Is a clinical target volume (CTV) necessary for locally advanced non-small cell lung cancer treated with intensity-modulated radiotherapy? —a dosimetric evaluation of three different treatment plans
Introduction
Radiotherapy is one of the most important therapeutic strategies for patients with locally advanced non-small cell lung cancer (NSCLC) but the long-term outcomes following treatment remain dismal (1). To examine whether increasing the intensity of radiotherapy improves both local-regional control and overall survival, several trials, initiated by the Radiation Therapy Oncology Group (RTOG), were conducted in which the safety and efficacy of increasing the total radiation dose were determined. However, in the phase III trial RTOG0617 (2), a higher radiation therapy dose of 74 Gy did not improve overall survival and may even have been harmful, because of the increased dose delivered to adjacent normal tissues. Thus, efforts are currently being directed at ensuring an effective radiation dose escalation while reducing the irradiated volumes to limit treatment-associated toxicities.
The clinical target volume (CTV) is defined as the tissue volume that contains the gross tumor volume (GTV) and subclinical microscopic malignant lesions. Decades of experience led to the conclusion that 45–50 Gy results in high control rates for subclinical disease in patients with epithelial tumors (3-6). However, recent studies have shown that omission of the CTV in lung cancer radiotherapy does not compromise local tumor control (7,8). This latter result may reflect sufficient dose coverage to the subclinical disease extent within certain margins by delivering volumes in which at least 60 Gy were administered for gross tumor eradication. Importantly, irradiation of a smaller target volume would improve normal-tissue sparing and thus allow dose escalation to achieve better tumor control (9,10). However, while the potential clinical benefits of CTV omission have been pointed out, its feasibility from a dosimetric perspective has not been evaluated. The aim of this study was to compare the dosimetric characteristics of the different approaches to treatment planning, with or without CTV implementation, in patients with locally advanced NSCLC.
Methods
Ethics statement
Local ethical principles have been reviewed in relation to this work and no ethical issues have been found to apply, as this is a retrospective dosimetric study with no involvement of impact on individuals. Thus, the Institutional Review Board had waived the requirement for written approval of this study.
Patient characteristics
Clinical cases representative of the different, commonly occurring tumor anatomies were analyzed in this small-scale study. The characteristics of the 13 patients included in the study are summarized in Table 1. All of the patients had pathologically confirmed stage III NSCLC according to the American Joint Committee on Cancer (7th edition) and were treated with conventionally fractionated involved-field radiotherapy.
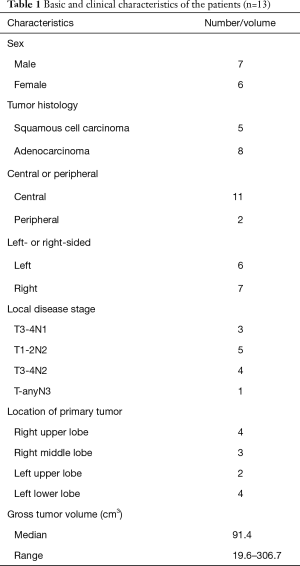
Full table
Computed tomography (CT) simulation
Patients were immobilized in the supine position, with their arms positioned above their head or beside their body. A vacuum bag or a thermoplastic mask was used to immobilize the thoracic region. A planning CT scan at 5-mm intervals from the mid-neck to the diaphragm with contrast enhancement was obtained using an AcQsim CT simulator (Philips Medical Systems, Fitchburg, WI, USA) for each patient during free-breathing. The acquired images were transferred to the Pinnacle version 8.0 treatment planning system (Philips Medical Systems, Fitchburg, WI, USA) for target and organs at risk (OAR) delineation.
Target and OARs delineation
The GTV was defined as the primary tumor displayed in the lung window together with the involved lymph nodes (short axis of >1 cm) seen on either the enhanced CT or pretreatment positron emission tomography (PET) scan. In our routine clinical practice, the CTV is equivalent to the GTV as applied in some RTOG studies (10,11). Determination of the planning target volume (PTV) took into account of tumor movement during free breathing, as observed under fluoroscopy in the right-left, the superoinferior, and the anteroposterior directions, and uncertainty in patient positioning. It is observed that there is a median of 5-mm tumor motion. In addition, the setup errors were assessed to be no more than 5 mm according to bony anatomy translation before image guidance [we used electronic portal imaging device (EPID) prior to treatment to assess patient positioning] and our experience. Thus, to form the PTV, at least 1 cm of margin was added to the GTV (CTV) in the axial and superior-inferior directions (5 mm for tumor motion and 5 mm for set up errors) and the margins can be further larger according to different tumor motion magnitude of each case.
