Hemorrhage under veno-venous extracorporeal membrane oxygenation in acute respiratory distress syndrome patients: a retrospective data analysis
Introduction
Extracorporeal membrane oxygenation (ECMO) is increasingly used in patients suffering from acute respiratory distress syndrome (ARDS) as rescue therapy to avoid life-threatening hypoxemia and to allow lung protective mechanical ventilation (MV) to minimize ventilator induced lung injury, while preventing hypercapnia and respiratory acidosis (1).
Due to the large nonbiologic surface, an uncontrolled activation of thrombotic and inflammatory response occurs. Hypercoagulation is counterbalanced by fibrinolytic response, resulting in clotting factor deficiencies, platelet dysfunction, thrombocytopenia, and fibrinolysis, with a higher risk of thrombosis or hemorrhage (2). Neurologic complications including intracranial hemorrhage are particularly frequent and are associated with increased mortality (3). New advances in technology such as development of heparin-coated ECMO circuits and cannulas as well as biocompatible membranes have decreased the demand for blood products and improved patient outcomes (4,5). However, hemorrhage complications are still frequent in patients on ECMO and are directly attributable to death in up to 17% of them (6-8). Introduction of membranes coated with covalent binding of heparin resulted in a decrease in inflammatory response, improved coagulation and thereby reduced rates of hemorrhage (9-11). Despite heparin coated ECMO circuits, manufacturers suggest providing additional therapeutic anticoagulation (AC), e.g., with heparin targeting an activated partial thromboplastin time (aPTT) of 1.5–2.5 times baseline (about 50–70 seconds) (12). ECMO therapy with prolonged PTT has been shown to be associated with adverse hemorrhage events such as intracranial hemorrhage (13). Our hypothesis is that addition to aPTT, there are other coagulation parameters indicative of risk for adverse hemorrhage effects under veno-venous (VV)-ECMO therapy. To test this hypothesis, we performed a retrospective analysis of patients under ECMO who received routine coagulation profiles regularly.
Methods
This retrospective study was approved by the institutional review board of the Medical Faculty of the University of Bonn and since only routine data was analyzed (Ethical-committee University of Bonn, Medical Faculty No. 295/17), the need for informed consent was waived. We examined data from 36 consecutive patients treated with VV-ECMO due to severe respiratory failure from November 2006 to February 2011. Patients were excluded if they were under the age of 18 years, receiving a veno-arterial ECMO support, or required a target aPTT higher than 40 seconds, due to other medical reasons. VV-ECMO was initiated in all patients as a rescue therapy to treat hypoxemia under conventional MV. Eighteen patients were cannulated in our intensive care unit (ICU). Eighteen additional patients were initially treated at other hospitals before cannulation on site by our institution’s ECMO-retrieval team, and transported to our ICU under VV-ECMO support.
VV-ECMO was initiated using either two or three cannulas, usually by cannulating bilateral femoral veins (17–24 Ch) and the right jugular vein for reperfusion (15–21 Ch). In the study period various ECMO circuits and pumps were used: Medtronic Bio-Pump (Minneapolis, MN, USA); Levitronix® CentriMag® (Waltham, Massachusetts, USA; Jostra-Maquet, Rastatt, Germany) and the Maquet Cardiohelp System (Maquet Holding B.V. & Co. KG, Rastatt, Germany). Both Cardiohelp and Centrimag used the Quadrox™ Oxygenator with Bioline™ coating. All systems were primed with crystalloids. The Bio-Pump was flushed with 5 IU/mL of heparin; the other systems were primed without heparin. The blood flow in the VV-ECMO circuit and the sweep gas oxygen-fraction (FiO2) were adjusted to achieve adequate oxygenation defined as an arterial partial pressure of oxygen (PaO2) >70 mmHg and an arterial saturation of oxygen (SaO2) >90%. FiO2 of the ventilator was set at the same FiO2 as the VV-ECMO sweep gas flow. All patients received time-cycled pressure controlled ventilation with ventilator rates of 4–10 min−1 and tidal volumes below 4 mL·kg−1/predicted body weight (PBW) while targeting normocapnia or mild hypercapnic acidosis. Ventilator settings were adjusted by an attending physician. Weaning from VV-ECMO support was performed by gradually decreasing blood flow to a minimum of 2.5 L/min as tolerated. Gas flow was stopped prior to discontinuation of VV-ECMO. If the patient remained stable blood flow was stopped and the patient was decannulated. VV-ECMO circuits where visually inspected daily for major thrombosis in the oxygenator or tubes.
