Robotically assisted minimally invasive mitral valve surgery
Introduction
Increased recognition of advantages, over the last decade, of minimizing surgical trauma by operating through smaller incisions and its direct impact on reduced postoperative pain, quicker recovery, improved cosmesis and earlier return to work (1) has spurred the minimally invasive cardiac surgical revolution. This transition began in the early 1990s with advancements in endoscopic instruments, video & fiberoptic technology and improvements in perfusion systems for establishing cardiopulmonary bypass (CPB) via peripheral cannulation (2-8). Society of Thoracic Surgeons data documents that 20% of all mitral valve surgeries are performed using minimally invasive techniques, with half being robotically assisted (9).
Endoscopic instrumentation, with its four degrees of freedom, restricts the dexterity required for safe execution of many cardiac surgical procedures and lack of depth perception with two dimensional video monitors adds a further dimension of complexity to the operation. Robotically assisted minimally invasive cardiac surgery solves both these problems and has allowed a paradigm shift in delivery of healthcare with greatly enhanced patient satisfaction. Robotic systems consist of telemanipulators where micro-instruments, are controlled remotely from a console. The da Vinci SI system (Intuitive Surgical, Mountain View, CA, USA) is the most widely used and is comprised of a surgeon console, an instrument cart and a video platform. The operative console provides the surgeon with a high-definition three-dimensional (3-D) magnified view of the operative field. Finger and wrist movements are registered through sensors and translated into motion-scaled tremor-free movements avoiding the fulcrum effect and instrument shaft shear forces common to long-shafted endoscopic instruments. Wrist-like articulations at the ends of micro-instruments bring the pivoting action of the instrument to the plane of the operative field improving dexterity in tight spaces and allowing truly ambidextrous suture placement.
The mitral valve lies in an annular plane that nearly approximates the sagittal plane of the body and hence the small right anterolateral chest incision for minimally invasive approaches is particularly well suited for its exposure. As the incision moves more laterally in robotically assisted procedures, this angle becomes even more direct. The long robotic instruments allow the surgeons to easily overcome the limitations imposed by increased distance between the operating port and the mitral valve. The small size of access port incision, measuring 1.5-2 cm leads to improved cosmesis and patient satisfaction.
During robotically assisted procedures the surgeon sits on a separate console and manipulates mechanical wrists that allow seven degrees of multidirectional motion. The bedside team, comprising of assistant and nurses, help in exchange of instruments and handing sutures/retrieval of needles. The robotic system will transmit the scaled movements of the surgeon to the robotic arms and the computer corrects for surgical tremors. Figure 1 shows typical setup for a robotically assisted mitral valve repair. Enhanced 3-D visualization of the valve from the console is provided by two parallel camera arms, controlled by the surgeon. Moreover, the use of a dynamic atrial retractor (10) with the robotic platform allows for rapid and continuous retractor repositioning to maximize valve exposure providing an additional advantage over traditional endoscopic approaches. The lack of tactile feedback with robotic procedures is still a limitation, leaving the surgeon to rely on visual cues to determine tissue strain. Potential difficulties with robotic knot-tying is a negative aspect associated with robotic surgery however advent of the Cor-KnotTM and prelooped Leyla sutures (11) as employed by our group have significantly simplified this problem and reduced operative times. The Cor-KnotTM device (LSI Solutions, Inc., Victor, NY) uses a titanium clip to secure sutures, eliminating intra-cardiac knot tying and reducing operative times. Between May 2000 and December 2012, 800 patients underwent robotically assisted mitral valve surgery at our institution. The mean age was 59.7±12.1 years. The mean Cor-KnotTM device time for annuloplasty deployment was significantly shorter when compared with robotic knot tying. Furthermore annuloplasty band placement time, CPB and cross-clamp times also were significantly shorter in the Cor-KnotTM cohort and we concluded that compared to intra-cardiac robotic suture tying, the Cor-KnotTM device shortens all operative times significantly. The mean annuloplasty band placement time was reduced by 10 minutes.

The first robotic-assisted mitral valve surgery was performed in France by Carpentier and colleagues (12) in 1997 using a prototype of the current da Vinci robotic system (Intuitive Surgical, Sunnyvale, CA). Dr Chitwood led the team from East Carolina University in 2000 with the first robotic mitral valve case in the United States (13). In 2002 the Food and Drug Administration provided approval of the da Vinci system for use in cardiac surgery following the pivotal multicenter trial led by Drs. Nifong and Chitwood from the East Carolina University (14).
As the surgical teams worldwide have continued to learn and enhance their experience with this technology, subsequent publications have consistently shown improved recovery times and less pain, associated with robotically assisted procedures (15-18). In a series by Murphy and colleagues (19), more than 60% of robotically assisted mitral valve surgery patients were discharged within 4 days of surgery. In the most recently published meta-analysis from Cheng and colleagues (20), a statistically significant reduction in bleeding, transfusion, incidence of atrial fibrillation, and time to resumption of normal activities was shown when comparing minimally invasive mitral valve surgery with conventional mitral surgery.
