs, a finding highly suggestive of lung cancer (36-38). More recent studies demonstrate many NSNs spontaneously resolve. Felix (39) reported 75 GGOs in 37/280 patients (prevalence 13%; slice thickness 0.75 mm). The population was atypical for screening studies as over half had a history of lung or head and neck cancer. Approximately half the GGOs were present at baseline and half disappeared over a median 29 months follow-up. No morphological features allowed reliable discrimination between resolving and non-resolving GGOs. Kwon (40) reported 69 pGGO and 117 psGGO mostly detected by screening in 186 patients (total screenees not reported; slice thickness 5 mm). After 3 months, 45% regressed or disappeared. Malignant and benign lesions were similar in size (average 15-16 mm). Only 27% (33/122) were malignant but this may reflect a short follow-up time (mean 8.6 months; 64 lesions were still under active follow-up at publication). A second Korean study (41) identified 126 NSNs >5 mm diameter in 93 of 16,777 (0.5%) asymptomatic screenees. Forty-four had never smoked. 70% of NSNs were transient. Younger age, detection at a follow-up scan, blood eosinophilia, multiple lesions, larger solid component and ill-defined border independently predicted transiency. Mario (42) reported 76 NSNs retrospectively identified in 56/1,866 baseline screening scans in a high-risk screening cohort (prevalence 3%; slice thickness 0.75 mm) and followed for 50±7.3 months. Only 13 nodules were prospectively identified. 40 of 48 pGGOs (83%) resolved, decreased in size or remained stable. 16 of 28 psGGOs (57%) resolved or remained stable. Overall, 74% NSNs resolved, decreased in size or remained stable and 26% progressed. One psGGO (2%) was confirmed as lung adenocarcinoma.
In summary, perhaps as many as 50-70% of NSNs detected on modern thin-slice CT scans are transient but predicting which will persist is currently beyond our ability. The data suggest that a substantial difference in NSN prevalence between Western and Asian populations is unlikely. In view of slower growth rates for non-solid tumours (37,43) active surveillance for >2 years may be prudent for non-resolving NSNs (44).
Growth rate
Once a follow-up scan is obtained, assessment of growth can be made. Generally, absence of growth in a solid nodule over a 2 year period makes malignancy unlikely (45), although a contemporary review found the underpinning data (based on CXR studies from the 1950s) less than compelling (46).
Growth is best assessed by CT. For example, assuming exponential growth, a 5 mm diameter nodule with a volume doubling time (VDT) of 460 days will only increase to 6 mm diameter after one year and 7.2 mm after two years-changes which may not be measurable on CXR but which can be appreciated on CT. However, reproducible measurement is difficult: the 95% confidence intervals (CIs) for inter-reader measurements of nodules with a mean diameter of 8.5 mm were +/– 1.73 mm in one study (47). Semi-automated volumetric measurement using computer software may be more reproducible and accurate (48,49) and is the basis of nodule management in the NELSON trial (47-50). However even this is subject to error, e.g., with smaller nodules, in the presence of motion artefact (51), nodules attached to other structures and NSNs (52).
There are limited long-term data supporting the two year stability guideline for sub-centimetre NCNs; In an Irish study (53) 83 subjects with NCNs <10 mm stable over two years were imaged again at seven years. Virtually all nodules remained unchanged at the seven-year CT, however one 3 mm GGO grew to 15 mm in four years and was subsequently diagnosed as (what was previously called) bronchioloalveolar cell carcinoma. Thus ideally, the two year stability guideline suggested by CXR studies should be validated in larger, contemporary CT datasets.
The importance of baseline size and interval growth is shown in data from the NELSON study (54). 891 solid nodules 5-10 mm diameter were followed for one year. 743 nodules, all with smooth margins and/or attached to fissures, pleura, or vessels (contact length ≥50% of nodule diameter) were benign and excluded from multivariate analysis. Spiculated, irregular or lobulated nodules were analysed further. 10 of 69 (14.5%) nodules with spiculated or irregular margins and 6 of 168 (3.6%) nodules with lobulated margins were malignant. At baseline the only characteristic that predicted malignancy was volume ≥130 mm3 (OR 6.3; 95% CI: 1.7 to 23.0). At 3-months, baseline volume and VDT <400 days were significant (OR 4.9; 95% CI: 1.2 to 20.1 and OR 15.6; 95% CI: 4.5 to 53.5, respectively); At one year only VDT was predictive (OR 213.3; 95% CI: 18.7 to 2,430.9). Very few nodules showed change in margin or shape over 12 months, so these features were unable to distinguish malignant from benign nodules (55).
Other morphological features
Diederich (56), in a study of 133 consecutive resolving nodules, found the demographic and morphologic features of resolving and non-resolving nodules overlapped so greatly that none could be used to predict outcome over two years’ follow-up.
Features of benignity noted by Takashima after two years follow-up (72 nodules ≤10 mm diameter including 25 cancers) were polygonal shape, subpleural location, solid attenuation and elongation (higher long-axis-to-short-axis diameter ratio) (57). Long-term analysis of 234 similar nodules (perifissural with any of the following features: polygonal shape, long-axis-to-short-axis diameter ratio >1.78, peripheral location, vascular attachment) detected in 98/146 consecutive screenees found the nodules were multiple in half the subjects, ranged from 1-13 mm diameter, were mostly triangular or oval (86%), inferior to the carina (84%) and had a septal connection (73%) (58). 139 screenees were accounted for after 7.5 years, and none of the perifissural nodules had developed into cancers. These types of nodules most probably represent intrapulmonary lymph nodes, however histopathologic confirmation was not performed in either study (57,58).
The difficulty in predicting which nodules might be malignant is highlighted by low PPV in screening studies; with a cancer prevalence of 1-2% the PPV of a nodule designated by the radiologist as ‘suspicious’ or large in size or with VDT <400 days actually being malignant was only around 35% in two studies (50,59).
Nodule management protocols
LDCT nodule management protocols reflect the association of size and growth with malignancy. The protocols from the three largest studies, NLST, NELSON and I-ELCAP are summarized in Table 1 (52,60,61). These protocols have been applied to 26,722, 7,557 and 31,567 LDCT screenees respectively although I-ELCAP has no control arm. Size category definitions vary slightly, but in general terms ‘micronodules’ (usually less than 4-5 mm diameter) are followed after 12 months, large nodules (>10-15 mm diameter) are sent for immediate investigation and medium size nodules are followed-up to determine growth. Most studies use linear measurements of nodule size but the NELSON study uses volumetric measurement (50). Retrospective analysis of I-ELCAP data suggested the threshold to define a ‘positive’ baseline scan may be too inclusive; increasing the threshold to 7-8 mm (mean of maximal diameter and width) may reduce the false positive rate and subsequent work-up by 50-68% but at the cost of diagnostic delay for 5-6% of true positive cases (62). To date, only the NLST protocol has been proven to reduce lung cancer mortality.
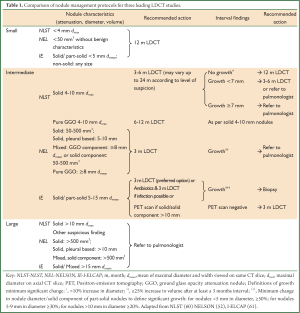
Full Table
Non-nodule (incidental) findings (IFs)
Non-cancer IFs such as coronary artery calcification (CAC), emphysema, and thyroid nodules are common but rates vary widely depending on study definitions and recording protocols. A NELSON substudy (n=1,929) found an IF rate of 81%. Six percent of participants received follow-up but only 1% had clinically important findings arguing against systematically searching for IFs (63). A Canadian study (n=4,073) found IFs in 19%; Approximately half would have required follow-up and 0.8% immediate action (64).