After starting ECMO therapy a mild hypothermia with a target body temperature of 35 to 36 °C was performed to reduce the cerebral metabolic rate of oxygen and thus to achieve a possible neuroprotective effect (14), provided there were no contraindications such as bleedings. Patients received standard care, including vasopressor, fluid, antibiotic, nutrition, and sedation therapy. If hemodialysis was required, a separate catheter was used while AC targets remained unchanged.
Unfractioned heparin (UFH) was continuously administered intravenously to achieve an aPTT of 35–40 seconds. Laboratory testing for coagulation variables was performed every 8 hours according to standard ICU care or if heparin dosage was adjusted or special circumstances occurred leading to more frequent control. Factors II, V, VII, VIII and X were tested for less frequently and irregularly, depending on the retrospective character of the patients’ file.
If aPTT was out of the target, infusion rate of UFH was changed as needed. All laboratory tests were performed at the clinical laboratory of the University Hospital on several automated analyzers. Prothrombin time (PT), thrombin time, reptilase, fibrinogen factors II, V, VII, VIII, and X were measured using coagulatoric-mechanical endpoint measurement (AMAX CS 190, Amelung) and fixed-absorbance method (BCS XP, Siemens Healthcare Diagnostik). APTT was measured using drifting baseline (ACL TOP, Fa. IL and BCS XP Geräten, Siemens Healthcare Diagnostik). Antithrombine (AT III) was measured chromogenic with substrat turnover. Plasminogen was measured using chromogenic tests (Photometric, kinetic Absorptionsspectometry), (BCS XP Geräten, Siemens Healtcare Diagnostik). D-Dimers fibrin degradation products (D-Dimers-FDP) were measured using enzyme immunoassay (Vidas BioMérieux) and using latex enzyme immunoassay method (LEIA) (BCS XP, Siemens Healthcare). ROTEG® parameters (ROTEG-R, ROTEG-K, ROTEG-ME) were measured by an opto-mechanic detection method (Model ROTEG® 04 und 05, Fa. Pentapharm). Erythrocytes, hemoglobin, hematocrit, leukocytes, and platelets were measured using impedance measurement, sodiumaurylsulfat-hemoglobinmethod, cumulative impulslevelsummation, fluorescenc-flowcytomtry (Sysmex XT 2000i, Fa. Sysmex).
Transfusion of packed red blood cells (RBC), fresh frozen plasma (FFP), and platelets was at the discretion of the attending physicians based on current national and international recommendations and guidelines (15-18). Usually the targeted hemoglobin level was above 8–9 g/dL.
Data analysis
The following data were collected: demographics, hemodynamic data, coagulation samples, transfusion requirements, change of VV-ECMO circuit during treatment, and adverse effects like hemorrhage or thromboembolic events. Laboratory data, and Simplified Acute Physiology Score (SAPS II) (19) were collected from the electronic medical records (EMR) (Orbis®, KAS, Agfa-Gevaert N.V., Belgium) as part of routine clinical data. Glasgow Coma Scale was not included in calculation of SAPS II. Hemodynamic-, VV-ECMO- and ventilator parameters were collected from routine documentation in the ICU charts. Only 4 of the 36 patients remained in the ICU on VV-ECMO for longer than 12 days. We therefore present the laboratory data for the first 11 days and did not include laboratory data for analysis after day 11.