To create new CTV plans, the CTV for each patient was re-contoured as follows: for primary lung lesions, CTV margins were 0.6 cm beyond the GTV for squamous cell cancer and 0.8 cm for adenocarcinoma (12). For involved lymph nodes, CTV margins were 0.3 cm beyond the GTV for nodes with a short axis <2 cm, and 0.5 cm for those with a short axis ≥2 cm (13). Notably, the CTVs were manually modified to exclude the vertebral bodies, trachea, proximal bronchial trees, heart, large vessels, and esophagus. The PTV was then generated by adding a margin to the CTV as described for the routine plan. In the following, the PTV generated from the GTV (without the CTV) is referred to as the PTV_g, and the PTV generated from the CTV as the PTV_c. The PTV_g and PTV_c volumes are shown in Figure 1. The OARs were the lung (defined as the bilateral lung minus the GTV), spinal cord, esophagus, and heart.
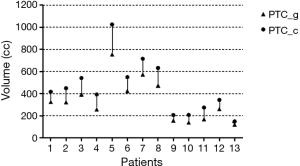
Intensity-modulated radiotherapy (IMRT) planning
Three IMRT plans were generated: plan_routine, plan_CTV, and plan_SIB. During routinely performed planning in our hospital (plan_routine), a dose of 60 Gy is prescribed in 30 fractions with respect to the isodose line, with the requirement that at least 95% of the PTV_g receives the prescribed dose. The plans in this study were generated using the step-and-shoot technique and the Pinnacle planning system (Phillips Medical Systems). Five to nine coplanar 6-MV photon fields from a Synergy (Elekta Oncology Systems, Crawley, UK) accelerator were created. Beam arrangements were optimized for each patient according to the tumor’s location and relationship with the surrounding normal tissues. Adaptive convolution was used to calculate dose distributions, optimizing the plan by applying the Direct Machine Parameter Optimization algorithm. In setting the optimization objectives, PTV coverage was assigned the highest priority, followed by the avoidance of an excessive dose to OAR. The parameters of dose constraints were summarized in Table 2.
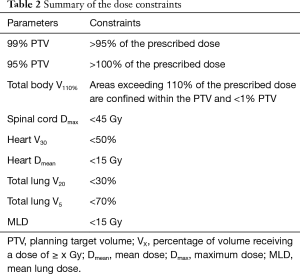
Full table
Plan_CTV was generated similarly to plan_routine, but with the requirement that at least 95% of the PTV_c received the prescribed 60 Gy in 30 fractions.
In plan_SIB, we used the simultaneous integrated boost (SIB) technique to prescribe adequate doses to the PTV_g and PTV_c simultaneously. Thus the generation of plan_SIB was similar to that of the other two plans except that the PTV_c received 50 Gy and the dose to the PTV_g was simultaneously escalated to 60 Gy in 30 fractions.
Comparison of the three plans
The final dose distribution in each plan was normalized to 95% coverage of the PTV receiving the prescribed dose. Dose coverage of the PTVs by the three plans was compared using two endpoints: V60 (volume receiving at least 60 Gy) and V50 (volume receiving at least 50 Gy). Various dose-volume parameters for specific OARs were generated to assess the effectiveness of the three plans in OAR sparing. The lung (bilateral lungs minus the GTV) was evaluated using V5, V20, and the mean lung dose (MLD) as predictors of lung toxicity. The esophagus was appraised according to the maximum dose, the mean dose, and V55. The heart was assessed in terms of V5, V20, V30, and the mean dose, and the spinal cord in terms of its maximum dose and maximum dose to 1 cm3.
Statistical analysis
The data were analyzed using the statistical software SPSS 19.0 (SPSS, Inc., Chicago, IL, USA). The equality of means for continuous variables was assessed in t-tests. A P value ≤0.05 was considered to indicate statistical significance. Statistical tests were based on a two-sided significance level.
Results
All of the plans created by the three planning methods fulfilled the specified dose constraints. Table 3 summarizes the dose coverage parameters of the three plans. Excellent target coverage was consistently achieved, with ≥95% of the PTVs receiving at least 100% of the prescription dose. Importantly, the 50 Gy isodose line of plan_routine covered at least 95% of the PTV_c volume in all 13 patients, suggesting sufficient irradiation to the regions of subclinical disease. The isodose distributions of the three plans for a representative patient are shown in Figure 2 as an example.
