Physicochemical and pharmacokinetic properties of polymeric films loaded with cisplatin for the treatment of malignant pleural mesothelioma
Introduction
Mesothelioma is an aggressive tumor of serosal surfaces, such as the pleura and the peritoneum. The principal cause of this tumor is the professional or environmental exposure to asbestos by inhalation (1). Even if it is a rare disease, concerns about this cancer are constantly growing since a peak in the incidence is expected in the next few years, as a result of the extensive industrial and household use of asbestos in the past decades (2). Furthermore, the life expectancy for mesothelioma-stricken patients is 4–12 months after the diagnosis, if a therapy is not initiated in the early stages of the disease. Current mesothelioma treatment is unsatisfactory and local tumor recurrence is common, even after complete surgical resection combined with chemotherapy and radiotherapy (3,4).
Polymeric films, beads or microparticles have been proposed as anticancer drug delivery systems to be used as local implants to achieve high drug concentrations locally, while limiting severe systemic toxicity (5). In particular, this approach has been applied clinically with the aim of limiting relapses after surgical debulking of glioma and other brain tumors (6). Gliadel® (UK-Guilford Pharmaceuticals) is a carmustine-loaded wafer, composed of polifeprosan 20, a biodegradable polyanhydride copolymer, able to release the drug over three weeks and is an increasingly popular approach for the delivery of chemotherapy to glioma patients (7).
Recently, a local intrapleural approach was proposed for the treatment of malignant pleural mesothelioma (MPM). The local application of chemotherapy and immunotherapy for the treatment of malignant pleural mesothelioma has been tested in a clinically relevant orthotopic tumor model in rats (8,9). Opitz and coworkers (10) were able to demonstrate a better control of tumor relapses when cisplatin, intrapleurally applied, was loaded to a fibrin sealant called Vivostat® (Vivostat A/S, Danemark).
In this paper, cisplatin-loaded chitosan and hyaluronic acid films, conceived for a locoregional treatment of the malignant mesothelioma, are presented.
Cisplatin (cisplatinum or cis-diamminedichloroplatinum) is a metallic platinum coordination compound with anticancer properties, as, once penetrated into cancer cells, it forms adducts with many cellular components; however, DNA is the primary target of the drug. Since its approval in 1978, cisplatin has been employed in several anticancer protocols either alone or in combination with other compounds. In fact, cisplatin is approved for the treatment of malignant mesothelioma and several other malignancies, i.e., ovarian, testicular, head and neck, non-small cell lung and bladder cancer (11).
Platinum compounds have been investigated both as single agents and in combined regimens for MPM. Cisplatin mono-therapy demonstrated an overall response rate of 14% at a dose of 100 mg/m2 given every 21 days in patients with diffuse malignant mesothelioma. At higher doses (e.g., 80 mg/m2 weekly), cisplatin achieved a response rate of 36% with significant discontinuation (34%) due to toxic effects (12,13).
Hyaluronate and chitosan are polysaccharides that have been employed for drug delivery applications because of their remarkable biopharmaceutical properties.
Hyaluronic acid, an animal ubiquitous polysaccharide composed of repeating disaccharide units of D-glucuronic acid and N-acetyl-D-glucosamine, because of its biocompatibility, non-immunogenicity, biodegradability and viscoelasticity has emerged as an ideal biomaterial for cosmetic, medical and pharmaceutical applications (14). In fact, hyaluronate has found clinical application in the treatment of osteoarthritis, in wound healing and in ophthalmological and otological surgery (15), but it has also been proposed as main constituent of various pharmaceutical forms, such as microspheres (16,17), hydrogels (18,19), films (20,21) and implants (22). Moreover, the bioconjugate paclitaxel-hyaluronate (ONCOFID-P®) have been proposed for the intravescical local treatment of superficial bladder cancer (23,24).
Chitosan is a polymer obtained from the deacetylation of chitin, the main component of the crustacean exoskeleton with mucoadhesive properties that can be used to enhance the therapeutic efficacy of the drug either by increasing the contact time at the site of action or by enhancing the permeation through mucosal barriers by “opening” tight junctions (25,26). Its potential role in drug delivery as functional excipient in various dosage forms have been well described (27,28).
These biomaterials have been used to prepare polymeric films loaded with cisplatin, suitable for a local application after surgical resection of the tumor nodules on the pleural surface and able to reduce recurrence. The films prepared have been studied both in vitro and in vivo in a mesothelioma animal model, to evaluate if their favorable mechanical and biopharmaceutical characteristics would allow to propose polysaccharide films as a drug delivery platform for the surgical administration of anticancer drugs for the prevention of tumors recurrence.