Hemorrhage- and thrombotic/ischemic side effects were extracted from the patient files. Hemorrhage from mouth, nose or pharynx region, or in combination from these regions, either diffuse or local were combined as hemorrhage from nose-/oropharynx region. Hemorrhage effects from puncture sites of central venous lines, VV-ECMO cannulas, chest tubes or any other drain were combined as hemorrhage from puncture sites. Hemorrhage from tracheostomy site was counted as own hemorrhage complication, as well as hemorrhage complications from a gastric tube. Hemorrhage from the indwelling urinary catheter was defined as hematuria. Hemorrhage from the lungs was defined if either hemorrhage was found during bronchoscopy or endotracheal suction maneuvers. Intracranial hemorrhage or intracranial infarction were defined as a cranial computer tomography showing signs of hemorrhage or infarction. Hematomas were separated as occurring hematomas either spontaneously or after an intervention like puncturing of a vessel or removing a catheter/drain.
Thrombotic/ischemic complications were defined as thrombosis if either a macroscopic thrombus formation was found or a radiologic examination like ultrasound or CT scan showed signs for thrombotic formation or ischemia.
Severity of hemorrhagic complications was defined as follows: any intracranial or intratumoral hemorrhage, and any intraabdominal hemorrhage needing more intervention than inserting a drain were counted as severe hemorrhage. The same criteria were used for any hemorrhage complication lasting longer than 4 days in a row with a transfusion requirement of more than two packed RBC per day or a single transfusion requirement of six packed RBCs or higher. If time on VV-ECMO was less than 6 days the parameter criteria for severe hemorrhage were defined as a hemorrhage time of 50% of time on VV-ECMO. Any other hemorrhage event was defined as mild. Only hemorrhage events occurring under VV-ECMO treatment were used for separation into groups. Adverse events occurring pre- or post VV-ECMO-treatment were not used for separation into groups, e.g., hemorrhage from lungs into group severe hemorrhage.
Statistical analysis
After separation into severe-hemorrhage-group and mild-hemorrhage-group analysis of data was performed. Data are presented either as means ± standard (SD) error of the mean (SEM) or as median and 25%/75% interquartile range. Demographic data (Table 1) were checked for normality using the Shapiro-Wilk W test. If values were normally distributed, they are presented as mean ± SD and an unpaired t-test was performed. If values were not normally distributed data are presented as median (25/75 percentile) and a Mann-Whitney test was performed. Gender, inter-hospital transfer, survival on ICU and ECMO were analyzed using a Chi-Square test.
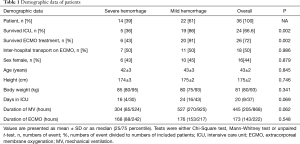
Full table
Because the count of values at each day for coagulation/laboratory values was usually less than or equal to six, the median and percentile were used instead of standard deviation. Laboratory data were tested for normal distribution by using skewness and kurtosis. If necessary parameter were log-transformed and checked again. These numbers were compared using univariate split-approach of analysis of variance with repeated measures. Differences were considered to be statistically significant if P<0.05.
GraphPad Prism® Version 5.02, GraphPad Software, Inc., USA, JMP® 11.0.0, SAS Institute Inc. and Microsoft® Office Excel® 2007, Microsoft Corporation were used for statistical analysis.
Results
Thirty-six consecutively treated patients with VV-ECMO due to underlying respiratory failure were included into this analysis. Demographic data are presented in Table 1; 14 patients were in the severe hemorrhage group and 22 patients were in the mild hemorrhage group. In the severe hemorrhage group survival rate on VV-ECMO (43% vs. 91%; P=0.002) and in the ICU (36% vs. 86%; P=0.002) was significantly lower compared to mild hemorrhage group. There was a group effect on SAPS II, that was significantly higher in severe hemorrhage group (P=0.0194). The most common reason for initiation of VV-ECMO therapy was pneumonia. Influenza-A-virus H1N1 infections were observed in nine cases.
Hemorrhage complications
We observed several hemorrhagic complications (Table 2). Bleeding from the lungs occurred in eight patients, while intracranial bleeding occurred in four patients. Transfusion requirements are presented in Table 3, showing a difference between groups in transfused RBC per 24 hours, RBC per patient and transfused platelets per 24 hours. Correlation analysis of transfused packages of RBC to maximum D-Dimers-FDP per day using Spearman test for nonparametric correlation resulted in r2 =0.014 with a confidence interval −0.002 to 0.23.