LDCT screening may be an opportunity to screen for other conditions which can be detected on chest CT such as CAC, chronic obstructive pulmonary disease (COPD) and osteoporosis (65,66). This may increase cost-effectiveness and provide better global outcomes but is currently untested. Radiologist-detected emphysema on CT scans appears to confer an independent increased risk of lung cancer (OR 2.1) (67) and may have the potential to help determine screening frequency following baseline scan (68) (i.e., more frequent screening for those with visually-detected emphysema), but this hypothesis remains to be tested.
CAC, a risk marker for cardiac events (69) is potentially the most important IF. Worldwide, smoking is estimated to cause 0.8 million deaths from acute heart attacks annually (70). The ELCAP investigators found varying degrees of CAC in 64% of 4,250 screenees (71). They developed a simple visual scoring system which was able to stratify cardiovascular death risk in a second cohort of 8,782 screenees followed for a median of six years (72). The NELSON study reported higher hazard ratios for all-cause mortality with increasing CAC in 958 participants followed for 21 months (73). However these findings do not appear to be reflected in NLST data where approximately 75% of all deaths were from non-lung cancer causes (13). Cardiovascular illness accounted for 486/1,865 (26.1%) deaths in the LDCT group and 470/1,991 (23.6%) in the CXR group. The 6.7% reduction in all-cause mortality in the LDCT group lost statistical significance when lung cancer deaths were removed from the comparison (3.2%, P=0.28) indicating that reduced lung cancer mortality was largely responsible for the reduction in all-cause mortality (13). Clinically significant IFs were identified in 7.5% of all scans and although details of CAC prevalence and follow-up are not yet reported, it seems unlikely that identification of CAC on LDCT screening made a significant impact on cardiovascular mortality in this study.
Thus IFs are common but mostly of little significance. Exhaustive investigation of IFs will increase the costs of screening through downstream investigation and follow-up, and should be accounted for in cost analyses. Further analysis of CAC and possibly other conditions in screening studies is warranted.
Screening by LDCT-effectiveness
Observational studies
The earliest LDCT screening studies were observational cohort studies from the USA and Japan (Table 2). CT appeared to be 3-4 times more sensitive than CXR in the ELCAP study, and the majority of tumours were stage I. Entry criteria were varied. Studies recruiting younger participants (<50 years old) and never-smokers had lower prevalence and/or incidence rates. For example, in a Japanese study (75) in which the majority of screenees had never smoked, cancer prevalence was only 0.4% compared to ELCAP 2.7% (31). These results underline the importance of recruiting a high-risk population. Subsequently, most studies follow the ELCAP strategy recruiting older persons with extensive smoking histories. Risk stratification is an area of current research interest and is discussed later.
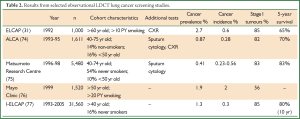
Full Table
Although very promising, these studies lacked control groups to allow estimation of mortality benefit. Survival, as a surrogate endpoint of effectiveness, is subject to several biases and cannot therefore be used to prove screening efficacy (Box 2). To add to the debate, studies modelling mortality benefit markedly diverged in their conclusions (78-80).
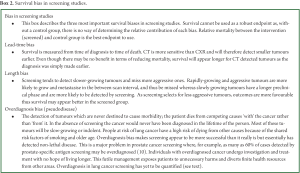
Full Table
Randomised controlled trials
The randomised control trials of LDCT screening are summarized in Table 3. Two trials, the NLST (USA) and NELSON (Holland/Belgium), have adequate statistical power to detect a reduction in lung cancer mortality. The smaller European studies are planning a meta-analysis (93). All European studies (except for Depiscan and DANTE) randomised LDCT screening against no screening, the current standard of care.
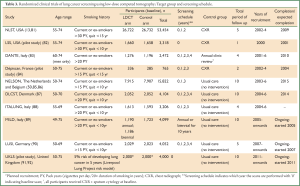
Full Table
The most important RCT result to date is from the NLST study (13). This landmark study randomised 53,454 high risk volunteers to three rounds of screening by CXR or LDCT (baseline, year 1 and year 2) and followed up for a median of 6.5 years. Eligibility criteria included: current or former smokers with ≥30 pack year smoking history (quit no more than 15 years previously); No history of lung or other cancer in the past five years; No current symptoms suggesting lung cancer; No chest CT in the previous 18 months. The study demonstrated a relative reduction in lung cancer-specific mortality of 20.0% in the LDCT arm (95% CI: 6.8 to 26.7; P=0.004).
Despite this positive result, several issues remain particularly generalizability and cost-effectiveness. The NLST authors stated their data alone are ‘insufficient’ to fully inform lung-cancer screening recommendations (13) and the Position Statement from the International Association for the Study of Lung Cancer (IASLC) Task Force on CT Screening reminds us that screening benefit, costs and potential harms must be defined in a ‘cultural context’, i.e., positive results seen in USA studies may not translate directly to other countries or healthcare systems (94). Additionally, the negative effects of screening and knowledge gaps, discussed below, must be considered.
Screening adherence
Good adherence is important to the success of mass screening. NLST reported 95% adherence across all three screening scans and NELSON reported 97% at year two. Long-term observational studies report 80% adherence at year five and 86% at year seven (76, 95). How this will translate to the ‘real world’ is not known.
Downstream healthcare use
Positive scans and incidental findings require clinical and radiological follow-up. Healthcare use may rise in the first six months following screening but return to baseline levels 6-12 months after screening and appears independent of result (i.e., negative, indeterminate or suspicious findings) (96). Although this study found doctor visits increased by 50%, in absolute terms this only meant one extra visit per participant (96).
Cost-effectiveness
Cost-effectiveness, a fundamental requirement of screening implementation, remains to be addressed. It depends on a complex mix of factors which vary from program to program and country to country (Table 4). Estimates vary widely depending on the underlying assumptions and models used, making conclusions difficult to draw (97). Using NLST data, Goulart estimated that if 75% of the eligible US population underwent screening, the cost to avoid one lung cancer death would be $240,000 (98). McMahon’s analysis paid particular attention to a model combining screening and smoking-cessation (99). The estimated cost per Quality Adjusted Life Year (QALY) in a cohort of 50 years old could be below $75,000/QALY if quit rates could be doubled from the background rate. From a health insurance perspective cost estimates were highly favourable (100); screening high-risk 50-64 years old would cost $1 per insured member per month, and the cost per life-year saved would be below $19,000.
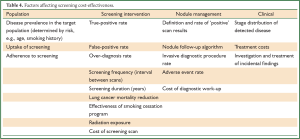
Full Table
To date, heterogeneous modelling methodologies and underlying assumptions have led to highly conflicting cost-effectiveness estimates. The final analysis from NLST has yet to be reported in a peer-reviewed format and is eagerly awaited. Preliminary data (101) suggest that it will be cost-effective with an Incremental Cost Effectiveness Ratio (ICER) of $72,900 US per QALY.