Methods
Materials
The anticancer drug, cisplatin, with purity higher than 99.9% and lysozyme (lyophilized powder from chicken egg white, 50,000 UI/mg) were obtained from Sigma-Aldrich (Saint Louis, USA). Chitosans with high (103 cP) and low (8 cP) viscosity were purchased from Primex Primex (ChitoClear® FG, deacetylation degree 95 and 99% respectively, Siglufjordur, Iceland). Sodium hyaluronate (HA Ophthalmic, code 811240) was a kind gift of Fidia Pharmaceuticals (Abano Terme, Italy). Polyvinyl alcohol was obtained from Nippon-Goshei (PVA 83000, Osaka, Japan). Polyethylene glycol 1000 mono stearate (PEG 1000S), Polyethylene glycol 200 (PEG 200), acetic acid and sorbitol were purchased from ACEF (Fiorenzuola d’Arda, Italy). Water for films preparation was ultrapure filtered water (Purelab Flex; ELGA-Veolia LabWater, Windsor Court, UK).
All other chemicals, reagents and solvents were of analytical grade.
Production of the cisplatin-loaded polysaccharide films
Films containing chitosan or hyaluronic acid as main component were produced, with no drug or containing 0.5% or 4% w/w of cisplatin. Beside cisplatin-loaded films (CPt0.5, CPt4, HPt0.5, HPt4), blank films (CB, HB) were produced for comparison. The composition by weight of films is presented in Table 1 for hyaluronate-based films and Table 2 for chitosan-based films.
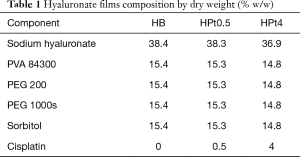
Full table
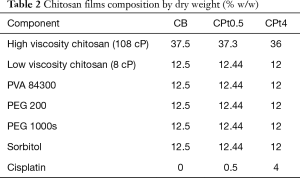
Full table
The polysaccharide and the drug were dissolved in water along with PVA, PEG 1000S, PEG 200 and sorbitol, that were used as film forming and plasticizer agents. For chitosan, the polysaccharide dissolution was obtained by adding 1% v/v acetic acid to the viscous solution. Total components concentration was 6.5 g/100 mL for hyaluronate-based films and 8.0 g/100 mL for chitosan-based films. The polymeric films were produced by laminating the viscous solution obtained (variable opening casting knife BYK Gardner, Geretsried, Germany, gap 2 mm) on a polyester translucent laminate film (Scotchpak 1220 Backing, 3M Italia, Segrate, Italy) and subsequent drying for 8 h in an oven (55 °C).
Films thickness was evaluated using a digital thickness gauge having sensibility of the order of 0.01 mm (Thickness gage Model 547-300S, Mitutoyo, Laiante, Italy).
Residual water content was evaluated for all films by Karl-Fisher water titration (Titromatic S, Crison, Barcelona, Spain) using sodium tartrate tetrahydrate as standard.
Evaluation of the film mechanical properties
The mechanical properties of films were evaluated with an electronic dynamometer (AG MC2, Acquati, Arese, Italy) equipped with a 5 kg load cell. Strips of films (1 cm × 7 cm), held between two clamps positioned at a distance of 5 cm, were subjected to constant traction (0.5 mm/s) and the force applied to the film and elongation were recorded until the film broke.
The tensile strength (Tsb) and the elongation to break (Eb) were calculated as shown in Eqs. [1] and [2] respectively:
where Ts (N/mm2) is the tensile strength, Fb is the breaking force (N) and Cs is the cross-section area of the sample (mm2);
where Eb is the elongation at break expressed as percentage, ∆L is the increase in length at the breaking point (mm) and L0 is the original length of the film (mm).
Hot stage polarized light optical microscopy
The films were observed under an optical microscope with polarized light and a hot stage. A hot stage apparatus (HSF 91, Linkam Scientific Instruments, Tadworth, UK) equipped with a polarising microscope (Optiphot 2-POL, Nikon, Tokyo, Japan) and a digital camera (Coolpix 4500, Nikon, Japan) allowed the recording of images during temperature scans. Samples, placed between two glass cover slides, were heated from ambient temperature up to 250 °C, scan rate 10 K•min–1.
Scanning electron microscopy
To investigate the morphology and elemental composition of the surface of the polymeric films, they were examined with a scanning electron microscope (JSM 6400, Jeol Ltd., Tokyo, Japan), equipped with a detector EDS (Energy Dispersive X-Ray Spectrometry).
Cisplatin release from polysaccharide films
For the release of the anticancer drug from polymeric films, samples with the same shape and surface area (rectangular shaped 1 cm × 7 cm, 7 cm2) were cut, weighed and placed in amber glass containers with 15 mL of saline solution (NaCl 0.9%). In some experiments lysozyme 3 mg/mL was added to the dissolution medium in order to provide a simulated bioerosion. The containers were placed in a water bath shaker at 37 °C (Gyrotory, New Brunswick Scientific, Edison, USA). Samples were taken at regular intervals. The concentration of cisplatin was determined by HPLC.
The concentration of cisplatin in release samples was determined by liquid chromatography according to the method reported in European Pharmacopeia (Ph. Eur. 5.0) monograph for cisplatin. The chromatographic analysis was carried out on a Shimadzu HPLC apparatus (Kyoto, Japan) using a column of 25 cm and 4.6 mm of internal diameter, packed with strong-anion-exchange-silica gel for chromatography (Spherisorb SAX, Waters, Santry, Ireland). As mobile phase flowing at 1.2 mL/min a mixture of NaCl 9 g/L aqueous solution and methanol 10:90 was used. The UV detector was set at 220 nm and injection volume was 20 µL. The linearity of the method was confirmed in the range 5–500 µg/mL (R2=0.9999).