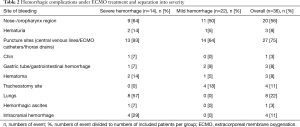
Full table
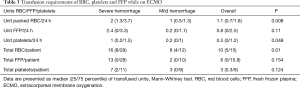
Full table
Coagulation laboratory values, temperature, transfusion requirements, heparin dosage and SAPS II are presented in Figures 1-5.
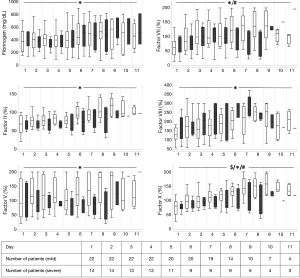
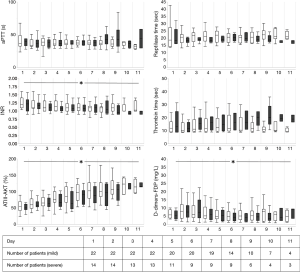
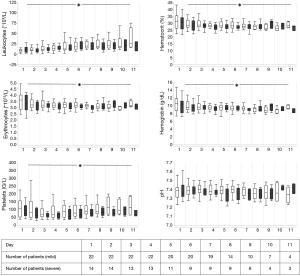
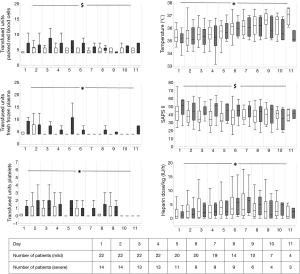
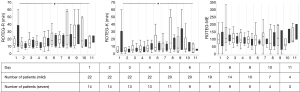
Thrombotic/ischemic complications
No thrombosis or clotting resulting in dysfunction or reduced effectiveness within an VV-ECMO-circuit was observed. Five events (14%) occurred that we rated as thrombotic/ischemic complications based on our definitions in our patients following VV-ECMO treatment.
Coagulation related therapy
Nine Patients required specific coagulation therapy during VV-ECMO. Two patients received prothrombin complex, two AT III, one factor XIII, five tranexamic acid, and one fibrinogen.
Discussion
In this retrospective VV-ECMO study, we experienced several hemorrhagic complications despite a relatively low AC target. Patients with severe hemorrhage had a relevantly higher mortality (57% vs. 9%) on ECMO. Frequent monitoring of coagulation parameters revealed differences between severe and mild hemorrhage groups in factors VII, X and SAPS II.
In our opinion the great advantage of our study is, that we analyzed multiple coagulation- and AC parameters frequently. Despite this extensive AC diagnostics, however, we could not determine any other valid parameter that might be used for prediction of onset of hemorrhagic complications during ECMO treatment. During ECMO therapy physicians have to balance the aim of avoiding clotting of the ECMO circuit with the risk of bleeding due to AC and ECMO associated coagulopathy.
A large surface area of an extracorporeal oxygenation membrane activates the coagulation cascade necessitating AC even when using heparin coated systems to deliver ECMO therapy safely (12). Thrombo-embolization as well as thrombus formation around the outer surface of the cannulas at the time of removing the cannulas, can contribute to morbidity and mortality (20). On the other hand, AC therapy itself can cause hemorrhage associated with increased requirement for blood transfusions (6-8) or fatal outcomes, e.g., due to intracranial or intrapulmonary hemorrhage. Minimizing such side effects should be advantageous for safety during ECMO therapy. Close monitoring of the coagulation status to reduce the need for AC may be an option. Hemostatic changes under ECMO therapy seem to be independent of the deployed ECMO system (21).
Monitoring of AC therapy under ECMO is recommended and the most common parameter used to assess adequacy of AC therapy in ECMO is activated clotting time (ACT) with a goal range between 180–200 seconds (22) or aPTT with a target of 1.5–2.5 of baseline (12). ECMO-AC management policies still vary widely, as shown in a recent survey from 121 ECMO centers, concluding that further research is necessary to find the optimal AC management under ECMO-therapy (22).