Negative effects of screening
Screening for any disease has risks and benefits. The balance helps determine overall effectiveness and acceptability of the screening program. The main negative effects are discussed below.
Radiation
It is generally accepted that ionising radiation is a cause of cancer without a lower “dose” threshold, although the absolute level of risk is debated (102,103). Minimising radiation dose according to the ALARA principle (‘as low as reasonably acceptable’) (104) is particularly important when screening asymptomatic, healthy subjects. CT radiation dose is determined by many factors including tube current, tube voltage, the use of filters and scan length (Z-axis). In screening studies, the most common way to limit dose is to adjust tube current (milliamperes, mA) (105) according to patient weight. This can degrade image quality as image noise (grainy mottling) is inversely proportional to the square root of the radiation dose. Fortunately the inherently high contrast between air-filled lung parenchyma and soft tissue lesions means pulmonary nodules are well-visualised. The mean effective dose from screening CT scans can be reduced from 8 mSv (standard CT chest) to approximately 1.5 mSv without significant deterioration in resolution or image quality (13,106,107). Although the lower radiation dose results in more noise it has been shown to provide adequate diagnostic pictures and is thus the current standard for screening (108-110). Total radiation dose can be further limited by restricting the scope of follow-up CTs to a region of interest surrounding the nodule(s) in question rather than covering the entire chest, so-called ‘limited’ LDCT (111).
Smoking appears to interact synergistically with ionising radiation. In absolute terms the risk of cancer from LDCT is small, perhaps only an excess lifetime risk of 0.85% (95% CI: 0.28% to 2.2%) for the worst case scenario of a 50-year-old female smoker receiving 25 annual LDCT scans. This compares to a 17% risk of developing lung cancer (112). Berrington de Gonzalez estimated the cumulative risk of excess death from lung cancer from LDCT screening in 50-year-old smokers to be 2 per 10,000 men screened and 5 per 10,000 women screened. Additionally an estimated 3 cases of breast cancer per 10,000 women screened may occur (113). The NLST estimated the number needed to screen (NNS) to prevent one death from lung cancer was 320, equating to a rate of 30 fewer deaths per 10,000 screenees (13) a larger benefit than the radiation harm particularly as the cancers induced occur after a delay of many years and the lives saved are over the short term. Estimates from the ITALUNG RCT reached similar conclusions with an estimated 1.1 excess deaths per 10,000 screenees compared to approximately 15-100 lives saved per 10,000 screenees (women and men respectively) assuming a 20% mortality reduction from screening (114). Thus the radiation risk-benefit ratio of LDCT screening appears quite favourable in older populations of smokers.
Adverse events
Adverse events may result from investigation of LDCT findings. As 25-50% of screenees may have one or more nodules detected, a potentially large reservoir of patients at risk exists. In the NLST the cumulative chance of a positive screening scan was 39.1%.
Despite guidelines (115), significant variation in pulmonary nodule biopsy rates (14.7 to 36.2 per 100,000 adults) and complication rates have been found between hospitals in the USA (116). The risk of haemorrhage and pneumothorax requiring intercostal catheter drainage (ICC) were 1.0% and 6.6% respectively. Complications were associated with an increased length of stay and risk of respiratory failure. Those at highest risk were smokers, persons aged 60 to 69 years, and those with COPD, i.e., the types of patients targeted for screening. LDCT screening study adverse event rates may be slightly higher than the above study but this probably reflects more rigorous, prospective reporting. There appears to be no standard way of defining or reporting adverse event data which makes some studies difficult to compare directly. ‘Number of events per 10,000 scans’ may be a useful metric to allow cross-study comparison.
A study of 4,782 participants (117) screened using the I-ELCAP protocol reported a biopsy rate of 2.6% (n=127) including 110 percutaneous CT-guided fine-needle aspiration biopsies (CT-FNA). 13% of CT-FNAs were complicated by a moderate-to-large pneumothorax requiring ICC or hospitalization. Overall 16% of biopsies were for benign disease (117). Using a volumetric-based protocol, NELSON reported the surgical diagnostic procedure rate as 1.2% in round one and 0.8% in round two; 32/92 (35%) and 13/61 (21%) procedures in each round were for benign disease. Very few CT-FNAs were performed: 5/13 CT-FNA in round one and 3/3 FNA in round 2 showed benign disease. Across both rounds bronchoscopy diagnosed cancer in 111/247 (45%) procedures-a lower than expected figure likely reflecting peripheral tumour location. Complication rates were not reported (50).
The PLuSS study (118) screened 3,642 participants using an in-house protocol. 82 (2.3%) underwent surgical procedures (thoracotomy or VATS), twenty-eight of whom (34%) had benign disease. The study investigators cited “an apparent community bias toward aggressive intervention” for indeterminate lung nodules.
At baseline, 27.3% in the NLST LDCT group had a positive scan result (13). 155/7,191 participants had a percutaneous diagnostic procedure (CT-FNA in 120) and 297 (4.1% of positive scans) had a diagnostic surgical procedure (thoracotomy, thoracoscopy, mediastinoscopy or mediastinotomy) including 197 thoracotomies. Across all three screening rounds (75, 126 screenings), 164/673 (24%) of surgical procedures in the LDCT group resulted in a non-cancer diagnosis. 191/673 (29%) of participants whose most invasive diagnostic procedure was surgical experienced at least one complication; in 80 (12%) this was classified as major. Only 14 of 99 (14%) participants who underwent a needle biopsy as their most invasive diagnostic procedure experienced one or more complication and none were major. 16 participants (10 with lung cancer) died within 60 days of an invasive diagnostic procedure, but it is not known whether death resulted directly from the diagnostic procedure. Put differently, 33 per 10,000 screenees suffered major complications during any diagnostic evaluation, but complications following bronchoscopy or needle biopsy were low, 1.5 and 0.7 per 10,000 screenees respectively; the frequency of death occurring within 2 months of a diagnostic evaluation was 8 per 10,000 (16). I-ELCAP has not reported its rates of diagnostic procedures or complications.
CT-FNA appears safe with a complication rate of 13-14% and good concordance of biopsy result with resected pathological specimens histology (119). Bronchoscopy on the other hand, although safe, may have a lower yield for small, peripheral cancers detected by screening, although newer techniques such as endobronchial ultrasound and electromagnetic navigation may be able to improve yield (120,121). Surgical procedures have major complication rates of 12% but around 20-35% of cases are ultimately diagnosed with benign disease. This has an impact on cost-effectiveness.
Although ultimately the decision to resect an indeterminate nodule is a clinical one, given the high proportion of reported benign disease detected by screening, a positive tissue diagnosis prior to surgical resection is desirable. As demonstrated by the NELSON study, definite growth over a three month interval was due to benign disease in up to one third of cases. To date most studies have been run from expert tertiary centres where CT-FNA is available as the initial diagnostic procedure for small peripheral lesions. It is likely that strict governance and quality assurance will be needed to keep unnecessary biopsies and resections to a minimum.
Lung-preserving surgery
As reviewed by Blasburg et al., evolving surgical technique, the recognition of good prognosis for small tumours, especially with a high GGO component, and the on-going risk of subsequent tumours, has turned attention to ‘lung preserving’ surgery (anatomical segmentectomy and wedge resection) as an alternative to lobar resection for small tumours (122). Two randomized controlled trials which will hopefully be able to answer this important question are currently recruiting [CALGB 140503 and JCOG0802/WJOG4607L (123)].