Cell culture studies
Cytotoxicity studies were performed on A549 (human non-small cell lung cancer cell line, NSCLC) in six well plates seeded with 20,000 cells/cm2. Cells were cultured as recommended (29). Films discs CB, HB, CPt0.5, HPt0.5 (1 cm Ø) were laid on cells 24 h after seeding. Cell viability was determined after 8, 24 and 72 h of incubation by imaging after crystal violet staining and by MTT assay, as previously described (30).
In vivo experiments in an orthotopic rat recurrence model of malignant pleural mesothelioma
The study protocol was approved by the local veterinary committee and the experiments were performed according to Guiding Principles in the Care and Use of animals (DL 116/92).
Male Fisher rats 344 weighing 280–300 g (mean 291 g) were purchased from Charles River Laboratories (Calco, Italy). Upon arrival, the animals were placed in the animal facility of the Department of Food and Drug of the University of Parma. They were kept on a schedule of 12 hours of light/12 hours of darkness; food and water were supplied ad libitum.
The mesothelioma cell line IL-45, derived from asbestos-induced peritoneal mesothelioma in rats, was used to develop the tumor in animals (10). Cells were kindly donated by the laboratory of the University Hospital of Zurich (CH-Zurich). The tumor cells were cultured in RPMI medium supplemented with glutamine 2 mM, 10% fetal calf serum, 100 U/mL penicillin and 100 mg/mL streptomycin, and placed in a 37 °C, 5% CO2 incubator. Before the experiment, 1 million cells were suspended in 50 µL of complete growth medium and the tubes were put on ice.
Thirty-five rats were randomly assigned to the different treatment groups: (I) control (no adjuvant therapy after the surgical procedure); (II) blank chitosan films (CB); (III) blank hyaluronate films (HB); (IV) cisplatin solution (1.5 mL NaCl 0.9% containing cisplatin at a concentration of 0.6 mg/mL); (V) cisplatin-loaded chitosan films (CPt0.5); (VI) cisplatin-loaded hyaluronate films (HPt0.5). Formulations were administered in such an amount to have a dose of 3 mg/kg per rat.
The surgical procedures have already been reported in detail (31). Briefly, a tumor nodule of 5.5 mean diameter (range, 4.4–6.1 mm) always developed six days after subpleural mesothelioma cell inoculation. After pleural tumor resection and pneumonectomy were carried out, animals received the adjuvant treatment according to randomization. In the case of polysaccharide-based films, they were accurately placed into the cavity in order to cover all the pleural surfaces. The application of the device over all the chest cavities was facilitated by a small cotton tip. In all the cases, the film was well adherent to the parietal pleura at the end of the procedure.
Cisplatin determination in the serum was performed at prefixed time points by ICP-MS with the method described in the following section. Blood samples were collected at predetermined time points (24, 48 and 144 hours post-treatment) through a puncture of one of the veins of the tongue of rats anesthetized with ether. Blood samples were centrifuged for 10 min at 1,000 g and serum was t stored at −80 °C until analysis.
Six days after the treatment (surgical resection and intrapleural therapy), all the animals were humanely euthanized and subsequently necropsy was performed. The length, width and thickness of the tumor nodule were measured and the volume of the tumor recurrence evaluated using the formula: V= 4/3π abc, being a, b and c semi-axes of the tumor.
Platinum quantification in biological samples
Quantification of cisplatin in biological samples was performed by detection of elemental platinum using the ICP-MS X Series II (ThermoFisher Corporation, USA-Waltham) equipped with an AS-500 autosampler (CETAC, USA-Omaha). The operating parameters of the ICP-MS instrument were as follows: RF power 1,400 W; coolant gas flow 15.5 L•min−1; auxiliary gas flow 0.98 L•min−1; nebulizer gas flow 0.87 L•min−1; nickel standard Xi cones; peak jumping data acquisition mode: dwell time 100 ms; duration time 60 s; standard resolution. The instrument optimization was performed daily with the auto-tune procedure to assure a response of at least 80,000 cps/µg•L−1 for indium and 100,000 cps/µg•L−1 for uranium in the high mass range. All the platinum isotopes were acquired to account for spectroscopic interferences, but only 195Pt was used for calculations. The cisplatin concentrations were calculated starting from amount of platinum found in each sample. All the analyzed samples were previously treated with aqua regia in a microwave assisted MLS-1200 MEGA apparatus (Milestone, Sorisole, Italy) equipped with an MDR-1000-6 rotor and diluted with ultrapure water to a final volume of 10 mL.
Statistics
The statistical test for evaluating data was performed with the software Kaleidagraph (ver. 4.1.1, Synergy Software, Reading, PA, USA). Data are provided as mean and standard deviation. Student t-test or the ANOVA test applying Bonferroni post-hoc test were applied to compare data groups. Bonferroni correction was applied for comparison between all groups. Values of P<0.05 were considered to be significant.