In line with previous studies (23,24), ECMO was applied even in early post-traumatic or surgical patients and a relatively low aPTT of 35–40 was targeted. Lower and or delayed AC was safely used in experimental studies or in trauma patients (24,25). In one reported series of ECMO cases authors did not use any AC for a short time (26), although in one of these patients’ multiple thrombus formations were detected in the inferior cava vein. We believed that AC is advantageous for safe ECMO delivery albeit the AC target may be decreased. In this study seconds we did not see clinical relevant thrombus formation during ECMO therapy with low levels of AC, although we did not examine thrombus formation at the cannulas prior to explanation, nor did we do routine CT-scans/ultrasound examination post ECMO-therapy. Out of 36 patients, only in one patient (3%) a thrombus was shown, after discontinuing ECMO therapy, in a vessel that was not cannulated for the delivery of ECMO, but for a central venous line.
Despite a low target aPTT of 35–40 seconds, the number of hemorrhage complications was still unexpectedly high, with some fatal cases. When separating our patients into two groups, patients with severe hemorrhagic complications had a significantly higher mortality than patients with mild hemorrhagic complications (57% vs. 9%). Especially intrapulmonary hemorrhage resulted in higher mortality (6/8; 75%). In line with previous reports, we observed a rate of 11% of intracranial hemorrhage during ECMO (3,13). A higher aPTT seems to be an independent risk factor for higher need of transfusion (27) and hemorrhage under ECMO (28). Because our target-aPTT was lower compared to Aubron et al., this effect maybe was not obvious in our patients. Usage of our AC-protocol resulted in similar aPTT and a similar heparin dosage per kg body weight as presented in Figure 2 between the groups. We can therefore exclude an effect of heparin on the bleeding results.
Our data show that factor VII and X may be indicators for a higher probability of hemorrhage under VV-ECMO. It appears that these factors are on a supranormal level in the low bleeding group. The rational for this remains unclear. Maybe the higher bleeding resulted in an extended loss of these two factors. However, we did not measure factor XIII nor did we measure platelet function using a method other than ROTEG®. Hemorrhage during ECMO can be caused by an acquired von Willebrand syndrome with loss of large multimers of the von Willebrand factor, even within 24 hours after ECMO implantation (29), resulting in a less effective platelet binding (30). Shear stress induced to thrombocytes seem to uncoil multimers of von Willebrand factor and then activate platelets (31). Thermoelectrometry measured by ROTEG® did not differ between the two groups (Figure 5) and thus cannot serve as predictor for hemorrhage under VV-ECMO. Previous investigations show conflicting results if thermoelectrometry can be used as predictor for hemorrhage under ECMO (32,33). Furthermore, measurement of thermoelectrometry was different in these studies which also may make comparison difficult (34).
Compared to a study by Ang et al. (35), transfusion requirements were similar while daily and overall platelet transfusions were lower in our patients. While threshold for transfusion of platelets in the study of Ang et al. was 100×109/L, transfusion of platelets in our study was at the discretion of the attending physician based on current national recommendations (50–100 G/L) (15) and international results (18).
With the exception of packed RBC, factor VII, factor X and SAPS II we did not see any significantly different parameters between the two groups. The difference in packed RBC is understandable, since packed RBC is a parameter that was used for group separation. Both factor VII and X were lower in the severe hemorrhage group. Factor VII and X have a major function in the coagulation cascade, while activated factor VII activates factor X to reach the common coagulation pathway (2). Imbalance of these factors may have a high impact on coagulation and hemorrhage. In fact, there is evidence that substitution of recombinant activated factor VII controlled intractable hemorrhage in patients under ECMO (36). Anti-factor Xa monitoring is one way to control AC using heparin (37), highlighting the important impact of factor X in coagulation.
It was part of our clinical concept to avoid fever and use even mild hypothermia for neuroprotection (38,39) after initiation of ECMO therapy. Our temperature target of 35–36 °C during initial therapy was higher compared to studies using therapeutic hypothermia (14,38,39). Previously, a higher rate of hemorrhage was attributed to even mild hypothermia (40). Also, it influences thromboxane B2 and the platelets (41) and influences coagulation cascade (42). We could not detect a difference of body temperature between the two groups in our study (Figure 4).