Quality of Life (QoL)
Three studies have reported generic health-related QoL (HRQoL), anxiety and lung-cancer specific distress data from approximately 2,500 screening participants (124-126). All found some transient negative psychological effects for participants who received an indeterminate or suspicious screening result. These effects subsided fairly rapidly such that there were no significant differences in HRQoL between baseline and 12-24 months follow-up. The NELSON study reported that half the participants found waiting for their baseline CT scan results ‘discomforting’, but that an indeterminate result at the second round of screening had no impact on HRQoL. This suggests that minimizing the waiting time for test results is beneficial and that participants soon accept that an indeterminate scan result does not necessarily warrant high anxiety (124,127).
Smoking cessation
Smoking cessation is important not only for future risk reduction in participants without cancer, but may also improve the prognosis of those diagnosed with early stage lung cancer (128). Screening for lung cancer may be a “teachable moment” increasing motivation to quit, particularly if the participant receives an abnormal CT scan report (129-131). As successful smoking cessation programs may also make screening more cost-effective (99), and smoking cessation assistance ‘adds value’ to screening in several ways, it should be a core component of any lung cancer screening program.
Knowledge gaps
Despite the positive result from NLST, screening outside of a research trial should be conducted in a controlled environment with careful risk assessment prior to recommending screening and careful analysis of all outcomes to ensure quality. Two international workshops have considered the current state of evidence and future directions for research. Areas that need addressing were highlighted including: (I) how to optimise identification of high-risk individuals; (II) Screening protocols (e.g., screen interval, number of screening rounds); (III) Definition of a positive screen result; (IV) Management of indeterminate nodules; (V) Diagnostic and therapeutic interventions for suspicious nodules; (VI) Integrated smoking cessation programs; (VII) The role of early detection biomarkers in individual lung cancer risk assessment; (VIII) The rate of overdiagnosis. Important steps will be to standardise equipment and image quality, nodule analysis and interpretation, and participant follow-up and outcome reporting (93,132). Some of these areas are discussed below.
Overdiagnosis
Overdiagnosis is difficult to ascertain (see
Screening interval and length of follow-up
The appropriate screening interval should provide a favourable ratio between disease control and screening costs (134). The MILD trial recently published their findings from a three-arm RCT of observation vs. annual vs. biennial screening in 4,099 participants (89). Stage distribution and resection rates were similar in the two LDCT arms. The cumulative 5-year lung cancer incidence was highest in the annual LDCT group compared to biennial and control groups (620/100,000 vs. 457 and 311 respectively, P=0.036). Adherence to the screening protocol was >95% in each LDCT arm but median duration of follow-up was only 4.4 years. Recruitment fell significantly short of the planned 10,000 participants meaning the study was underpowered to detect mortality differences. Also, differences in characteristics of screened and non-screened groups (such as smoking status, smoking intensity and lung function) raise doubts about the adequacy of randomization (135). Long-term follow-up results from this study may be more informative. The NELSON study, in which participants are screened at Year 1 (baseline), Year 2 and Year 4, i.e., a two-year gap between the second and third scan, could also inform on optimal screen interval when Year 4 results are reported. As previously mentioned, data gathered at baseline scan (i.e., presence of radiographic emphysema) may be useful in determining risk and thus optimal screening interval (68). Regarding duration of screening, the NLST LDCT arm detected 649 cancers after a positive screening test (270 at baseline and 168 and 211 at years1 and 2 respectively) and 367 in participants who either missed the screening or were diagnosed after completing the trial screening phase (median follow-up 6.5 years). This suggests that cancer detection rates (i.e., cancer risk) do not drop significantly over time and that on-going screening may be required. Accordingly, current guidelines suggest annual screening until the age of 74 (14,16) or 79 (15).
Recruitment
Recruitment strategies have varied between studies, most commonly direct mailing and/or media releases, but some used general practitioner referral (84,88). Smokers, by definition are less risk averse than non-smokers, at least in terms of their health. The decision to enter a screening trial is a complex balance of factors including acceptability of screening methods, risk perception, altruism, and self-interest (136). Inevitably, volunteers in any trial are self-selected and contribute to the ‘healthy volunteer’ effect. This may result in overly optimistic outcomes (e.g., better screening compliance, higher smoking cessation rates) or overly pessimistic outcomes (e.g., lower effectiveness as lower-risk individuals benefit less from screening).
Both the NLST and NELSON studies found some differences between their study populations and eligible general population; Participants were younger and less likely to be current smokers and had higher education levels (a proxy for socio-economic status). These differences were considered minor, meaning that a significant healthy volunteer effect was unlikely (81,137).
Risk stratification
Risk stratification has been applied at a basic level with most studies adopting the ELCAP strategy of screening older persons with a smoking history. Although age and tobacco smoke exposure account for the vast majority of lung cancer risk it is well recognised that other risk factors such as family history, socioeconomic status, occupational exposure and COPD contribute (138). Further risk stratification using other readily available information may be able to improve screening efficiency by excluding lower risk participants (139). Various models have been proposed, the largest derived from PLCO Trial data and recently updated (140,141). A retrospective analysis of this model applied to the PLCO dataset found that it was more efficient in comparison to the standard age- and smoking-based NLST entry criteria improving sensitivity from 71% to 83% (P<0.001), positive predictive value from 3.4% to 4.0% (P=0.01), and maintaining specificity (63% each). Use of the risk model to select screenees would have missed 41.3% fewer lung cancers (141). Prospective evaluation of another risk model is being undertaken by the UK Lung Cancer Screening Trial (142). Risk stratification may enhance screening effectiveness and cost-effectiveness by increasing lung cancer prevalence and incidence and reducing false-positive scan results. Although risk stratification makes intuitive sense it has not been proven experimentally, thus screening guideline recommendations diverge [recommend use of published risk model (15), informal risk assessment (14), no recommendation (16)].
Screening implementation
Generalization of findings from tightly controlled trial situations to large-scale mass screening programs require uniform standards and high quality control in order to be able to accurately track and assess nodules over time (132). Lung cancer screening is more than simple provision of a CT service; It is as a long-term commitment requiring extensive infrastructure to allow for invitation and recruitment; quality improvement; workforce/facility capacity for screening, diagnosis and treatment; health professional training; participant information and support. On-going evaluation and monitoring of the program is essential to ensure high standards of care are met and delivered in a consistent and acceptable way (134,143).
Future research
Minimally invasive, inexpensive tests to identify individuals at highest risk of lung cancer most likely to benefit from screening or to distinguish benign from malignant screen-detected nodules would represent major advances in lung cancer screening. Promising new technologies in this regard include analysis of blood for circulating microRNAs and exhaled breath for volatile organic compounds (144-146). Most recent LDCT screening studies included biomarker collection in their protocols, so we can expect exciting new insights into these areas in the near future.
Conclusions
The results of the landmark NLST have proven the long-held belief that screening for lung cancer can save lives. Understandably, as a new intervention, many questions remain making generalizability to non-US settings difficult. Over the next few years, further analysis of NLST data and maturation of other important trials will be able to fill these knowledge gaps allowing the lung cancer community to evolve and refine the way we screen.
Acknowledgements
We thank the study volunteers and staff of The Prince Charles Hospital for their involvement in our research program and the Queensland Lung Cancer Screening Study research team.