Results
Cisplatin-loaded polysaccharide film characterization
Polysaccharide film produced was thin with a smooth surface. SEM images of the film surfaces are presented in Figure 1. The microscopic image showed a flat, relatively regular surface for all the samples, except in the case of the high cisplatin loading in hyaluronate film where some irregularly shaped stains were noticed. Energy dispersive X-ray analysis confirmed the presence of platinum (data not shown) in the films loaded with cisplatin.
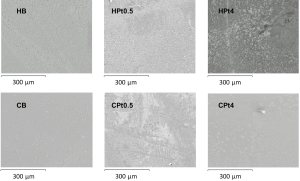
Thickness, weight and residual water content of polysaccharide films are presented in Table 3. Films where thin, being thickness below 100 µm, had a weight per surface area approaching 10 mg/cm2 and retained a fair amount of water, higher for blank films and significantly reduced in the case of cisplatin-loaded films for both polysaccharides (CB vs. CPt05 or CPt4, t-test P<0.05; HB vs. HPt05 or HPt4, t-test P<0.001).

Full table
Films were resistant and still sufficiently elastic to allow their bending and cutting for further uses. These mechanical properties were considered pivotal for the manipulation and adaptation to the pleural surface during surgery. Table 4 summarizes the mechanical properties of the polysaccharide films produced.

Full table
The result showed that chitosan films are more rigid, with higher tensile strength and lower elongation, while hyaluronate films appear to be more plastic, with higher elongation at brake. Cisplatin inclusion does not appear to affect the properties of chitosan based films, while an increase in the tensile strength of hyaluronate films appears evident at least for the films with the lower loading of the anticancer drug.
When dissolution studies were performed they were conducted initially on the films with the higher cisplatin loading. The results obtained are presented in Figure 2. For the higher loading of cisplatin 4% w/w, a burst release was observed for the hyaluronate films, with more than 90% of the dose released in just three hours. On the contrary, chitosan films showed a slow drug release with just below 25% of the dose released in 8 days.
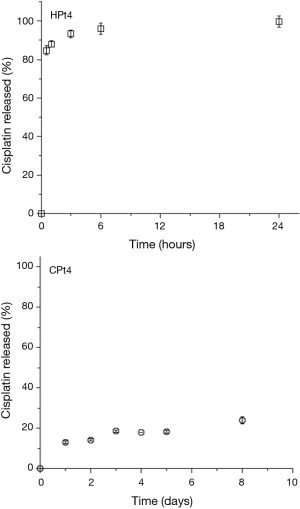
The rapid release of cisplatin from the hyaluronate films was attributed to the high loading of cisplatin, which was hypothesized to lead to a precipitation of the drug in the polysaccharide matrix, leading to a rapid dissolution of the crystals and a poor interaction with the hyaluronate. This hypothesis was confirmed by the observation of the films under polarized light microscopy (Figure 3). At ambient temperature, it appears clear the presence of bright crystals inside the film, upon heating the crystals start to show loss of the crystalline structure around 200 °C. In fact, cisplatin is not melting but decomposes at temperature around 270 °C (32).
Considering that the aim of the drug formulation was to provide a controlled release of the cisplatin at the site of the primary tumor after surgical removal, it was decided to reduce the loading of the films in order to have a better control of the release rate. The results of the release obtained with polysaccharide films loaded with 0.5% w/w cisplatin are presented in Figure 4.
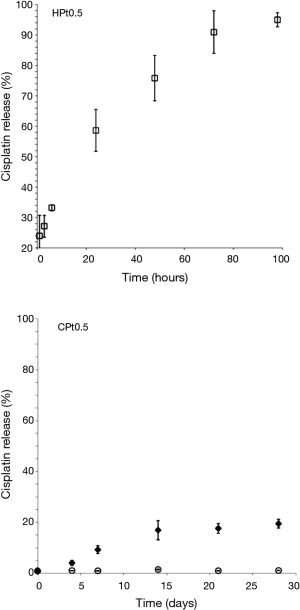
The release for HPt0.5 was more controlled with an almost complete release of the drug obtained in 96 hours. On the contrary CPt0.5 did not show signs of release of cisplatin. Cisplatin was released only in presence of a physiologic enzyme able to degrade the chitosan matrix such as lysozyme making this film a promising approach to obtain a drug release through progressive bio-erosion.
Considering the results obtained, it was decided to study in further experiments only the polysaccharide films containing the lower amount of cisplatin, since the drug delivery platform offered a better control of the release of the platinum compound.
Cell culture studies on A549 human NSCLC cell line
The efficacy of the cisplatin present in the films was assessed by means of in vitro studies on A549 NSCLC cell line. As shown in Figure 5, only the cisplatin-loaded hyaluronate films (HPt0.5) were able to significantly inhibit the growth of the cells. Cisplatin-loaded chitosan films did not show any toxicity possibly as a consequence of the extremely low release rate and of the necessity of bio-erosion to allow the delivery of the drug. The films not containing cisplatin (CB and HB) did not show any significant reduction in cell viability.