An added measure of confidence in this technology comes from the fact that all the above benefits accrue without compromising the quality of outcomes or the durability of mitral valve repair. In the available meta-analyses, perioperative and long-term mortality were equivalent between techniques for mitral valve surgery, as was long-term freedom from reoperations (15,20,21). These findings are supported by two large single-center outcomes studies. Eight-year freedom from reoperation was better as was short-term and 1-year survival (22,23). The largest single-center report of robotically assisted mitral valve surgery comes from our group at the East Carolina Heart Institute (24) which showed a low short and long term mortality of 0.4% and 1.7% respectively in 540 consecutive patients with a 2.9% reoperation rate. These results compared favorably with the meta-analysis published by Cheng et al. (20), in which 30-day mortality was 1.2% and reoperation rate was 2.3%.
However, a significant learning curve exists with the use of robotically assisted mitral valve surgery (25). Both Cheng and colleagues (26) and our group (27) showed a decrease in mitral valve repair failure rate only after 74 and 100 robotic-assisted cases respectively. Operative times, including the cardiopulmonary and aortic cross-clamp times have also been shown to be longer with robotically assisted minimally invasive mitral valve procedures compared with sternotomy approach (20).
One should be cognizant of the fact that most series of minimally invasive procedures including mitral valve surgery are carefully selected and when comparing outcomes (non-randomized) between robotically assisted mitral valve surgery and conventional surgery, acknowledgement of selection bias will be critical. For example, patients with significant peripheral vascular disease are more likely to be excluded from consideration for robotically assisted minimally invasive mitral valve surgery. In the meta-analysis of Cheng and colleagues (20), patients were younger and had a lower incidence of baseline renal failure and pulmonary disease.
Technical considerations
Majority of preoperative evaluation of patients for robotic mitral valve procedures follows along the lines of patients undergoing standard mitral valve surgery with a few notable exceptions. The need for peripheral cannulation for establishing CPB leads to the most important difference. There is increasing support for the role of computed tomography angiography (CTA) of the vascular tree in preoperative assessment of patients being considered for peripheral cannulation. In a study of 141 patients, scheduled for minimally invasive cardiac surgery including robotic procedures at the Cleveland Clinic, 20% patients had their surgical approach changed to sternotomy because of CTA findings related to significant aorto-illiac atherosclerosis and mitral annular calcification (28). Previous right chest surgery or adhesions and presence of breast implants are additional issues which might preclude a robotically assisted procedure. At East Carolina University, we obtain preoperative pulmonary function (PFT) testing in patients with history of COPD and will follow it with right heart catheterization, if needed, because combination of impaired PFT, pulmonary hypertension and impaired right heart function increases the chances of postoperative right heart dysfunction and unilateral pulmonary edema. Lung function tests will also tell us about the patient’s ability to tolerate single lung ventilation.
Patient positioning is typically supine with the right upper trunk elevated (Figure 2). The right arm is positioned at the side to allow the shoulder to drop down to improve surgical exposure. The surgical team should appropriately support the right shoulder to avoid stress on the brachial plexus and the neck. External defibrillator pads should be applied correctly and connected before starting the procedure. The right lung can be reexpanded to attempt enhancement of defibrillation efficacy. The robotic arms should be withdrawn prior to defibrillation to prevent iatrogenic injury.
Robotic mitral valve surgery utilizes right anterolateral thoracotomy approach and hence lung isolation has been a mainstay of anesthetic management. The right lung is typically deflated as the surgeon enters the chest cavity and opens the pericardium to expose the left atrium before the initiation of CPB. Single lung ventilation is also typically used at certain points after separation from bypass: as surgical sites are checked, bleeding is controlled, and the chest closed.
The most common techniques for achieving lung isolation are with a left-sided double-lumen tube (DLT) or a right-sided bronchial blocker placed through a standard single-lumen endotracheal tube. Abnormalities (FEV1 <40% predicted, DLCO <40% predicted) in preoperative lung function tests should alert the surgeon regarding potential problems with single lung ventilation during surgery and the patient should have been counseled appropriately.