Funding: This work was supported by National Health and Medical Research Council (NHMRC) project grants, NHMRC Practitioner Fellowship (KF), NHMRC Career Development Award (IY), Cancer Council Queensland Senior Research Fellowship (KF), Cancer Council Queensland project grants, Queensland Smart State project grants, Office of Health and Medical Research (OHMR) project grants, The Prince Charles Hospital Foundation, NHMRC Postgraduate Medical Scholarship (HM), University of Queensland PhD Scholarship (HM).
Disclosure: The authors declare no conflict of interest.
References
- Ferlay J, Shin H, Bray F, et al. GLOBOCAN 2008. Available online:
- Jemal A, Bray F, Center MM, et al. Global cancer statistics. CA Cancer J Clin 2011;61:69-90. [PubMed]
- Gu D, Kelly TN, Wu X, et al. Mortality attributable to smoking in China. N Engl J Med 2009;360:150-9. [PubMed]
- Sant M, Allemani C, Santaquilani M, et al. EUROCARE-4. Survival of cancer patients diagnosed in 1995-1999. Results and commentary. Eur J Cancer 2009;45:931-91. [PubMed]
- Howlader N, Noone AM, Krapcho M, et al. SEER cancer statistics review, 1975-2008. Available online:
- Sankaranarayanan R, Swaminathan R, Brenner H, et al. Cancer survival in Africa, Asia, and Central America: a population-based study. Lancet Oncol 2010;11:165-73. [PubMed]
- Scott WJ, Howington J, Feigenberg S, et al. Treatment of non-small cell lung cancer stage I and stage II*. Chest 2007;132:234S-242S. [PubMed]
- Goldstraw P, Crowley J, Chansky K, et al. The IASLC Lung Cancer Staging Project: proposals for the revision of the TNM stage groupings in the forthcoming (seventh) edition of the TNM classification of malignant tumours. J Thorac Oncol 2007;2:706-14. [PubMed]
- Wilson JMG, Jungner G. eds. Principles and practice of screening for disease. Geneva: World Health Organization, 1968.
- Welch HG, Black WC. Overdiagnosis in Cancer. J Natl Cancer Inst 2010;102:605-13. [PubMed]
- Manser RL, Irving LB, Stone C, et al. Screening for lung cancer. Cochrane Cochrane Database Syst Rev 2004;(1):CD001991.
- Oken MM, Hocking WG, Kvale PA, et al. Screening by chest radiograph and lung cancer mortality. JAMA 2011;306:1865-73. [PubMed]
- National Lung Screening Trial Research Team. Reduced lung-cancer mortality with low-dose computed tomographic screening. N Engl J Med 2011;365:395-409. [PubMed]
- Wood DE, Eapen GA, Ettinger DS, et al. Lung cancer screening. J Natl Compr Canc Netw 2012;10:240-65. [PubMed]
- Jaklitsch MT, Jacobson FL, Austin JH, et al. The American Association for Thoracic Surgery guidelines for lung cancer screening using low-dose computed tomography scans for lung cancer survivors and other high-risk groups. J Thorac Cardiovasc Surg 2012;144:33-8. [PubMed]
- Bach PB, Mirkin JN, Oliver TK, et al. Benefits and harms of CT screening for lung cancer: a systematic review. JAMA 2012;307:2418-29. [PubMed]
- Force UPST. US preventive services task force. Draft recommendation statement. Available online:
- Hansell DM, Bankier A, MacMahon H, et al. Fleischner society: glossary of terms for thoracic imaging. Radiology 2008;246:697-722. [PubMed]
- Armato SG, Roberts RY, Kocherginsky M, et al. Assessment of radiologist performance in the detection of lung nodules: dependence on the definition of “truth”. Acad Radiol 2009;16:28-38. [PubMed]
- Gierada DS, Pilgram TK, Ford M, et al. Lung cancer: interobserver agreement on interpretation of pulmonary findings at low-dose CT screening. Radiology 2008;246:265-72. [PubMed]
- Swensen SJ, Jett JR, Sloan JA, et al. Screening for lung cancer with low-dose spiral computed tomography. Am J Respir Crit Care Med 2002;165:508-13. [PubMed]
- Wormanns D, Ludwig K, Beyer F, et al. Detection of pulmonary nodules at multirow-detector CT: effectiveness of double reading to improve sensitivity at standard-dose and low-dose chest CT. Eur Radiol 2005;15:14-22. [PubMed]
- Jankowski A, Martinelli T, Timsit JF, et al. Pulmonary nodule detection on MDCT images: evaluation of diagnostic performance using thin axial images, maximum intensity projections, and computer-assisted detection. Eur Radiol 2007;17:3148-56. [PubMed]
- Coakley FV, Cohen MD, Johnson MS, et al. Maximum intensity projection images in the detection of simulated pulmonary nodules by spiral CT. Br J Radiol 1998;71:135-40. [PubMed]
- Kawel N, Seifert B, Luetolf M, et al. Effect of slab thickness on the CT detection of pulmonary nodules: use of sliding thin-slab maximum intensity projection and volume rendering. AJR Am J Roentgenol 2009;192:1324-9. [PubMed]
- Armato SG 3rd, Li F, Giger ML, et al. Lung cancer: performance of automated lung nodule detection applied to cancers missed in a CT screening program. Radiology 2002;225:685-92. [PubMed]
- Yuan R, Vos PM, Cooperberg PL. Computer-aided detection in screening CT for pulmonary nodules. AJR Am J Roentgenol 2006;186:1280-7. [PubMed]
- Saba L, Caddeo G, Mallarini G. Computer-aided detection of pulmonary nodules in computed tomography: analysis and review of the literature. J Comput Assist Tomogr 2007;31:611-9. [PubMed]
- Midthun DE, Swensen SJ, Jett JR, et al. Evaluation of nodules detected by screening for lung cancer with low dose spiral computed tomography. Lung Cancer 2003;41:S40.