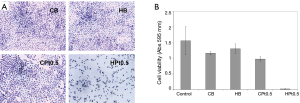
The time dependent toxicity linked to the release of cisplatin from the hyaluronate films was elegantly demonstrated with cytotoxicity assays carried out after 8 and 24 hours of incubation on A549 cells.
In Figure 6, it is shown that after 8 hours no apparent growth inhibition is evident, while after 24 hours cisplatin-loaded hyaluronate films caused a reduction of cell viability, while cells untreated or treated with hyaluronate films without the anticancer drug kept growing.
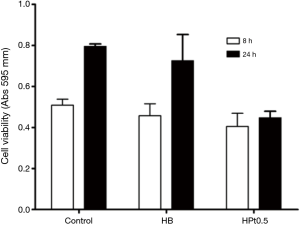
In vivo studies in an orthotopic animal model of mesothelioma recurrence
In vivo studies on the films in an orthotopic rat model of mesothelioma recurrence have been reported previously (31). Here some considerations relative to the efficacy and pharmacokinetics data obtained in relation to the physicochemical and biopharmaceutical properties of the film are presented.
A relevant observation came from the immediate examination of the thoracic wall of the animals at the end of the experiment (Figure 7). In the case of chitosan-based films, not only the film was found almost intact but a significant reaction towards this delivery system was evidenced with the formation of a fibrotic capsule around the film. In addition, the tumor actually recurred in animals treated with the cisplatin-loaded chitosan films (CPt0.5, average volume 2010 mm3).
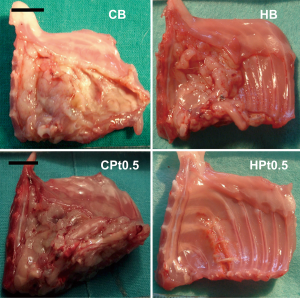
Films devoid of the anticancer drug did not prevent mesothelioma recurrence, with an average tumor volume of 1,376 and 970 mm3 for HB and CB, respectively. On the contrary, cisplatin-loaded hyaluronate films completely dissolved during the 6 days of experiment, leaving a thoracic wall completely healed of the surgical wound and almost completely devoid of the tumor (average volume 11 mm3). These results were in part explained by the pharmacokinetics of cisplatin after the intrapleural administration of the polysaccharide films (Table 5) . In fact, in parallel to what evidenced in vitro, chitosan films released minimal quantities of cisplatin during the 6 days of the experiment, leading to plasma concentrations in the range of 10–20 ng/mL and an AUC around 10% of that obtained by the intrapleural administration of a cisplatin solution. On the contrary, the release of cisplatin from hyaluronate films was progressive but sufficiently rapid to provide elevated plasma concentration lasting up to 6 days and higher than those obtained with the cisplatin solution and with an AUC almost seven times higher. Interestingly, despite the elevated and sustained cisplatin plasma concentrations for hyaluronate films, no significant toxicity related to the treatment was evidenced.
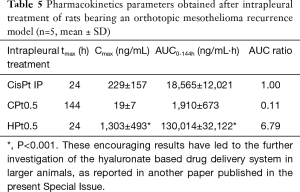
Full table
Discussion
Symptoms of pleural mesothelioma occur as a result of the important thickening of the pleural membrane and the production of fluid between membrane layers. Tissue thickening and pleural fluid production caused lungs atelectasis, leading to worsening reduced respiratory function. As a consequence of symptoms so unspecific, an early diagnosis is so rare that mesothelioma treatment is usually unable to provide a complete cure (33). MPM requires multiple therapeutic modalities, including surgery, chemotherapy, and radiation therapy, but the optimal combination of therapies remains debated (34). Generally, surgery is recommended for patients with clinical stage I disease who are considered medically fit and can tolerate the intervention. Patients with stage II-III disease should be offered trimodality therapy with surgery, chemotherapy and radiotherapy. Chemotherapy alone is recommended for patients who are not medically fit for surgery, have stage IV disease, and/or show sarcomatoid histology (35).
Extrapleural pneumonectomy (EPP) and pleurectomy/decortication (P/D) have been proposed as surgery intervention, but in most cases, do not offer a complete disease cure. As the aggressive local tumor infiltration into the chest wall and other surrounding tissue is a typical characteristic of MPM, local recurrence is a frequent problem in MPM patients after EPP (33%), but even higher after P/D (65%). The prevention or delay of local relapse after surgical removal of the tumor is the major concern related to the surgical treatment of pleural mesothelioma (36).
Cisplatin is used alone or in combination in MPM chemotherapy, but its systemic administration is often limited by severe toxic side effects, as well as the intrinsic and acquired resistance manifested by various cancers. Typical cisplatin side effects include nephrotoxicity, hematological toxicity and neurotoxicity. The necessity to improve the chemotherapy efficacy and especially, its ability to reduce local recurrence is evident from recent studies (37). The drug delivery system proposed here i.e., an implant film for intrapleural application composed of a polysaccharide matrix loaded with cisplatin to be used after surgery for tumor resection, is a drug delivery system addressing this need.