Limitations
First of all, this is a retrospective, observational study design which exposes it to the patient and treatment selection bias. Additionally, the study was limited to a single center and a relatively small, number of patients (n=36), which may have skewed the results. A bias can result from the fact, that we established ECMO in different kind of diseases, e.g., trauma patients and ARDS due to sepsis. The different origins of disease may need for statistical reasons a much higher number of patients, which is in these special patients not without problems. It cannot be excluded that different diseases causing ARDS may influence coagulation and hemorrhage differently. Furthermore, we did not routinely screen for thrombus formation prior and post decannulation, while we did not experience clinical relevant thrombus formation. The cause of ARDS in our patients was heterogeneous. The study period includes a longer period and a change of ECMO circuits, which may have influence on our results. Our results are limited to VV-ECMO and we cannot transfer our results to veno-arterial ECMO. Finally, by using our statistical method we generated a drop-out bias for laboratory parameters after day 11.
Conclusions
Severe hemorrhage under VV-ECMO is associated in this retrospective examination with higher mortality. Despite extensive and regular testing, we could not find a difference in groups other than factor VII and X and SAPS II during VV-ECMO therapy. Further clinical studies to determine the timing of initiation and targets for AC therapies during VV-ECMO and to find predictors of hemorrhage at the beginning of ECMO-treatment are necessary.
Acknowledgements
This work was supported by an unrestricted grant from Levitronix® Waltham, Massachusetts, USA. This study was performed at the Department of Anesthesiology and Intensive Care Medicine, University of Bonn, Sigmund-Freud-Str. 25, 53125 Bonn, Germany.
We gratefully thank Dr. Vittorio Scaravilli (Ospedale Maggiore Policlinico-Fondazione IRCCS Cà Granda, Milan, Italy) for giving input in anticoagulation therapy under ECMO; we gratefully thank William Baker (U.S. Army Institute of Surgical Research, JBSA Fort Sam Houston, Texas) for proofreading and optimizing language in this paper.
Footnote
Conflicts of Interest: Part of these data has been presented in form of abstracts at the American Thoracic Society international conference 2010 (New Orleans) and at the European Society of Intensive Care Medicine annual congress 2010 (Barcelona).
Ethical Statement: This retrospective study was approved by the institutional review board of the Medical Faculty of the University of Bonn and since only routine data was analyzed (Ethical-committee University of Bonn, Medical Faculty No. 295/17) and the need for informed consent was waived.
References
- Ricard JD, Dreyfuss D, Saumon G. Ventilator-induced lung injury. Eur Respir J Suppl 2003;42:2s-9s. [Crossref] [PubMed]
- Oliver WC. Anticoagulation and coagulation management for ECMO. Semin Cardiothorac Vasc Anesth 2009;13:154-75. [Crossref] [PubMed]
- Luyt CE, Bréchot N, Demondion P, et al. Brain injury during venovenous extracorporeal membrane oxygenation. Intensive Care Med 2016;42:897-907. [Crossref] [PubMed]
- Peek GJ, Mugford M, Tiruvoipati R, et al. Efficacy and economic assessment of conventional ventilatory support versus extracorporeal membrane oxygenation for severe adult respiratory failure (CESAR): a multicentre randomised controlled trial. Lancet 2009;374:1351-63. [Crossref] [PubMed]
- Patroniti N, Zangrillo A, Pappalardo F, et al. The Italian ECMO network experience during the 2009 influenza A(H1N1) pandemic: preparation for severe respiratory emergency outbreaks. Intensive Care Med 2011;37:1447-57. [Crossref] [PubMed]
- Brunet F, Belghith M, Mira JP, et al. Extracorporeal carbon dioxide removal and low-frequency positive-pressure ventilation. Improvement in arterial oxygenation with reduction of risk of pulmonary barotrauma in patients with adult respiratory distress syndrome. Chest 1993;104:889-98. [Crossref] [PubMed]
- Kolla S, Awad SS, Rich PB, et al. Extracorporeal life support for 100 adult patients with severe respiratory failure. Ann Surg 1997;226:544-64; discussion 565-6. [Crossref] [PubMed]
- Conrad SA, Rycus PT, Dalton H. Extracorporeal Life Support Registry Report 2004. ASAIO J 2005;51:4-10. [Crossref] [PubMed]
- Peek GJ, Firmin RK. The inflammatory and coagulative response to prolonged extracorporeal membrane oxygenation. ASAIO J 1999;45:250-63. [Crossref] [PubMed]
- Palatianos GM, Foroulis CN, Vassili MI, et al. A prospective, double-blind study on the efficacy of the bioline surface-heparinized extracorporeal perfusion circuit. Ann Thorac Surg 2003;76:129-35. [Crossref] [PubMed]
- Mahmood S, Bilal H, Zaman M, et al. Is a fully heparin-bonded cardiopulmonary bypass circuit superior to a standard cardiopulmonary bypass circuit? Interact Cardiovasc Thorac Surg 2012;14:406-14. [Crossref] [PubMed]
- Annich G, Lynch W, Maclaren G, et al. ECMO Extracorporeal Cardiopulmonary Support in Critical Care. 4th ed. Ann Arbor Michigan: Extraorporeal Life Support Organization, 2012:537.