- Gohagan J, Marcus P, Fagerstrom R, et al. Baseline findings of a randomized feasibility trial of lung cancer screening with spiral CT scan vs chest radiograph: the Lung Screening Study of the National Cancer Institute. Chest 2004;126:114-21. [PubMed]
- Henschke CI, McCauley DI, Yankelevitz DF, et al. Early Lung Cancer Action Project: overall design and findings from baseline screening. Lancet 1999;354:99-105. [PubMed]
- The National Lung Screening Trial Research Team. Results of initial low-dose computed tomographic screening for lung cancer. N Engl J Med 2013;368:1980-91. [PubMed]
- Bellomi M, Veronesi G, Rampinelli C, et al. Evolution of lung nodules < or =5 mm detected with low-dose CT in asymptomatic smokers. Br J Radiol 2007;80:708-12. [PubMed]
- Erasmus JJ, Connolly JE, McAdams HP, et al. Solitary pulmonary nodules: part I. morphologic evaluation for differentiation of benign and malignant lesions. Radiographics 2000;20:43-58. [PubMed]
- Henschke CI, Yankelevitz DF, Mirtcheva R, et al. CT screening for lung cancer: frequency and significance of part-solid and nonsolid nodules. Am J Roentgenol 2002;178:1053-7. [PubMed]
- Kakinuma R, Ohmatsu H, Kaneko M, et al. Progression of focal pure ground-glass opacity detected by low-dose helical computed tomography screening for lung cancer. J Comput Assist Tomogr 2004;28:17-23. [PubMed]
- Lindell RM, Hartman TE, Swensen SJ, et al. Five-year lung cancer screening experience: CT appearance, growth rate, location, and histologic features of 61 lung cancers. Radiology 2007;242:555-62. [PubMed]
- Aoki T, Nakata H, Watanabe H, et al. Evolution of peripheral lung adenocarcinomas: CT findings correlated with histology and tumor doubling time. AJR Am J Roentgenol 2000;174:763-8. [PubMed]
- Felix L, Serra-Tosio G, Lantuejoul S, et al. CT characteristics of resolving ground-glass opacities in a lung cancer screening programme. Eur J Radiol 2011;77:410-6. [PubMed]
- Oh JY, Kwon SY, Yoon HI, et al. Clinical significance of a solitary ground-glass opacity (GGO) lesion of the lung detected by chest CT. Lung Cancer 2007;55:67-73. [PubMed]
- Lee SM, Park C, Goo J, et al. Transient part-solid nodules detected at screening thin-section CT for lung cancer: comparison with persistent part-solid nodules. Radiology 2010;255:242-51. [PubMed]
- Mario M, Nicola S, Carmelinda M, et al. Long-term surveillance of ground-glass nodules: evidence from the mild trial. J Thorac Oncol 2012;7:1541-6. [PubMed]
- Hasegawa M, Sone S, Takashima S, et al. Growth rate of small lung cancers detected on mass CT screening. Br J Radiol 2000;73:1252-9. [PubMed]
- Godoy MC, Naidich DP. Overview and strategic management of subsolid pulmonary nodules. J Thorac Imaging 2012;27:240-8. [PubMed]
- MacMahon H, Austin JH, Gamsu G, et al. Guidelines for management of small pulmonary nodules detected on CT scans: a statement from the Fleischner Society. Radiology 2005;237:395-400. [PubMed]
- Yankelevitz DF, Henschke CI. Does 2-year stability imply that pulmonary nodules are benign? AJR Am J Roentgenol 1997;168:325-8. [PubMed]
- Revel MP, Bissery A, Bienvenu M, et al. Are two-dimensional CT measurements of small noncalcified pulmonary nodules reliable? Radiology 2004;231:453-8. [PubMed]
- Revel MP, Lefort C, Bissery A, et al. Pulmonary nodules: preliminary experience with three-dimensional evaluation. Radiology 2004;231:459-66. [PubMed]
- Yankelevitz DF, Reeves AP, Kostis WJ, et al. Small pulmonary nodules: volumetrically determined growth rates based on CT evaluation. Radiology 2000;217:251-6. [PubMed]
- van Klaveren RJ, Oudkerk M, Prokop M, et al. Management of lung nodules detected by volume CT scanning. N Engl J Med 2009;361:2221-9. [PubMed]
- Kostis WJ, Yankelevitz D, Reeves A, et al. Small pulmonary nodules: reproducibility of three-dimensional volumetric measurement and estimation of time to follow-up CT. Radiology 2004;231:446-52. [PubMed]
- Xu DM, Gietema H, de Koning H, et al. Nodule management protocol of the NELSON randomised lung cancer screening trial. Lung Cancer 2006;54:177-84. [PubMed]
- Slattery MM, Foley C, Kenny D, et al. Long-term follow-up of non-calcified pulmonary nodules (<10 mm) identified during low-dose CT screening for lung cancer. Eur Radiol 2012;22:1923-8. [PubMed]
- Xu DM, van der Zaag-Loonen HJ, Oudkerk M, et al. Smooth or attached solid indeterminate nodules detected at baseline CT screening in the NELSON study: cancer risk during 1 year of follow-up. Radiology 2009;250:264-72. [PubMed]
- Xu DM, van Klaveren RJ, de Bock GH, et al. Role of baseline nodule density and changes in density and nodule features in the discrimination between benign and malignant solid indeterminate pulmonary nodules. Eur J Radiol 2009;70:492-8. [PubMed]
- Diederich S, Hansen J, Wormanns D. Resolving small pulmonary nodules: CT features. Eur Radiol 2005;15:2064-9. [PubMed]
- Takashima S, Sone S, Li F, et al. Small solitary pulmonary nodules (1 cm) detected at population-based CT screening for lung cancer: reliable high-resolution CT features of benign lesions. AJR Am J Roentgenol 2003;180:955-64. [PubMed]
- Ahn MI, Gleeson TG, Chan IH, et al. Perifissural nodules seen at CT screening for lung cancer. Radiology 2010;254:949-56. [PubMed]
- Markowitz SB, Miller A, Miller J, et al. Ability of low-dose helical CT to distinguish between benign and malignant noncalcified lung nodules. Chest 2007;131:1028-34. [PubMed]
- ACRIN. ACRIN Protocol. Available online:
- International Early Lung Cancer Action Program. Enrollment and screening protocol. Available online:
- Henschke CI, Yip R, Yankelevitz DF, et al. Definition of a positive test result in computed tomography screening for lung cancer: a cohort study. Ann Intern Med 2013;158:246-52. [PubMed]
- van de Wiel JC, Wang Y, Xu DM, et al. Neglectable benefit of searching for incidental findings in the Dutch-Belgian lung cancer screening trial (NELSON) using low-dose multidetector CT. Eur Radiol 2007;17:1474-82. [PubMed]
- Kucharczyk MJ, Menezes RJ, McGregor A, et al. Assessing the impact of incidental findings in a lung cancer screening study by using low-dose computed tomography. Can Assoc Radiol J 2011;62:141-5. [PubMed]
- Mets OM, de Jong PA, Prokop M. Computed tomographic screening for lung cancer: an opportunity to evaluate other diseases. JAMA 2012;308:1433-4. [PubMed]
- Patel AR, Wedzicha J, Hurst J. Reduced lung-cancer mortality with CT screening. N Engl J Med 2011;365:2035-author reply 2037-8. [PubMed]
- Smith BM, Pinto L, Ezer N, et al. Emphysema detected on computed tomography and risk of lung cancer: a systematic review and meta-analysis. Lung Cancer 2012;77:58-63. [PubMed]
- Maisonneuve P, Bagnardi V, Bellomi M, et al. Lung cancer risk prediction to select smokers for screening CT--a model based on the Italian COSMOS trial. Cancer Prev Res (Phila) 2011;4:1778-89. [PubMed]
- Waugh N, Black C, Walker S, et al. The effectiveness and cost-effectiveness of computed tomography screening for coronary artery disease: systematic review. Health Technol Assess 2006;10:iii-iv, ix-x, 1-41.