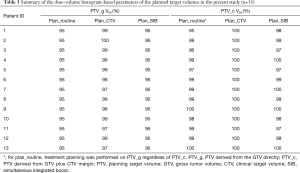
Full table
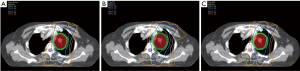
The numerical statistics for OAR calculated from the dose-volume histogram (DVH) are provided in Table 4. Generally, the statistics showed better OAR-sparing in plan_routine than in plan_CTV, and the best OAR sparing in plan_SIB.
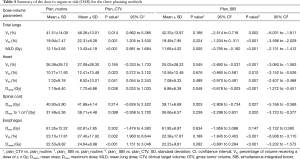
Full table
Plan_CTV vs. plan_routine
In plan_CTV vs. plan_routine, the V5 and V20 of the lung were increased by 4.8% and 2.4%, respectively, and the MLD was ~1.3 Gy higher. The differences were statistically significant. The V20 and V30 of the heart were 1.7% and 1.2% higher, respectively, and the mean dose to the heart was ~0.5 Gy higher. These differences were also statistically significant. The V5 of the heart were 0.7% higher with no statistical significance. The maximum doses to the spinal cord and to 1 cm3 of the spinal cord generated by plan_routine were 40.93±5.90 and 37.49±5.36 Gy, respectively, with non-significant increases to 41.88±4.14 and 38.71±4.48 Gy obtained with plan_CTV. The V55 and mean dose to the esophagus were 4.3% and 2.1 Gy higher in plan_CTV than in plan_routine. The differences were statistically significant.
Plan_SIB vs. the others plans
Among the three plans, plan_SIB resulted in the best OAR sparing. The MLD, mean dose to the heart, the V5 of the heart and maximum dose to the spinal cord were significantly lower in plan_SIB than in plan_routine. All the listed parameters were significantly lower in plan_SIB than in plan_CTV except for the maximum dose to the esophagus.
Discussion
The aim of this retrospective dosimetric analysis was to investigate the feasibility of omitting CTVs in IMRT plans for the treatment of locally advanced NSCLC. Comparisons of the dosimetric characteristics of the different treatment plans showed that the IMRT plan without CTV delivered sufficient doses to subclinical lesions, with better sparing of normal tissues than achieved with CTV implementation. Further reductions in the doses to surrounding normal tissues were possible with the plan using the SIB technique. To the best of our knowledge, this is the first study to provide dosimetric evidence supporting the omission of CTV in radiotherapy for NSCLC patients.
The first priority of our study was to determine whether IMRT without targeting the CTV delivers a radiation dose adequate for the eradication of subclinical disease. From a radiobiological perspective, the doses necessary to sterilize subclinical foci of common epithelial tumors should be lower than those used to eradicate gross tumors. Fletcher (3) showed that doses of 45–50 Gy in 2-Gy fractions resulted in high rates of control for surgically undisturbed lymph node metastases in head and neck cancer and in breast cancer. Studies of patients who underwent elective radiation for various tumors (4) showed that the dose-response curve for the control of subclinical metastases had a lower threshold and a shallower slope than the curves characteristic for the control of macroscopic disease, indicating that subclinical disease could be effectively eliminated with doses of 45–50 Gy. A similar conclusion was reached by Okunieff et al. (5) based on an analysis of 90 dose-response curves from macroscopic tumor therapies and elective therapies. The doses to control microscopic disease were ~12 Gy less than the doses required to control macroscopic disease. In addition to data from elective irradiation, recent studies have suggested that even incidental nodal irradiation with much lower doses can effectively control microscopic disease. In their study of node-negative NSCLC patients treated with three-dimensional conformal radiotherapy (3DCRT), Zhao et al. (14) reported that equivalent, uniform, incidental irradiation doses of 9.6, 22.6, and 62.9 Gy produce excellent local control in high-risk nodal regions. As shown by our routine IMRT plans, the isodose line of 50 Gy autonomously covered at least 95% of PTV_c in all cases. Taken together, these results substantiated the feasibility, at least from a biological perspective, of omitting the CTV.
An important goal of omitting the GTV-to-CTV expansion is to decrease the radiation volume and thus deliver less radiation to adjacent normal tissues. In our study, dose volume parameters were significantly lower with plan_routine than with plan_CTV for the lung, heart, and esophagus. The doses to the spinal cord delivered according to the two plans were similar because they were not considered a priority as long as the maximum doses were limited to ≤45 Gy. In the phase II studies that preceded RTOG0617, in which CTV was omitted (10), 74 Gy was shown to be safe and beneficial for patient survival. Therefore, our results support CTV omission based on the benefits conferred by limiting the toxic dose to normal tissues while facilitating the implementation of further dose-escalation strategies.