The importance of transesophageal echocardiography (TEE) to help guide clinical decision making in cardiac surgery has been well established particularly in the setting of mitral valve repair (29-31). TEE is intricately involved in guiding surgical cannulation, monitoring hemodynamics, and assessing the mitral valve before and after intervention in robotically aided mitral valve surgeries and is an essential tool for the team planning to embark on such operations (32). Planning the MV repair with quantitative mitral valve modeling using 3D echo has been a very useful adjunct (33). TEE will also alert us of presence of aortic regurgitation and left ventricular hypertrophy, both of which warrant adjustment in myocardial preservation strategy. Figure 3 illustrates the anesthetic preparation and monitoring, typical for such cases including double lumen endotracheal tube, TEE probe, Swan Ganz catheter and the right internal jugular (IJ) superior vena cava (SVC) cannula (17 Fr Biomedicus arterial)
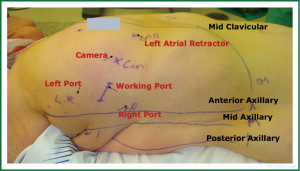
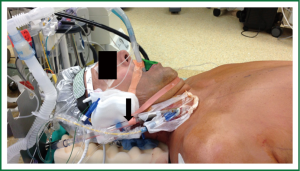
Vascular access & management of CPB
Venous return to the CPB circuit can be achieved in several ways. There are various commercially available long percutaneous venous return cannulas (22F-28F) that may be introduced up to the right atrium via the femoral venous system. After accessing the femoral vein, a long wire is advanced under TEE guidance into the right atrium. The venous cannula is then advanced over the wire into the right atrium with TEE confirmation. The SVC cannula is placed via the right IJ vein, under TEE guidance. Figures 3 & 4 demonstrate the neck and the groin venous cannulae in place.
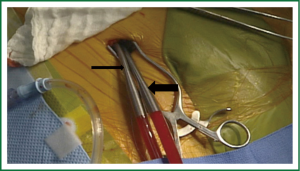
Adjunctive measures such as vacuum assistance are used to assist venous drainage when using long percutaneous cannulae and can increase flow dynamics by 20% to 40% (34). In fact, adequate total body venous drainage may be achieved with a single femoral venous cannula as long as the cannula’s multi-orificed side ports reside in the body of the right atrium with the tip in the proximal SVC. In our practice it is standard to place an additional percutaneous venous drainage cannula that resides in the SVC from the neck (Figure 3) and is positioned 2 cm proximal to the SVC-right atrial junction. This placement is best achieved via the right IJ vein given the near direct path it takes to the SVC.
The placement of a large-bore drainage catheter in the neck is not without substantial risk of vascular injury. Perforation of the right atrium, vena cava, or other vascular structure within the pericardium can lead to pericardial tamponade. Use of a strict Seldinger technique to minimize the chance of wire kinking during catheter advancement and use of TEE guidance for both wire and cannula placement is critical components for improving safety (35). Placement is facilitated by use of sequential dilators of increasing size in advance of the cannula. Ideally an assistant with sterile gown and gloves should be present.
A small dose of heparin should be administered just before IJ cannula placement. The cannula should then be flushed vigorously after it is secured to prevent any clotting or thrombus formation within it. Sutures may be placed around the cannulation site at this point or later, to facilitate closure of the defect and provide hemostasis upon cannula removal. When the right atrium must be opened during robotic mitral valve surgery (i.e., concomitant tricuspid valve repair), bicaval cannulation and occlusion are typically used to prevent associated air entrainment with an exposed right atrium. When the SVC is occluded, continuous monitoring of right IJ pressure is important to help ensure adequate SVC drainage from a percutaneous cannula.
Regardless of whether arterial outflow from the CPB circuit is achieved via percutaneous peripheral (Figure 4), or even direct central cannulation, use of TEE in guiding proper placement is key. We monitor the saturations of both the feet, in cases employing peripheral cannulation, using the NIRS probe (INVOS® Cerebral/Somatic oximeter) and a dual chamber monitor to record both cerebral and leg saturations simultaneously (Figure 5). A significant drop in peripheral saturation during the case (to <20% baseline) will prompt us to consider shunting the leg with a 5 Fr sheath introduced into the femoral artery distal to the cannulation site. The inflow to this shunt is through a 3 way tap pre-connected to the arterial cannula. This practice has allowed us to prevent any cases of significant limb ischemia in our series of patients.
Several complications associated with vascular access and retrograde arterial perfusion have been reported by Mohr and colleagues (36) with Port Access surgery. New York University Hospital describes an institutional drop in neurologic events from 4.7% to 1.2% during minimally invasive valvular surgical procedures, which they attribute to the avoidance of peripheral aortic cannulation (37). Murphy and colleagues (19) report the use of a screening protocol to identify and avoid femoral cannulation in patients considered at higher risk for retrograde embolization.
Regardless of approach, as already discussed, during aortic cannulation with Seldinger technique, the wire should be clearly observed on TEE to be intraluminal in the aorta prior. An elevation of arterial outflow-line pressure on the CPB circuit is often the first sign of aortic dissection. Immediate evaluation of the descending aorta with TEE should be made to rule out dissection. Unlike with standard direct surgical cannulation, retrograde aortic dissection may not be apparent in the surgical field until it extends up to the ascending aorta. Early recognition and conversion to antegrade central arterial flow may limit the damage of this potentially devastating complication.
Our preferred method for cross clamping the aorta is the angled Chitwood Transthoracic Aortic Cross Clamp (Scanlan International Inc, Minneapolis, MN), which is inserted percutaneously (Figure 6) and guided toward the ascending aorta under direct or endoscopic vision. Use of this technique over Port Access for aortic cross-clamping is based on several factors, not the least of which are decreases in infrastructure demands and costs (38) as well as shorter operative and cross-clamp times. A disadvantage is the need to place a separate aortic root vent percutaneously onto the ascending aorta for cardiac venting and antegrade cardioplegia administration. Figure 7 demonstrates the Chiwood clamp and the proximal aortic root vent/cardioplegia cannula in place.