In the case of the MPM, the local intrapleural administration of the anticancer drug combines two advantages: the increased local exposure of the pleura and the slow drug elimination from the pleural cavity due to a progressive absorption. It is then clear that innovation in the field of loco-regional drug administration is a viable strategy to increase the effectiveness of chemotherapy and to reduce toxic side effects for lung cancer patients undergoing chemotherapy (38). In the case of the two materials studied in this research, chitosan, despite being a material used often for implants and for tissue engineering (39) and considered biocompatible and relatively non pro-inflammatory (40), showed a strong foreign body reaction and released cisplatin at an extremely low rate in vitro as well as in vivo. However, recently it has been reported that chitosan can show mild to moderate chronic inflammation (41) and foreign body reaction (42) in the case of crosslinked chitosan derivatives. Actually, considering also the strong affinity of cisplatin evidenced for chitosan, it is possible that cisplatin is acting as a crosslinker of the polysaccharide in this case. The mechanism of action of cisplatin is related to the formation inside cells of a potent electrophilic form that can react with any nucleophilic group, including the sulfhydryl groups on proteins and nitrogen donor atoms on nucleic acids (43). Chitosan, with the available amino groups of its glucosamine monomers, is a perfect surrogate of the biomolecules target of cisplatin reactivity. Interestingly, the cisplatin has been found to interact also with hyaluronic acid, which presents carboxylic and acetylated amino groups, forming conjugates and even nanoparticles (44-46).
The benefit of the local therapy, in particular with hyaluronate-based films, is the ability to maintain high chemotherapy concentration at the tumor site for a long time, but at the same time to avoid systemic toxic effects. Hyaluronic acid, a physiological component of the extracellular matrix, is a biocompatible polymer that appears ideal for this application. The film, thin and flexible, was designed for a precise and gentle application on the pleural surface after surgery, with the idea of covering the entire pleural area exposed during surgery. The film is stuck on the wet pleura area by gentle pressure and by hydration forms a gel able to release cisplatin for at least four days in vitro, providing high cisplatin plasma concentrations for 6 days in vivo, without any apparent toxicity.
This latter point is a significant point of differentiation of the cisplatin-loaded hyaluronate-based films with the approach using the fibrin sealant Vivostat® proposed for the delivery of cisplatin intrapleurally. In fact, this latter approach has been reported to lead to very low blood levels of drug, indicating, similarly to what evidenced her for chitosan, a high interaction between the carrier and the anticancer drug (47). The hyaluronate film instead, is dissolving completely providing high cisplatin concentrations locally but allowing the complete release of the drug, its distribution and eventual anticancer action on secondary metastases and its disposal by renal elimination.
Conclusions
Chitosan and hyaluronate-based films loaded with cisplatin have been produced in view of the locoregional treatment of malignant pleural mesothelioma. The films showed mechanical resistance and flexibility suitable for the application after surgery aiming at the removal of the disease from the pleural surface. The release profiles obtained from the films were strongly dependent on the polysaccharide used. Hydrophilic water soluble hyaluronate films showed a cisplatin release dependent on drug loading, with a complete release within 6 hours for high cisplatin content (4% w/w) and a release protracted up to 4 days for low cisplatin content (0.5% w/w). Chitosan films controlled the release cisplatin over a long period of time and even required the action of specific enzymes to allow for cisplatin release, possibly because of a crosslinking action of cisplatin on the polysaccharide.
Animal studies in a model of mesothelioma showed that only cisplatin loaded hyaluronate-based films were able to almost completely prevent tumor recurrence, while chitosan films induced inflammation and foreign body reaction. Hyaluronate films on the contrary, provided higher local and plasmatic concentrations of cisplatin in comparison to the administration of an equivalent intrapleural dose of cisplatin solution, but without any sign of associated toxicity. These results indicate that the hyaluronate films are an ideal platform for the development of drug delivery systems providing biocompatibility, improved efficacy and reduced toxicity suitable for the loco-regional post-surgical treatment of lung malignancies with cisplatin.
Acknowledgements
This work was supported by CHIESI Farmaceutici S.p.A. (Parma); Transfer Oil S.p.A. (Colorno, PR); Famiglia Gigetto Furlotti (Parma); Associazione Volontaria Promozione Ricerca Tumori (A.VO.PRO.RI.T.) (Parma), Associazione Augusto per la Vita (Novellara, Reggio Emilia).
Footnote
Conflicts of Interest: P Colombo is the founder and CEO of the start-up Plumestars s.r.l. F Sonvico has received support for the development of polysaccharide films from Plumestars s.r.l. The other authors have no conflicts of interest to declare.
Ethical Statement: The study protocols were approved by the Local Veterinary Committee (Prot. N° 39/09, Prot. N° 23/10 and Prot. N° 92/11) and the experiments were conducted in accordance with the Guiding Principles in the Care and Use of animals (Legislative Decree 116/92) and Regional Decree 20/2002 of Emilia Romagna Region, Italy.