Retrospective data also showed that CTV omission in lung cancer radiotherapy would not compromise tumor control. Liang et al. (8) reviewed 105 patients with stage III NSCLC treated with IMRT and found similar rates of local recurrence, distant metastasis, progression-free survival, and overall survival in patients treated with or without CTV implementation, but the rate of grade 3–4 radiation pneumonitis was significantly lower in the latter group (18.0% vs. 5.5%). Kilburn et al. (15) reviewed local recurrence in 110 NSCLC patients treated with either three-dimensional conformal radiotherapy or IMRT without CTV expansion. After retrospectively applying a 1-cm uniform expansion from the PTV, only two cases of failed treatment were determined in the retrospectively derived CTV expansion, whereas the majority (86%) of the failures could be attributed to the original PTV in which the full prescribed dose was delivered. This suggests that failures at the primary site are a consequence of ineffective tumor cell killing and not of inappropriate target coverage.
In our opinion, once CTV is implemented in a treatment plan, it is reasonable to prescribe delivery of the dose required to eradicate subclinical disease. This may account for the success achieved with the SIB since it enabled the simultaneous delivery of different doses to different areas of the treatment volume. Compared to plan_routine and plan_CTV, plan_SIB provided the adequate dose coverage of PTV_g and PTV_c while further reducing the doses to the lung, heart, and spinal cord. The SIB technique has already been tested in clinical trials involving patients with locally advanced lung cancer. In a retrospective study of patients with locally advanced stage III NSCLC (16), SIB-IMRT was well tolerated by 40 out of 48 patients when the dose to the GTV was escalated to >60 Gy; the median survival time achieved with this regimen was 21 months, considered as good. Given the advantages of the SIB technique, we are currently designing a prospective study to evaluate its potential benefits in lung cancer patients.
One limitation of this study is that we did not perform 4D-CT simulation which may help reducing PTV margins. One reason is that at this moment 4D-CT has not been routinely used in our center for locally-advanced NSCLC, as in many radiotherapy departments in China, due to equipment insufficiency and heavy workload. Instead, we use fluoroscopy to assess tumor motion during the free breathing cycle. In this way, tumor localization was measured by tumor itself when visible as well as anatomic surrogates such as carina or diaphragm. It is reported that good correlation between the position of these surrogates and tumor can be achieved with linear models and the average prediction error can be less than 1 mm (17,18). Therefore, we think fluoroscopy can be generally adequate for tracking tumor location in this study. In addition, patient positioning was assessed prior to treatment by the EPID according to the locations of anatomical landmarks on the EPID image. We think that on-board imaging techniques such like cone beam CT (CBCT) would be better to define 3D anatomy, yet at present we are not able to apply CBCT in every patient’s treatment due to the problem of equipment insufficiency and heavy workload, the same as 4D-CT implementation. Given that PTV_g and PTV_c were affected by the same degree with these methods, we believe that our dosimetric results comparing three plans stay reliable although 4D-CT simulation or CBCT imaging was not applied.
Conclusions
In summary, our IMRT planning series illustrated that plans limited to targeting GTVs with 60 Gy in 30 fractions autonomously provide a sufficient dose to eradicate subclinical lesions while enabling better sparing of normal tissue than plans that include CTV implementation. Furthermore, we demonstrated that SIB-IMRT can ensure adequate doses to both the gross tumor and subclinical disease while further reducing toxicity to critical structures. Thus, for patients with stage III NSCLC treated with IMRT planning, the omission of CTV is feasible. In fact, the therapeutic ratio is theoretically improved by omitting CTV. Based on the results reported herein, this strategy should be considered in the design of future clinical trials.
Acknowledgements
We would like to thank all the physicians and physicists in Department of Radiation Oncology, Fudan University Shanghai Cancer Center for their assistance in this research.
Funding: This project was supported by Shanghai Pujiang Program (No. 15PJD008 to Z.ZF) and the National Science Foundation of China (No. 81572963 to Z.ZF).
Footnote
Conflicts of Interest: The authors have no conflicts of interest to declare.
Ethical Statement: Local ethical principles have been reviewed in relation to this work and no ethical issues have been found to apply, as this is a retrospective dosimetric study with no involvement of impact on individuals. Thus, the Institutional Review Board had waived the requirement for written approval of this study.