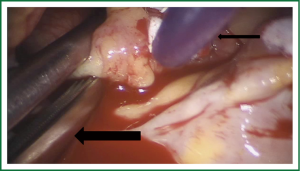
The Port Access system (Edwards Lifesciences, Irvine, CA) was designed to facilitate minimal access cardiac surgery and was introduced into clinical practice in 1997. A similar product is also produced by Estech (Danville, CA). At the cornerstone of this new technology was the development of the EndoClamp Aortic Catheter (Edwards Lifesciences), which is an endoluminal aortic balloon. The EndoClamp is placed through a side port of a Y-shaped large (21F or 24F) arterial cannula (EndoReturn Arterial Cannula) and advanced into the ascending aorta. This maneuver requires significant TEE guidance and a constant vigilance to look for balloon migration and malperfusion.
When evaluating studies comparing the overall safety of the two approaches, it is important to consider the more significant learning curve associated with Port Access surgery. The steepness of the curve was exemplified by the findings of the first Port Access International Registry, in which the incidence of iatrogenic aortic dissection was 1.3% in the first half of the study followed by a decrease to 0.2% in the second half (39).
Other alternatives to aortic cross-clamping exist, the simplest of which involves operating on a beating heart. The obvious advantage of this method is that it allows coronary flow to remain mostly uninterrupted. Disadvantages of this approach include a more disturbed surgical field and the possibility of systemic air embolization. In redo procedures or in presence of mild-moderate aortic insufficiency we have used cold fibrillatory arrest without aortic cross clamping with satisfactory results. One has to be cognizant about manipulating the left atrial retractor carefully and avoid worsening the aortic regurgitation. Fibrillation can be achieved either by a fibrillator applied directly to the heart or through the use of a pacing Swan Ganz catheter (40).
Cardioplegia delivery & solution of choice
We use a long combined aortic root vent cardioplegia cannula inserted directly through the working port incision across a 3-0 Gortex pursestring, securing it in the proximal ascending aorta for antegrade cardioplegia administration during our robotic cases.
An additional option is the use of a percutaneous coronary sinus catheter (41,42). The EndoPledge Coronary Sinus Catheter (Edwards Lifesciences) is advanced through an 11F introducer sheath, typically via the right IJ vein. The EndoPledge is a long triple-lumen catheter with a balloon on the end that allows for occlusion of the coronary sinus, measurement of distal pressure, and the infusion of cardioplegia. The obvious advantage of this approach is the ability to administer retrograde protection while remaining completely endoscopic. EndoPledge cannulation can be guided by TEE, fluoroscopy, or both. TEE is most commonly used to help direct the cannula into the coronary sinus ostia. Once in the coronary sinus, TEE is limited in its ability to exactly define the position of the tip, and provides limited functional information. The generation of a “ventricularized” distal pressure waveform during inflation of the occlusion balloon suggests adequate sinus occlusion. The cannula should be advanced until this ventricularized waveform is seen with balloon inflation. The cannula is deemed to be inserted too far if ventricularization occurs with less than 0.75 to 1 mL of balloon inflation and not far enough if more than 1 mL is required for occlusion.
Though conceptually appealing, the use of the EndoPledge cannula has not been widely adopted with robotic aided surgeries. Concerns about coronary sinus perforation risk, increased complexity of placement, and a high rate of dislodgment have limited its adoption. However the most important reason for avoidance of the EndoPledge cannula is that many surgeons do not believe that retrograde cardioplegia is necessary for cardiac protection when adequate antegrade cardioplegia can be delivered; this has been demonstrated in several case series (43,44).
Our preferred cardioplegia solution for robotically assisted mitral valve surgery is Custodiol® HTK Solution. As a crystalloid solution, Custodiol® HTK Solution is considered an intracellular solution, containing low concentrations of the electrolytes sodium, calcium, potassium and magnesium. It contains a high concentration of an amino acid buffering agent, histidine/histidine hydrochloride, the amino acid tryptophan a-ketoglutarate, and the osmotic agent mannitol. The histidine/histidine hydrochloride buffering system provides normal osmolarity and enhances the solution’s buffering capacity. A single cold 2-2.5 L dose allows electromechanical silence for upto an hour and allows surgeon to focus on surgery uninterrupted. The infusate should be administered slowly over 10-15 minutes. Top up doses of 100-300 cc may be administered on return of ECG activity or after an hour of ischemia time. The large volume low sodium infusate in Custodiol® HTK often drops the serum sodium and pH and the perfusionists needs to pay attention to correct them. Standard blood cardioplegia is still a viable alternative however the need to interrupt surgery for frequent top-up and increased chance of instilling air into the coronaries during the top-up dose instillation down an air filled aortic root are reasons for our team switching to Custodiol® HTK in line with the safety and efficacy data published (45).