- Kasirajan V, Smedira NG, McCarthy JF, et al. Risk factors for intracranial hemorrhage in adults on extracorporeal membrane oxygenation. Eur J Cardiothorac Surg 1999;15:508-14. [Crossref] [PubMed]
- Guenther U, Varelmann D, Putensen C, et al. Extended therapeutic hypothermia for several days during extracorporeal membrane-oxygenation after drowning and cardiac arrest Two cases of survival with no neurological sequelae. Resuscitation 2009;80:379-81. [Crossref] [PubMed]
- Vorstand und Wissenschaftlichem Beirat der Bundesärztekammer. Guidelines for therapy with blood components and plasmaderivates. 3rd ed. Köln: Deutscher Ärzte-Verlag, 2003.
- Hébert PC, Wells G, Blajchman MA, et al. A multicenter, randomized, controlled clinical trial of transfusion requirements in critical care. Transfusion Requirements in Critical Care Investigators, Canadian Critical Care Trials Group. N Engl J Med 1999;340:409-17. [Crossref] [PubMed]
- Stainsby D, MacLennan S, Hamilton PJ. Management of massive blood loss: a template guideline. Br J Anaesth 2000;85:487-91. [Crossref] [PubMed]
- Samama CM, Djoudi R, Lecompte T, et al. Perioperative platelet transfusion: recommendations of the Agence Française de Sécurité Sanitaire des Produits de Santé (AFSSaPS) 2003. Can J Anaesth 2005;52:30-7. [Crossref] [PubMed]
- Le Gall JR, Lemeshow S, Saulnier F. A new Simplified Acute Physiology Score (SAPS II) based on a European/North American multicenter study. JAMA 1993;270:2957-63. [Crossref] [PubMed]
- Bouras G, Burns EM, Howell AM, et al. Risk of Post-Discharge Venous Thromboembolism and Associated Mortality in General Surgery: A Population-Based Cohort Study Using Linked Hospital and Primary Care Data in England. PLoS One 2015;10:e0145759. [Crossref] [PubMed]
- Malfertheiner MV, Philipp A, Lubnow M, et al. Hemostatic Changes During Extracorporeal Membrane Oxygenation: A Prospective Randomized Clinical Trial Comparing Three Different Extracorporeal Membrane Oxygenation Systems. Crit Care Med 2016;44:747-54. [PubMed]
- Bembea MM, Annich G, Rycus P, et al. Variability in anticoagulation management of patients on extracorporeal membrane oxygenation: an international survey. Pediatr Crit Care Med 2013;14:e77-84. [Crossref] [PubMed]
- Huang YK, Liu KS, Lu MS, et al. Extracorporeal life support in post-traumatic respiratory distress patients. Resuscitation 2009;80:535-9. [Crossref] [PubMed]
- Arlt M, Philipp A, Voelkel S, et al. Extracorporeal membrane oxygenation in severe trauma patients with bleeding shock. Resuscitation 2010;81:804-9. [Crossref] [PubMed]
- Prat NJ, Meyer AD, Langer T, et al. Low-Dose Heparin Anticoagulation During Extracorporeal Life Support for Acute Respiratory Distress Syndrome in Conscious Sheep. Shock 2015;44:560-8. [Crossref] [PubMed]
- Muellenbach RM, Kredel M, Kunze E, et al. Prolonged heparin-free extracorporeal membrane oxygenation in multiple injured acute respiratory distress syndrome patients with traumatic brain injury. J Trauma Acute Care Surg 2012;72:1444-7. [Crossref] [PubMed]