- Jha P. Avoidable global cancer deaths and total deaths from smoking. Nat Rev Cancer 2009;9:655-64. [PubMed]
- Shemesh J, Henschke CI, Farooqi A, et al. Frequency of coronary artery calcification on low-dose computed tomography screening for lung cancer. Clin Imaging 2006;30:181-5. [PubMed]
- Shemesh J, Henschke CI, Shaham D, et al. Ordinal scoring of coronary artery calcifications on low-dose CT scans of the chest is predictive of death from cardiovascular disease. Radiology 2010;257:541-8. [PubMed]
- Jacobs PC, Gondrie MJ, van der Graaf Y, et al. Coronary artery calcium can predict all-cause mortality and cardiovascular events on low-dose ct screening for lung cancer. AJR Am J Roentgenol 2012;198:505-11. [PubMed]
- Sobue T, Moriyama N, Kaneko M, et al. Screening for lung cancer with low-dose helical computed tomography: anti-lung cancer association project. J Clin Oncol 2002;20:911-20. [PubMed]
- Sone S, Nakayama T, Honda T, et al. Long-term follow-up study of a population-based 1996-1998 mass screening programme for lung cancer using mobile low-dose spiral computed tomography. Lung Cancer 2007;58:329-41. [PubMed]
- Swensen SJ, Jett JR, Hartman TE, et al. CT screening for lung cancer: five-year prospective experience. Radiology 2005;235:259-65. [PubMed]
- The International Early Lung Cancer Action Program I, Henschke CI, Yankelevitz D, et al. Survival of patients with stage I lung cancer detected on CT screening. N Engl J Med 2006;355:1763-71. [PubMed]
- Bach PB, Jett JR, Pastorino U, et al. Computed tomography screening and lung cancer outcomes. JAMA 2007;297:953-61. [PubMed]
- Patz EF Jr, Swensen SJ, Herndon JE. Estimate of lung cancer mortality from low-dose spiral computed tomography screening trials: implications for current mass screening recommendations. J Clin Oncol 2004;22:2202-6. [PubMed]
- Foy M, Yip R, Chen X, et al. Modeling the mortality reduction due to computed tomography screening for lung cancer. Cancer 2011;117:2703-8. [PubMed]
- National Lung Screening Trial Research Team, Aberle DR, Adams AM, et al. Baseline characteristics of participants in the randomized national lung screening trial. J Natl Cancer Inst 2010;102:1771-9. [PubMed]
- Gohagan JK, Marcus PM, Fagerstrom RM, et al. Final results of the lung screening study, a randomized feasibility study of spiral CT versus chest X-ray screening for lung cancer. Lung Cancer 2005;47:9-15. [PubMed]
- Infante M, Cavuto S, Lutman FR, et al. A randomized study of lung cancer screening with spiral computed tomography: three-year results from the DANTE trial. Am J Respir Crit Care Med 2009;180:445-53. [PubMed]
- Blanchon T, Brechot JM, Grenier PA, et al. Baseline results of the Depiscan study: a French randomized pilot trial of lung cancer screening comparing low dose CT scan (LDCT) and chest X-ray (CXR). Lung Cancer 2007;58:50-8. [PubMed]
- van Iersel CA, de Koning HJ, Draisma G, et al. Risk-based selection from the general population in a screening trial: selection criteria, recruitment and power for the Dutch-Belgian randomised lung cancer multi-slice CT screening trial (NELSON). Int J Cancer 2007;120:868-74. [PubMed]
- Baecke E, de Koning HJ, Otto SJ, et al. Limited contamination in the Dutch-Belgian randomized lung cancer screening trial (NELSON). Lung Cancer 2010;69:66-70. [PubMed]
- Pedersen JH, Ashraf H, Dirksen A, et al. The Danish randomized lung cancer CT screening trial--overall design and results of the prevalence round. J Thorac Oncol 2009;4:608-14. [PubMed]
- Lopes Pegna A, Picozzi G, Mascalchi M, et al. Design, recruitment and baseline results of the ITALUNG trial for lung cancer screening with low-dose CT. Lung Cancer 2009;64:34-40. [PubMed]
- Pastorino U, Rossi M, Rosato V, et al. Annual or biennial CT screening versus observation in heavy smokers: 5-year results of the MILD trial. Eur J Cancer Prev 2012;21:308-15. [PubMed]
- Becker N, Motsch E, Gross ML, et al. Randomized study on early detection of lung cancer with MSCT in Germany: study design and results of the first screening round. Journal of cancer research and clinical oncology 2012;138:1475-86. [PubMed]
- Field JK, Baldwin D, Brain K, et al. CT screening for lung cancer in the UK: position statement by UKLS investigators following the NLST report. Thorax 2011;66:736-7. [PubMed]
- NIHR. Health technology assessment programme. Available online:
- Italian lung cancer CT screening trial workshop. International workshop on randomized lung cancer screening trials; Position statement, Pisa, March 3-4. 2011. Available online:
- International Association for the Study of Lung Cancer. IASLC task force on CT screening: position statement. 2011 [cited 01/12/2011]. Available online:
- Veronesi G, Maisonneuve P, Spaggiari L, et al. Long-term outcomes of a pilot CT screening for lung cancer. Ecancermedicalscience 2010;4:186. [PubMed]
- Byrne MM, Koru-Sengul T, Zhao W, et al. Healthcare use after screening for lung cancer. Cancer 2010;116:4793-9. [PubMed]
- Black C, Bagust A, Boland A, et al. The clinical effectiveness and cost-effectiveness of computed tomography screening for lung cancer: systematic reviews. Health Technol Assess 2006;10:iii-iv, ix-x, 1-90.
- Goulart BHL, Bensink ME, Mummy DG, et al. Lung cancer screening with low-dose computed tomography: costs, national expenditures, and cost-effectiveness. J Natl Compr Canc Netw 2012;10:267-75. [PubMed]
- McMahon PM, Kong CY, Bouzan C, et al. Cost-effectiveness of computed tomography screening for lung cancer in the United States. J Thorac Oncol 2011;6:1841-8. [PubMed]
- Pyenson BS, Sander MS, Jiang Y, et al. An actuarial analysis shows that offering lung cancer screening as an insurance benefit would save lives at relatively low cost. Health Aff (Millwood) 2012;31:770-9. [PubMed]
- Black WC. Cost Effectiveness of CT Screening in the National Lung Screening Trial, in 2nd Joint Meeting of the National Cancer Institute Board of Scientific Advisors & National Cancer Advisory Board 2013: Bethseda (Accessed 19 Aug 2013, at ).
- Berrington de González A, Darby S. Risk of cancer from diagnostic X-rays: estimates for the UK and 14 other countries. Lancet 2004;363:345-51. [PubMed]
- Herzog P, Rieger CT. Risk of cancer from diagnostic X-rays. Lancet 2004;363:340-41. [PubMed]
- ICRP. Recommendations of the ICRP. Annals of the ICRP 1977;1:3.
- Kubo T, Lin P-JP, Stiller W, et al. Radiation dose reduction in chest CT: a review. AJR Am J Roentgenol 2008;190:335-43. [PubMed]
- Marshall H, Smith I, Keir B, et al. Radiation dose during lung cancer screening with low-dose computed tomography: how low is low dose? J Thorac Oncol 2010;6.