Robotic assisted mitral valve surgery in redo situations
Previous cardiac surgery does not preclude robotic approach and in fact, in well selected patients may provide some significant advantages. Multiple studies have shown at least equal if not better mortality with redo right thoracotomy approach in comparison with redo sternotomy mitral valve surgery (46-48). Reduction in infection rate, transfusion requirement, and length of hospital stay were consistently shown in these studies. The Cleveland Clinic group reported a higher incidence of repair failure and stroke in 80 redo right thoracotomy approach mitral valve surgery patients compared with a similar group of 2,444 reoperative mitral repair, performed via sternotomy approach. Mortality between the two groups was similar (6.7% and 6.3%) (49). At East Carolina University, our group (49) reported a 3% thirty day mortality in 167 patients with previous sternotomy operated using right anterolateral thoracotomy approach over a 15 year period. This series comprised of 19 robotic cases. During the last five years examined, the 30-day mortality dropped to 0% in 85 patients with a low stroke rate. A higher than expected incidence of pneumonia, with 28% isolated to the right lung was noted in this series.
Management of patients with patent left internal mammary (LIMA) coronary bypass grafts requires some mention, although in practice it differs little from patients undergoing sternotomy. The ability to clamp the LIMA and prevent myocardial warming is limited, thus management strategies revolve around more aggressive systemic cooling or “beating-heart” surgical strategies (49-51).
The presence of anterior chest-wall adhesions from previous sternotomy may limit exposure to the right ventricle, preventing the ability to apply temporary epicardial pacing wires, and this may be particularly true when performing true endoscopic surgery. The preoperative placement of a pacing Swan Ganz catheter can alleviate this problem. Similarly, the combination of previous cardiac surgery and robotic cardiac surgery may also create difficulties in achieving caval occlusion if the right side of the heart must be entered. Placement of endoscopic bulldog clips on each cava during robotic aided surgery has also been reported (52). Aggressive use of assisted venous drainage, with a hard shell venous reservoir in the CPB circuit and the venous cannulae withdrawn into the IVC and SVC can also help manage the situation without recourse to caval occlusion.
Mitral valve exposure and repair
Exposure and visualization of the mitral valve during robotic surgery varies from the standard sternotomy approach and has been detailed in our previous publications (24). Specialized left atrial retractors have been developed to expose the mitral valve (Figure 8). We measure the anterior mitral valve leaflet to confirm the size of annuloplasty ring that we will use (Figure 9). Our current practice at East Carolina Heart Institute is to first perform the leaflet repair. Over the years we have moved from away from extensive resections to current practice of respecting the leaflet integrity. We excise the minimal amount of prolapsing leaflet segment tissue “Haircut resection technique” (53) ensuring that no part of the reconstructed leaflet is unsupported by chord (Figures 10,11). If need be, we will re-implant/transfer chords or insert Gortex neo-chords to support the leaflet segment. Subsequently we will complete the ring implantation using interrupted suture technique. We progress by securing each suture with Cor-KnotTM before moving to the next annuloplasty suture (Figure 12). Following ring implantation we will perform saline testing to check valve competence and then tighten the foldoplasty/neo-chord sutures. The robotic video imaging system greatly enhances field lighting and visualization of the mitral valve. Figure 13 shows a competent valve after repair with a well seated ring secured by Cor-KnotTM. The additional monitors in the operating room allow all members of the operative team to observe and even participate in the mitral valve repair.
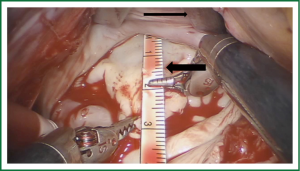
Weaning from CPB and transfer to ICU
During separation from CPB, in principle there are no dramatic differences from open operations. However, if increased cross-clamp and CPB times occur, this may need to be factored into decision making regarding post-CPB pharmacologic, ventilatory, fluid, and blood-product management. After a period of reperfusion, preparations are made and any requirements for pacing and hemodynamic support are adjusted. If a pacing PAC is being used, adjustments in position may need to be made because once the heart fills, the wire may migrate away from the ventricular wall. The amplitude may also need to be increased if the ventricular pacing threshold has increased. Initial ventilation of both lungs should be instituted when possible. Attempts to de-air the heart are made in the usual fashion. The ability to dislodge retained left atrial air is typically not hampered by the incision, but exposure of the left ventricular apex may be. Consideration for leaving a LV vent across the mitral valve should be made in patients with pre-existing aortic regurgitation especially in those with mild degree of LV impairment/ hypertrophy.
Given the close proximity of the noncoronary leaflet of the aortic valve to the anterior mitral valve annulus, a directed TEE examination of the aortic valve after CPB is also important. Similarly, the proximity of the circumflex coronary artery to the posterior and lateral annulus requires assessment of ventricular wall motion. The patency of right sided pulmonary veins should be checked before separation from bypass and similarly presence of a freely moving PAC is mandatory before discontinuation of CPB.