References
- Robinson BW, Lake RA. Advances in malignant mesothelioma. N Engl J Med 2005;353:1591-603. [Crossref] [PubMed]
- Peto J, Decarli A, La Vecchia C, et al. The European mesothelioma epidemic. Br J Cancer 1999;79:666-72. [Crossref] [PubMed]
- Sterman DH, Albelda SM. Advances in the diagnosis, evaluation, and management of malignant pleural mesothelioma. Respirology 2005;10:266-83. [Crossref] [PubMed]
- Taub RN, Keohan ML, Chabot JC, F, et al. Peritoneal mesothelioma. Curr Treat Options Oncol 2000;1:303-12. [Crossref] [PubMed]
- Saltzman WM, Fung LK. Polymeric implants for cancer chemotherapy. Adv Drug Deliv Rev 1997;26:209-30. [Crossref] [PubMed]
- Kumar Naraharisetti P, Yung Sheng Ong B, Wei Xie J, et al. In vivo performance of implantable biodegradable preparations delivering Paclitaxel and Etanidazole for the treatment of glioma. Biomaterials 2007;28:886-94. [Crossref] [PubMed]
- Attenello FJ, Mukherjee D, Datoo G, et al. Use of Gliadel (BCNU) wafer in the surgical treatment of malignant glioma: a 10-year institutional experience. Ann Surg Oncol 2008;15:2887-93. [Crossref] [PubMed]
- Lardinois D, Jung FJ, Opitz I, et al. Intrapleural topical application of cisplatin with the surgical carrier Vivostat increases the local drug concentration in an immune-competent rat model with malignant pleuromesothelioma. J Thorac Cardiov Surg 2006;131:697-703. [Crossref] [PubMed]
- Ampollini L, Soltermann A, Felley-Bosco E, et al. Immuno-chemotherapy reduces recurrence of malignant pleural mesothelioma: an experimental setting. Eur J Cardiothorac Surg 2009;35:457-62. [Crossref] [PubMed]
- Opitz I, Lardinois D, Arni S, et al. Local recurrence model of malignant pleural mesothelioma for investigation of intrapleural treatment. Eur J Cardiothorac Surg 2007;31:773-8. [Crossref] [PubMed]
- Macciò A, Madeddu C. Cisplatin: an old drug with a newfound efficacy - from mechanisms of action to cytotoxicity. Expert Opin Pharmacother 2013;14:1839-57. [Crossref] [PubMed]
- Steele JP, Klabatsa A. Chemotherapy options and new advances in malignant pleural mesothelioma. Ann Oncol 2005;16:345-51. [Crossref] [PubMed]
- Sørensen JB. Current concepts in chemotherapy for malignant pleural mesothelioma. Clin Respir J 2008;2:74-9. [Crossref] [PubMed]
- Liao YH, Jones SA, Forbes B, et al. Hyaluronan: pharmaceutical characterization and drug delivery. Drug Deliv 2005;12:327-42. [Crossref] [PubMed]
- Kogan G, Šoltés L, Stern R, et al. Hyaluronic acid: a natural biopolymer with a broad range of biomedical and industrial applications. Biotechnol Lett 2007;29:17-25. [Crossref] [PubMed]
- Esposito E, Menegatti E, Cortesi R. Hyaluronan-based microspheres as tools for drug delivery: a comparative study. Int J Pharm 2005;288:35-49. [Crossref] [PubMed]
- Lim ST, Forbes B, Berry DJ, et al. In vivo evaluation of novel hyaluronan/chitosan microparticulate delivery systems for the nasal delivery of gentamicin in rabbits. Int J Pharm 2002;231:73-82. [Crossref] [PubMed]
- Hahn SK, Oh EJ, Miyamoto H, et al. Sustained release formulation of erythropoietin using hyaluronic acid hydrogels crosslinked by Michael addition. Int J Pharm 2006;322:44-51. [Crossref] [PubMed]
- Mayol L, Quaglia F, Borzacchiello A, et al. A novel poloxamers/hyaluronic acid in situ forming hydrogel for drug delivery: rheological, mucoadhesive and in vitro release properties. Eur J Pharm Biopharm 2008;70:199-206. [Crossref] [PubMed]
- Pereira GG, Guterres SS, Balducci AG, et al. Polymeric Films Loaded with Vitamin E and Aloe vera for Topical Application in the Treatment of Burn Wounds. Biomed Res Int 2014;2014:641590.