- Weingart C, Lubnow M, Philipp A, et al. Comparison of Coagulation Parameters, Anticoagulation, and Need for Transfusion in Patients on Interventional Lung Assist or Veno-Venous Extracorporeal Membrane Oxygenation. Artif Organs 2015;39:765-73. [Crossref] [PubMed]
- Aubron C, DePuydt J, Belon F, et al. Predictive factors of bleeding events in adults undergoing extracorporeal membrane oxygenation. Ann Intensive Care 2016;6:97. [Crossref] [PubMed]
- Kalbhenn J, Schmidt R, Nakamura L, et al. Early diagnosis of acquired von Willebrand Syndrome (AVWS) is elementary for clinical practice in patients treated with ECMO therapy. J Atheroscler Thromb 2015;22:265-71. [Crossref] [PubMed]
- Heilmann C, Geisen U, Beyersdorf F, et al. Acquired von Willebrand syndrome in patients with extracorporeal life support (ECLS). Intensive Care Med 2012;38:62-8. [Crossref] [PubMed]
- Andrews RK, Berndt MC. Platelet physiology and thrombosis. Thromb Res 2004;114:447-53. [Crossref] [PubMed]
- Panigada M, Iapichino G, L`Acqua C, et al. Prevalence of “Flat-Line” Thromboelastography During Extracorporeal Membrane Oxygenation for Respiratory Failure in Adults. ASAIO J 2016;62:302-9. [Crossref] [PubMed]
- Nair P, Hoechter DJ, Buscher H, et al. Prospective observational study of hemostatic alterations during adult extracorporeal membrane oxygenation (ECMO) using point-of-care thromboelastometry and platelet aggregometry. J Cardiothorac Vasc Anesth 2015;29:288-96. [Crossref] [PubMed]
- Sankarankutty A, Nascimento B, Teodoro da Luz L, Rizoli S. TEG® and ROTEM® in trauma: similar test but different results? World J Emerg Surg 2012;7:S3. [Crossref] [PubMed]
- Ang AL, Teo D, Lim CH, et al. Blood transfusion requirements and independent predictors of increased transfusion requirements among adult patients on extracorporeal membrane oxygenation -- a single centre experience. Vox Sang 2009;96:34-43. [Crossref] [PubMed]
- Repessé X, Au SM, Bréchot N, et al. Recombinant factor VIIa for uncontrollable bleeding in patients with extracorporeal membrane oxygenation: report on 15 cases and literature review. Crit Care 2013;17:R55. [Crossref] [PubMed]
- Hirsh J, Raschke R. Heparin and low-molecular-weight heparin: the Seventh ACCP Conference on Antithrombotic and Thrombolytic Therapy. Chest 2004;126:188S-203S. [Crossref] [PubMed]
- Bernard SA, Gray TW, Buist MD, et al. Treatment of comatose survivors of out-of-hospital cardiac arrest with induced hypothermia. N Engl J Med 2002;346:557-63. [Crossref] [PubMed]
- Hypothermia after Cardiac Arrest Study Group. Mild therapeutic hypothermia to improve the neurologic outcome after cardiac arrest. N Engl J Med 2002;346:549-56. [Crossref] [PubMed]
- Rajagopalan S, Mascha E, Na J, et al. The effects of mild perioperative hypothermia on blood loss and transfusion requirement. Anesthesiology 2008;108:71-7. [Crossref] [PubMed]
- Valeri CR, Feingold H, Cassidy G, et al. Hypothermia-induced reversible platelet dysfunction. Ann Surg 1987;205:175-81. [Crossref] [PubMed]
- Rohrer MJ, Natale AM. Effect of hypothermia on the coagulation cascade. Crit Care Med 1992;20:1402-5. [Crossref] [PubMed]