During the initiation of surgical chest closure, single lung ventilation is typically needed. Superimposing this additional strain on the right ventricle in patients with preexisting dysfunction or residual myocardial stunning may lead to decompensation. Measures such as positive end-expiratory pressure to the ventilated lung or intermittent lung inflation may be necessary.
At the conclusion of surgery there are several preparations the anesthesia team must make before transport that are not usually necessary following sternotomy. First, if a double-lumen endotracheal tube has been used, it should be switched to a single lumen tube. The use of an airway-exchange catheter may be helpful. Adequate hemostasis should be secured at the RIJ SVC cannulation site, usually with a box stitch.
Adequacy of peripheral pulses on the side of cannulated femoral artery should be checked before leaving the room.
Postoperative pain management
Although many of the patients for robotic mitral valve surgery are excellent candidates for early extubation, caution must still be exercised because of the potential occurrence of significant postoperative chest-wall bleeding, given the multiple chest-wall puncture sites.
The simplest adjunctive pain-management technique involves the use of limited intercostal injections of local anesthetic during surgical closure. The desire for a longer duration of local anesthesia has led many to use continuous infusions via temporary catheters and specialized pumps. Catheters may simply be left under the subcutaneous tissue before closure and then tunneled out through the skin (54,55). An extrapleural intercostal catheter can also be placed under direct vision, and may also provide improvements in pain control and, possibly, pulmonary function (56). The inability to adequately strip the parietal pleura posteriorly beyond the incision may limit optimal positioning of the catheter tip.
Conclusions
Robotically aided mitral valve surgery is rapidly becoming the “gold-standard” and its outcomes are being proposed for use as benchmark for valve repair quality related outcome measures (57). Patients are experiencing a decrease in pain and faster recoveries without sacrificing the durability of their surgical repair. Most importantly, these results can be achieved with an equivalent morbidity and mortality in comparison with standard mitral valve surgery. The recent publication from the Mayo Clinic group (58) also suggests that with systems engineering approach, even a capital intensive technique like robotic mitral valve surgery can be made cost effective. At present, the majority of robotic cases are performed at only a few centers of excellence and although definitive advantages of robotic surgery over non-robotic-assisted minimally invasive surgery have yet to be shown, expansion of robotic aided minimally invasive cardiac surgery seems inevitable, fueled by continued technological advancements and patient demands. It is important to acknowledge that consistent successful outcomes require a significant investment in training and experience for the whole “robotic team”.
Acknowledgements
Disclosure: The authors declare no conflict of interest.
References
- Suri RM, Antiel RM, Burkhart HM, et al. Quality of life after early mitral valve repair using conventional and robotic approaches. Ann Thorac Surg 2012;93:761-9. [PubMed]
- Arom KV, Emery RW. Minimally invasive mitral operations. Ann Thorac Surg 1997;63:1219-20. [PubMed]
- Chitwood WR Jr, Wixon CL, Elbeery JR, et al. Video-assisted minimally invasive mitral valve surgery. J Thorac Cardiovasc Surg 1997;114:773-80; discussion 780-2. [PubMed]
- Chitwood WR Jr, Elbeery JR, Moran JF. Minimally invasive mitral valve repair using transthoracic aortic occlusion. Ann Thorac Surg 1997;63:1477-9. [PubMed]
- Mohr FW, Falk V, Diegeler A, et al. Minimally invasive port-access mitral valve surgery. J Thorac Cardiovasc Surg 1998;115:567-74; discussion 574-6. [PubMed]
- Navia JL, Cosgrove DM 3rd. Minimally invasive mitral valve operations. Ann Thorac Surg 1996;62:1542-4. [PubMed]
- Schwartz DS, Ribakove GH, Grossi EA, et al. Minimally invasive cardiopulmonary bypass with cardioplegic arrest a closed chest technique with equivalent myocardial protection. J Thorac Cardiovasc Surg 1996;111:556-66. [PubMed]
- Glower DD, Siegel LC, Frischmeyer KJ, et al. Predictors of outcome in a multicenter port-access valve registry. Ann Thorac Surg 2000;70:1054-9. [PubMed]
- Gammie JS, Zhao Y, Peterson ED, et al. J. Maxwell Chamberlain Memorial Paper for adult cardiac surgery. Less-invasive mitral valve operations trends and outcomes from the Society of Thoracic Surgeons Adult Cardiac Surgery Database. Ann Thorac Surg 2010;90:1401-8, 1410.e1; discussion 1408-10.