References
- Curran WJ Jr, Paulus R, Langer CJ, et al. Sequential vs. concurrent chemoradiation for stage III non-small cell lung cancer: randomized phase III trial RTOG 9410. J Natl Cancer Inst 2011;103:1452-60. [Crossref] [PubMed]
- Bradley JD, Paulus R, Komaki R, et al. Standard-dose versus high-dose conformal radiotherapy with concurrent and consolidation carboplatin plus paclitaxel with or without cetuximab for patients with stage IIIA or IIIB non-small-cell lung cancer (RTOG 0617): a randomised, two-by-two factorial phase 3 study. Lancet Oncol 2015;16:187-99. [Crossref] [PubMed]
- Fletcher GH. Textbook of radiotherapy. 3rd edition. Philadelphia: Lea and Febiger, 1980:195.
- Withers HR, Peters LJ, Taylor JM. Dose-response relationship for radiation therapy of subclinical disease. Int J Radiat Oncol Biol Phys 1995;31:353-9. [Crossref] [PubMed]
- Okunieff P, Morgan D, Niemierko A, et al. Radiation dose-response of human tumors. Int J Radiat Oncol Biol Phys 1995;32:1227-37. [Crossref] [PubMed]
- Withers HR, Suwinski R. Radiation dose response for subclinical metastases. Semin Radiat Oncol 1998;8:224-8. [Crossref] [PubMed]
- Cai S, Shi A, Yu R, et al. Feasibility of omitting clinical target volume for limited-disease small cell lung cancer treated with chemotherapy and intensity-modulated radiotherapy. Radiat Oncol 2014;9:17. [Crossref] [PubMed]
- Liang X, Yu H, Yu R, et al. Efficacy of the smaller target volume for stage III non-small cell lung cancer treated with intensity-modulated radiotherapy. Mol Clin Oncol 2015;3:1172-6. [Crossref] [PubMed]
- Kong FM, Ten Haken RK, Schipper MJ, et al. High-dose radiation improved local tumor control and overall survival in patients with inoperable/unresectable non-small-cell lung cancer: long-term results of a radiation dose escalation study. Int J Radiat Oncol Biol Phys 2005;63:324-33. [Crossref] [PubMed]
- Bradley JD, Moughan J, Graham MV, et al. A phase I/II radiation dose escalation study with concurrent chemotherapy for patients with inoperable stages I to III non-small-cell lung cancer: phase I results of RTOG 0117. Int J Radiat Oncol Biol Phys 2010;77:367-72. [Crossref] [PubMed]
- Bradley J, Graham MV, Winter K, et al. Toxicity and outcome results of RTOG 9311: a phase I-II dose-escalation study using three-dimensional conformal radiotherapy in patients with inoperable non-small-cell lung carcinoma. Int J Radiat Oncol Biol Phys 2005;61:318-28. [Crossref] [PubMed]
- Giraud P, Antoine M, Larrouy A, et al. Evaluation of microscopic tumor extension in non-small-cell lung cancer for three-dimensional conformal radiotherapy planning. Int J Radiat Oncol Biol Phys 2000;48:1015-24. [Crossref] [PubMed]
- Yuan S, Meng X, Yu J, et al. Determining optimal clinical target volume margins on the basis of microscopic extracapsular extension of metastatic nodes in patients with non-small-cell lung cancer. Int J Radiat Oncol Biol Phys 2007;67:727-34. [Crossref] [PubMed]
- Zhao L, Chen M, Ten Haken R, et al. Three-dimensional conformal radiation may deliver considerable dose of incidental nodal irradiation in patients with early stage node-negative non-small cell lung cancer when the tumor is large and centrally located. Radiother Oncol 2007;82:153-9. [Crossref] [PubMed]
- Kilburn JM, Lucas JT, Soike MH, et al. Is a Clinical Target Volume (CTV) Necessary in the Treatment of Lung Cancer in the Modern Era Combining 4-D Imaging and Image-guided Radiotherapy (IGRT)? Cureus 2016;8:e466. [PubMed]
- Ji K, Zhao LJ, Liu WS, et al. Simultaneous integrated boost intensity-modulated radiotherapy for treatment of locally advanced non-small-cell lung cancer: a retrospective clinical study. Br J Radiol 2014;87:20130562. [Crossref] [PubMed]
- van der Weide L, van Sörnsen de Koste JR, Lagerwaard FJ, et al. Analysis of carina position as surrogate marker for delivering phase-gated radiotherapy. Int J Radiat Oncol Biol Phys 2008;71:1111-7. [Crossref] [PubMed]
- Cerviño LI, Chao AK, Sandhu A, et al. The diaphragm as an anatomic surrogate for lung tumor motion. Phys Med Biol 2009;54:3529-41. [Crossref] [PubMed]