- Mascalchi M, Mazzoni LN, Falchini M, et al. Dose exposure in the ITALUNG trial of lung cancer screening with low-dose CT. Br J Radiol 2012;85:1134-9. [PubMed]
- Takahashi M, Maguire WM, Ashtari M, et al. Low-dose spiral computed tomography of the thorax: comparison with the standard-dose technique. Invest Radiol 1998;33:68-73. [PubMed]
- Mayo JR, Hartman TE, Lee KS, et al. CT of the chest: minimal tube current required for good image quality with the least radiation dose. AJR Am J Roentgenol 1995;164:603-7. [PubMed]
- Diederich S, Lenzen H, Windmann R, et al. Pulmonary nodules: experimental and clinical studies at low-dose CT. Radiology 1999;213:289-98. [PubMed]
- Marshall HM, Bowman RV, Crossin J, et al. Queensland lung cancer screening study: rationale, design and methods. Intern Med J 2013;43:174-82. [PubMed]
- Brenner DJ. Radiation risks potentially associated with low-dose CT screening of adult smokers for lung cancer. Radiology 2004;231:440-5. [PubMed]
- Berrington de González A, Kim KP, Berg CD. Low-dose lung computed tomography screening before age 55: estimates of the mortality reduction required to outweigh the radiation-induced cancer risk. J Med Screen 2008;15:153-8. [PubMed]
- Mascalchi M, Belli G, Zappa M, et al. Risk-benefit analysis of X-ray exposure associated with lung cancer screening in the Italung-CT trial. AJR Am J Roentgenol 2006;187:421-9. [PubMed]
- Eisenberg RL, Bankier A, Boiselle P. Compliance with Fleischner Society guidelines for management of small lung nodules: a survey of 834 radiologists. Radiology 2010;255:218-24. [PubMed]
- Wiener RS, Schwartz LM, Woloshin S, et al. Population-based risk for complications after transthoracic needle lung biopsy of a pulmonary nodule: an analysis of discharge records. Ann Intern Med 2011;155:137-44. [PubMed]
- Wagnetz U, Menezes RJ, Boerner S, et al. CT screening for lung cancer: implication of lung biopsy recommendations. AJR Am J Roentgenol 2012;198:351-8. [PubMed]
- Wilson DO, Weissfeld JL, Fuhrman CR, et al. The Pittsburgh Lung Screening Study (PLuSS): outcomes within 3 years of a first computed tomography scan. Am J Respir Crit Care Med 2008;178:956-61. [PubMed]
- Vazquez MF, Koizumi JH, Henschke CI, et al. Reliability of cytologic diagnosis of early lung cancer. Cancer 2007;111:252-8. [PubMed]
- Leong S, Ju H, Marshall H, et al. Electromagnetic navigation bronchoscopy: a descriptive analysis. J Thorac Dis 2012;4:173-85. [PubMed]
- Steinfort DP, Khor YH, Manser RL, et al. Radial probe endobronchial ultrasound for the diagnosis of peripheral lung cancer: systematic review and meta-analysis. Eur Respir J 2011;37:902-10. [PubMed]
- Blasberg JD, Pass HI, Donington JS. Sublobar resection: a movement from the Lung Cancer Study Group. J Thorac Oncol 2010;5:1583-93. [PubMed]
- Nakamura K, Saji H, Nakajima R, et al. A phase III randomized trial of lobectomy versus limited resection for small-sized peripheral non-small cell lung cancer (JCOG0802/WJOG4607L). Jpn J Clin Oncol 2010;40:271-4. [PubMed]
- van den Bergh KA, Essink-Bot ML, Borsboom GJ, et al. Long-term effects of lung cancer computed tomography screening on health-related quality of life: the NELSON trial. Eur Respir J 2011;38:154-61. [PubMed]
- Vierikko T, Kivisto S, Jarvenpaa R, et al. Psychological impact of computed tomography screening for lung cancer and occupational pulmonary disease among asbestos-exposed workers. Eur J Cancer Prev 2009;18:203-6. [PubMed]
- Byrne MM, Weissfeld J, Roberts MS. Anxiety, fear of cancer, and perceived risk of cancer following lung cancer screening. Med Decis Making 2008;28:917-25. [PubMed]
- van den Bergh KA, Essink-Bot ML, Bunge EM, et al. Impact of computed tomography screening for lung cancer on participants in a randomized controlled trial (NELSON trial). Cancer 2008;113:396-404. [PubMed]
- Parsons A, Daley A, Begh R, et al. Influence of smoking cessation after diagnosis of early stage lung cancer on prognosis: systematic review of observational studies with meta-analysis. BMJ 2010;340:b5569. [PubMed]
- Ostroff JS, Buckshee N, Mancuso CA, et al. Smoking cessation following CT screening for early detection of lung cancer. Prev Med 2001;33:613-21. [PubMed]
- Cox LS, Clark MM, Jett JR, et al. Change in smoking status after spiral chest computed tomography scan screening. Cancer 2003;98:2495-501. [PubMed]
- Styn MA, Land SR, Perkins KA, et al. Smoking behavior 1 year after computed tomography screening for lung cancer: effect of physician referral for abnormal CT findings. Cancer Epidemiol Biomarkers Prev 2009;18:3484-9. [PubMed]
- Field JK, Smith RA, Aberle DR, et al. International Association for the Study of Lung Cancer Computed Tomography Screening Workshop 2011 report. J Thorac Oncol 2012;7:10-9. [PubMed]
- Bach PB. Reduced lung-cancer mortality with CT screening. N Engl J Med 2011;365:2036-author reply 2037-8. [PubMed]
- Strong K, Wald N, Miller A, et al. Current concepts in screening for noncommunicable disease: World Health Organization Consultation Group Report on methodology of noncommunicable disease screening. J Med Screen 2005;12:12-9. [PubMed]
- Humphrey LL, Deffebach M, Pappas M, et al. Screening for lung cancer with low-dose computed tomography: a systematic review to update the U.S. preventive services task force recommendation. Ann Intern Med 2013. [Epub ahead of print]. [PubMed]
- Patel D, Akporobaro A, Chinyanganya N, et al. Attitudes to participation in a lung cancer screening trial: a qualitative study. Thorax 2012;67:418-25. [PubMed]
- van der Aalst CM, van Iersel CA, van Klaveren RJ, et al. Generalisability of the results of the Dutch-Belgian randomised controlled lung cancer CT screening trial (NELSON): does self-selection play a role? Lung Cancer 2012;77:51-7. [PubMed]
- Youlden DR, Cramb SM, Baade PD. The international epidemiology of lung cancer: geographical distribution and secular trends. J Thorac Oncol 2008;3:819-31. [PubMed]
- Marshall H, Bowman R, Crossin J, et al. The Queensland Lung Cancer Screening Study: risk stratification using participant data and lung function tests can significantly increase screening effectiveness, Journal of Thoracic Oncology 4th Australian Lung Cancer Conference (ALCC), Adelaide, 2012.
- Tammemagi CM, Pinsky PF, Caporaso NE, et al. Lung cancer risk prediction: Prostate, Lung, Colorectal And Ovarian Cancer Screening Trial models and validation. J Natl Cancer Inst 2011;103:1058-68. [PubMed]
- Tammemägi MC, Katki HA, Hocking WG, et al. Selection criteria for lung-cancer screening. N Engl J Med 2013;368:728-36. [PubMed]
- Cassidy A, Myles JP, van Tongeren M, et al. The LLP risk model: an individual risk prediction model for lung cancer. Br J Cancer 2008;98:270-6. [PubMed]
- Australian Population Health Development Principal Committee Screening Subcommittee, Population based screening framework. Commonwealth of Australia, Canberra, 2008.
- Bianchi F, Nicassio F, Marzi M, et al. A serum circulating miRNA diagnostic test to identify asymptomatic high-risk individuals with early stage lung cancer. EMBO Mol Med 2011;3:495-503. [PubMed]
- Hennessey PT, Sanford T, Choudhary A, et al. Serum microRNA biomarkers for detection of non-small cell lung cancer. PloS One 2012;7:e32307. [PubMed]
- Peled N, Hakim M, Bunn PA Jr, et al. Non-invasive breath analysis of pulmonary nodules. J Thorac Oncol 2012;7:1528-33. [PubMed]