- Smith JM, Stein H, Engel AM, et al. Totally endoscopic mitral valve repair using a robotic-controlled atrial retractor. Ann Thorac Surg 2007;84:633-7. [PubMed]
- Kiliç L, Şenay Ş, Ümit Güllü A, et al. Leyla loop a time-saving suture technique for robotic atrial closure. Interact Cardiovasc Thorac Surg 2013;17:579-80. [PubMed]
- Carpentier A, Loulmet D, Aupècle B, et al. Computer assisted open heart surgery. First case operated on with success. C R Acad Sci III 1998;321:437-42. [PubMed]
- Chitwood WR Jr, Nifong LW, Elbeery JE, et al. Robotic mitral valve repair trapezoidal resection and prosthetic annuloplasty with the da vinci surgical system. J Thorac Cardiovasc Surg 2000;120:1171-2. [PubMed]
- Nifong LW, Chitwood WR, Pappas PS, et al. Robotic mitral valve surgery a United States multicenter trial. J Thorac Cardiovasc Surg 2005;129:1395-404. [PubMed]
- Modi P, Hassan A, Chitwood WR Jr. Minimally invasive mitral valve surgery a systematic review and meta-analysis. Eur J Cardiothorac Surg 2008;34:943-52. [PubMed]
- Yamada T, Ochiai R, Takeda J, et al. Comparison of early postoperative quality of life in minimally invasive versus conventional valve surgery. J Anesth 2003;17:171-6. [PubMed]
- Walther T, Falk V, Metz S, et al. Pain and quality of life after minimally invasive versus conventional cardiac surgery. Ann Thorac Surg 1999;67:1643-7. [PubMed]
- Vleissis AA, Bolling SF. Mini-reoperative mitral valve surgery. J Card Surg 1998;13:468-70. [PubMed]
- Murphy DA, Miller JS, Langford DA, et al. Endoscopic robotic mitral valve surgery. J Thorac Cardiovasc Surg 2006;132:776-81. [PubMed]
- Cheng DC, Martin J, Lal A, et al. Minimally invasive versus conventional open mitral valve surgery: a meta-analysis and systematic review. Innovations (Phila) 2011;6:84-103. [PubMed]
- Suri RM, Schaff HV, Dearani JA, et al. Survival advantage and improved durability of mitral repair for leaflet prolapse subsets in the current era. Ann Thorac Surg 2006;82:819-26. [PubMed]
- Galloway AC, Schwartz CF, Ribakove GH, et al. A decade of minimally invasive mitral repair: long-term outcomes. Ann Thorac Surg 2009;88:1180-4. [PubMed]
- Iribarne A, Karpenko A, Russo MJ, et al. Eight-year experience with minimally invasive cardiothoracic surgery. World J Surg 2010;34:611-5. [PubMed]
- Nifong LW, Rodriguez E, Chitwood WR Jr. 540 consecutive robotic mitral valve repairs including concomitant atrial fibrillation cryoablation. Ann Thorac Surg 2012;94:38-42; discussion 43. [PubMed]
- Charland PJ, Robbins T, Rodriguez E, et al. Learning curve analysis of mitral valve repair using telemanipulative technology. J Thorac Cardiovasc Surg 2011;142:404-10. [PubMed]
- Cheng W, Fontana GP, De Robertis MA, et al. Is robotic mitral valve repair a reproducible approach? J Thorac Cardiovasc Surg 2010;139:628-33. [PubMed]
- Chitwood WR Jr, Rodriguez E, Chu MW, et al. Robotic mitral valve repairs in 300 patients: a single-center experience. J Thorac Cardiovasc Surg 2008;136:436-41. [PubMed]
- Moodley S, Schoenhagen P, Gillinov AM, et al. Preoperative multidetector computed tomograpy angiography for planning of minimally invasive robotic mitral valve surgery: impact on decision making. J Thorac Cardiovasc Surg 2013;146:262-8.e1.
- Eltzschig HK, Rosenberger P, Löffler M, et al. Impact of intraoperative transesophageal echocardiography on surgical decisions in 12,566 patients undergoing cardiac surgery. Ann Thorac Surg 2008;85:845-52. [PubMed]
- Minhaj M, Patel K, Muzic D, et al. The effect of routine intraoperative transesophageal echocardiography on surgical management. J Cardiothorac Vasc Anesth 2007;21:800-4. [PubMed]
- Freeman WK, Schaff HV, Khandheria BK, et al. Intraoperative evaluation of mitral valve regurgitation and repair by transesophageal echocardiography: incidence and significance of systolic anterior motion. J Am Coll Cardiol 1992;20:599-609. [PubMed]
- Wang Y, Gao CQ, Wang JL, et al. The role of intraoperative transesophageal echocardiography in robotic mitral valve repair. Echocardiography 2011;28:85-91. [PubMed]
- Jassar AS, Brinster CJ, Vergnat M, et al. Quantitative mitral valve modeling using real-time three-dimensional echocardiography: technique and repeatability. Ann Thorac Surg 2011;91:165-71. [PubMed]
- Toomasian JM, McCarthy JP. Total extrathoracic cardiopulmonary support with kinetic assisted venous drainage: experience in 50 patients. Perfusion 1998;13:137-43. [PubMed]