- Pereira GG, Detoni CB, Balducci AG, et al. Hyaluronate nanoparticles included in polymer films for the prolonged release of vitamin E for the management of skin wounds. Eur J Pharm Sci 2016;83:203-11. [Crossref] [PubMed]
- Yadav AK, Mishra P, Agrawal GP. An insight on hyaluronic acid in drug targeting and drug delivery. J Drug Target 2008;16:91-107. [Crossref] [PubMed]
- Rosato A, Banzato A, De Luca G, et al. HYTAD1-p20: a new paclitaxel-hyaluronic acid hydrosoluble bioconjugate for treatment of superficial bladder cancer. Urol Oncol 2006;24:207-15. [Crossref] [PubMed]
- Banzato A, Bobisse S, Rondina M, et al. A paclitaxel-hyaluronan bioconjugate targeting ovarian cancer affords a potent in vivo therapeutic activity. Clin Cancer Res 2008;14:3598-606. [Crossref] [PubMed]
- Smith J, Wood E, Dornish M. Effect of Chitosan on Epithelial Cell Tight Junctions. Pharm Res 2004;21:43-9. [Crossref] [PubMed]
- Singla AK, Chawla M. Chitosan: Some pharmaceutical and biological aspects--an update. J Pharm Pharmacol 2001;53:1047-67. [Crossref] [PubMed]
- Bernkop-Schnürch A, Dünnhaupt S. Chitosan-based drug delivery systems. Eur J Pharm Biopharm 2012;81:463-9. [Crossref] [PubMed]
- Illum L. Chitosan and its use as a pharmaceutical excipient. Pharm Res 1998;15:1326-31. [Crossref] [PubMed]
- Cavazzoni A, Alfieri RR, Carmi C, et al. Dual mechanisms of action of the 5-benzylidene-hydantoin UPR1024 on lung cancer cell lines. Mol Cancer Ther 2008;7:361-70. [Crossref] [PubMed]
- Alfieri RR, Galetti M, Tramonti S, et al. Metabolism of the EGFR tyrosin kinase inhibitor gefitinib by cytochrome P450 1A1 enzyme in EGFR-wild type non small cell lung cancer cell lines. Mol Cancer 2011;10:143. [Crossref] [PubMed]
- Ampollini L, Sonvico F, Barocelli E, et al. Intrapleural polymeric films containing cisplatin for malignant pleural mesothelioma in a rat tumour model: a preliminary study. Eur J Cardiothorac Surg 2010;37:557-65. [Crossref] [PubMed]
- Dasari S, Tchounwou PB. Cisplatin in cancer therapy: molecular mechanisms of action. Eur J Pharmacol 2014;740:364-78. [Crossref] [PubMed]
- Zauderer MG, Krug LM. The evolution of multimodality therapy for malignant pleural mesothelioma. Curr Treat Options Oncol 2011;12:163-72. [Crossref] [PubMed]
- Opitz I. Management of malignant pleural mesothelioma-The European experience. J Thorac Dis 2014;6 Suppl 2:S238-52. [PubMed]
- Mott FE. Mesothelioma: a review. Ochsner J 2012;12:70-9. [PubMed]
- Stahel RA, Weder W, Lievens Y, et al. Malignant pleural mesothelioma: ESMO Clinical Practice Guidelines for diagnosis, treatment and follow-up. Ann Oncol 2010;21 Suppl 5:v126-8. [Crossref] [PubMed]
- De Souza R, Zahedi P, Allen CJ, et al. Polymeric drug delivery systems for localized cancer chemotherapy. Drug Deliv 2010;17:365-75. [Crossref] [PubMed]
- Garrastazu Pereira G, Lawson AJ, Buttini F, et al. Loco-regional administration of nanomedicines for the treatment of lung cancer. Drug Deliv 2016;23:2881-96. [Crossref] [PubMed]
- Parisi L, Galli C, Bianchera A, et al. Anti-fibronectin aptamers improve the colonization of chitosan films modified with D-(+) Raffinose by murine osteoblastic cells. J Mater Sci: Mater Med 2017;28:136. [Crossref] [PubMed]
- Lu L, Xing C, Xin S, et al. Alkyl chitosan film-high strength, functional biomaterials. J Biomed Mater Res A 2017;105:3034-41. [Crossref] [PubMed]
- Moura MJ, Brochado J, Gil MH, et al. In situ forming chitosan hydrogels: Preliminary evaluation of the in vivo inflammatory response. Mater Sci Eng C Mater Biol Appl 2017;75:279-85. [Crossref] [PubMed]
- Hong Y, Mao Z, Wang H, et al. Covalently crosslinked chitosan hydrogel formed at neutral pH and body temperature. J Biomed Mater Res A 2006;79:913-22. [Crossref] [PubMed]
- Desai N. Challenges in development of nanoparticle-based therapeutics. AAPS J 2012;14:282-95. [Crossref] [PubMed]
- Maeda M, Takasuka N, Suga T, et al. Antitumor activity of a new series of platinum complexes: trans(+/-)-1,2-cyclohexanediammineplatinum(II) conjugated to acid polysaccharides. Anticancer Drugs 1993;4:167-71. [Crossref] [PubMed]
- Cai S, Xie Y, Bagby TR, et al. Intralymphatic Chemotherapy Using a Hyaluronan-Cisplatin Conjugate. J Surg Res 2008;147:247-52. [Crossref] [PubMed]
- Ishiguro S, Cai S, Uppalapati D, et al. Intratracheal Administration of Hyaluronan-Cisplatin Conjugate Nanoparticles Significantly Attenuates Lung Cancer Growth in Mice. Pharm Res 2016;33:2517-29. [Crossref] [PubMed]
- Opitz I, Erne BV, Demirbas S, et al. Optimized intrapleural cisplatin chemotherapy with a fibrin carrier after extrapleural pneumonectomy: a preclinical study. J Thorac Cardiov Surg 2011;141:65-71. [Crossref] [PubMed]