- Chaney MA, Minhaj MM, Patel K, et al. Transoesophageal echocardiography and central line insertion. Ann Card Anaesth 2007;10:127-31. [PubMed]
- Mohr FW, Onnasch JF, Falk V, et al. The evolution of minimally invasive valve surgery--2 year experience. Eur J Cardiothorac Surg 1999;15:233-8; discussion 238-9. [PubMed]
- Grossi EA, Loulmet DF, Schwartz CF, et al. Evolution of operative techniques and perfusion strategies for minimally invasive mitral valve repair. J Thorac Cardiovasc Surg 2012;143:S68-70. [PubMed]
- Reichenspurner H, Detter C, Deuse T, et al. Video and robotic-assisted minimally invasive mitral valve surgery: a comparison of the Port-Access and transthoracic clamp techniques. Ann Thorac Surg 2005;79:485-90; discussion 490-1. [PubMed]
- Galloway AC, Shemin RJ, Glower DD, et al. First report of the Port Access International Registry. Ann Thorac Surg 1999;67:51-6; discussion 57-8. [PubMed]
- Levin R, Leacche M, Petracek MR, et al. Extending the use of the pacing pulmonary artery catheter for safe minimally invasive cardiac surgery. J Cardiothorac Vasc Anesth 2010;24:568-73. [PubMed]
- Siegel LC. Coronary sinus catheterization for minimally invasive cardiac surgery. Anesthesiology 1999;90:1232-3. [PubMed]
- Plotkin IM, Collard CD, Aranki SF, et al. Percutaneous coronary sinus cannulation guided by transesophageal echocardiography. Ann Thorac Surg 1998;66:2085-7. [PubMed]
- Lebon JS, Couture P, Rochon AG, et al. The endovascular coronary sinus catheter in minimally invasive mitral and tricuspid valve surgery: a case series. J Cardiothorac Vasc Anesth 2010;24:746-51. [PubMed]
- Casselman FP, La Meir M, Jeanmart H, et al. Endoscopic mitral and tricuspid valve surgery after previous cardiac surgery. Circulation 2007;116:I270-5. [PubMed]
- Viana FF, Shi WY, Hayward PA, et al. Custodiol versus blood cardioplegia in complex cardiac operations: an Australian experience. Eur J Cardiothorac Surg 2013;43:526-31. [PubMed]
- Sharony R, Grossi EA, Saunders PC, et al. Minimally invasive reoperative isolated valve surgery: early and mid-term results. J Card Surg 2006;21:240-4. [PubMed]
- Bolotin G, Kypson AP, Reade CC, et al. Should a video-assisted mini-thoracotomy be the approach of choice for reoperative mitral valve surgery? J Heart Valve Dis 2004;13:155-8; discussion 158. [PubMed]
- Burfeind WR, Glower DD, Davis RD, et al. Mitral surgery after prior cardiac operation: port-access versus sternotomy or thoracotomy. Ann Thorac Surg 2002;74:S1323-5. [PubMed]
- Arcidi JM Jr, Rodriguez E, Elbeery JR, et al. Fifteen-year experience with minimally invasive approach for reoperations involving the mitral valve. J Thorac Cardiovasc Surg 2012;143:1062-8. [PubMed]
- Svensson LG, Gillinov AM, Blackstone EH, et al. Does right thoracotomy increase the risk of mitral valve reoperation? J Thorac Cardiovasc Surg 2007;134:677-82. [PubMed]
- Umakanthan R, Petracek MR, Leacche M, et al. Minimally invasive right lateral thoracotomy without aortic cross-clamping: an attractive alternative to repeat sternotomy for reoperative mitral valve surgery. J Heart Valve Dis 2010;19:236-43. [PubMed]
- Gullu AU, Senay S, Kocyigit M, et al. A simple method for occlusion of both venae cavae in total cardiopulmonary bypass for robotic surgery. Interact Cardiovasc Thorac Surg 2012;14:138-9. [PubMed]
- Chu MW, Gersch KA, Rodriguez E, et al. Robotic “haircut” mitral valve repair: posterior leaflet-plasty. Ann Thorac Surg 2008;85:1460-2. [PubMed]
- Sostaric M, Gersak B, Novak-Jankovic V. The analgesic efficacy of local anesthetics for the incisional administration following port access heart surgery: bupivacaine versus ropivacaine. Heart Surg Forum 2010;13:E96-E100. [PubMed]
- Sostaric M. Incisional administration of local anesthetic provides satisfactory analgesia following port access heart surgery. Heart Surg Forum 2005;8:E406-8. [PubMed]
- Ganapathy S. Anaesthesia for minimally invasive cardiac surgery. Best Pract Res Clin Anaesthesiol 2002;16:63-80. [PubMed]
- Suri RM, Burkhart HM, Daly RC, et al. Robotic mitral valve repair for all prolapse subsets using techniques identical to open valvuloplasty: establishing the benchmark against which percutaneous interventions should be judged. J Thorac Cardiovasc Surg 2011;142:970-9. [PubMed]
- Suri RM, Thompson JE, Burkhart HM, et al. Improving affordability through innovation in the surgical treatment of mitral valve disease. Mayo Clin Proc 2013;88:1075-84. [PubMed]