Sleep disordered breathing in motor neurone disease
Motor Neurone Disease (MND) is a neurodegenerative disease characterised by axonal loss and gliosis of upper and lower motor neurones in the motor cortex, lower brainstem nuclei and ventral horn of the spinal cord. MND is currently incurable and has a poor prognosis, with death typically occurring 3 to 5 years after disease onset (1,2). It is a relatively uncommon disease estimated to affect 4.7 per 100,000 people (3) although is one of the most common adult neurodegenerative diseases (4). MND is characterised by rapidly progressive weakness leading to paralysis, fasciculations, bulbar symptoms (including dysarthria and dysphagia) and respiratory compromise.
Respiratory complications arise as a result of weakness of upper airway (pharyngeal and laryngeal) muscles and respiratory muscles (diaphragm, intercostal and accessory muscles) leading to respiratory failure. Acute respiratory failure, often precipitated by infective or aspiration pneumonia or impacted secretions, is the leading cause of death in patients with MND (5,6). Management of respiratory failure with non-invasive ventilation (NIV) improves both quality of life and survival (4,7).
Sleep disordered breathing (SDB) is an umbrella term which includes various separate disorders in which abnormal respiratory events occur during sleep, which are associated with adverse clinical outcomes. Due to early involvement of respiratory muscles in MND, SDB occurs at a higher frequency than compared to the general population (8). It significantly impacts upon patients’ quality of life (9) and survival (4) and its presence may predict prognosis. SDB usually precedes daytime respiratory symptoms and chronic respiratory failure (10). Managing SDB in MND with NIV improves quality of life and survival. Early identification and management of SDB in patients with MND is therefore crucial (11).
Due to the rapid disease progression that occurs amongst patients with this uncommon condition, large studies investigating SDB in patients with MND are difficult. This update will review investigation of respiratory muscle weakness in MND, physiological changes that occur during sleep in healthy subjects and how this differs in MND, types of SDB that MND patients experience and considerations on why they occur, and the effects of NIV on SDB in MND.
Respiratory muscle involvement in MND
Respiratory muscle weakness, particularly diaphragm weakness and ultimately paralysis, is common in MND and directly contributes to the development of SDB and respiratory failure (12). Diaphragm dysfunction is also associated with reduced survival in MND, with one cohort demonstrating a reduction in median survival of 402 days (median survival of 217 days for MND patients with evidence of diaphragm dysfunction compared to 619 days for those with no evidence of diaphragm dysfunction) (12). Inspiratory intercostal muscle weakness contributes to dyspnoea and nocturnal hypoventilation and weakness of expiratory muscles, in combination with swallowing difficulties, leads to ineffectual cough and impaired secretion clearance, thereby increasing risk of aspiration (13). Tests of respiratory muscle function should be performed regularly in MND patients to facilitate early identification and management of SDB and chronic respiratory failure (11).
Clinical examination may reveal paradoxical inward abdominal movement during inspiration (14), “respiratory pulse” during inspiration [where there is visible contraction of scalene muscles (15)] and/or rapid, shallow breathing. This breathing pattern reduces inspiratory time and trans-diaphragmatic pressure which limits alveolar ventilation, thereby contributing to the development of chronic respiratory failure (16).
A variety of tests can be performed to assess respiratory muscle function. Vital capacity (VC) tested in the sitting and supine positions, which can fall by up to 19% in healthy subjects (17), can fall precipitously in MND. A fall more than 30% is pathognomonic for diaphragmatic paralysis (18). Recent evidence demonstrates that, although VC falls throughout the disease course of MND, the cut-off at which it is predictive of a poor prognosis beyond 3 months from baseline assessment still falls within the normal range (>80% predicted). Its clinical utility as a risk stratification tool is therefore limited (19).
Measurement of maximal inspiratory pressure (MIP) is a useful non-invasive measurement of global inspiratory muscle strength and is closely related to diaphragmatic strength. Recent studies have demonstrated that higher MIP values are associated with increased survival in patients with neuromuscular disease (13) and are highly sensitive for predicting 36-month ventilation-free and absolute survival in MND patients (19,20). Measurements may be technically difficult to obtain and produce unreliable recordings in patients with advanced disease or bulbar involvement due to difficulty forming a tight seal around the mouthpiece, upper airways collapse and dyspraxia of respiratory and upper airways muscles (21). Sniff nasal inspiratory pressure (SNIP) in evaluating inspiratory muscle strength in MND has been shown to be a valuable biomarker of respiratory muscle strength (19). It is performed reliably, even in advanced and bulbar disease, and may be easier to perform than VC since measurements do not require the use of a mouthpiece (22). In MND patients, SNIP is sensitive to early deterioration in respiratory muscle weakness (22), there is a linear correlation between SNIP and nocturnal desaturation (7), lower values are associated with hypercapnia in MND (21) and, when compared to Forced VC (FVC), SNIP is a better predictor of mortality or need for tracheostomy in 1 year (23). It has recently been demonstrated to be a highly sensitive and specific biomarker of respiratory muscle strength in MND and is predictive of 36-month ventilation-free and absolute survival (19).
Hemi-diaphragm weakness may be identified using radiological methods such as fluoroscopic sniff testing and thoracic ultrasonography. These investigations may reveal raised hemi-diaphragms and paradoxical upward movement of the diaphragm during inspiration. However, these methods cannot determine severity of the weakness and are limited if there is no normal hemi-diaphragm to compare to (24).
Although of limited use in routine clinical practice, the most accurate assessment of diaphragm function is diaphragm electromyography (EMG) and measurement of voluntary and twitch (following magnetic or electrical stimulation) trans-diaphragmatic pressures (Tw Pdi). Tw Pdi can be considered the gold standard for predicting mortality and NIV since it is independent of patient motivation and investigator skills (19). Diaphragm EMG responses to cervical or cortical magnetic stimulation and phrenic nerve conduction studies can also be used (12,25).
In summary, tests of respiratory muscle function permit early identification of respiratory muscle weakness, which is associated with SDB and respiratory failure, and may predict survival. Early recognition and initiation of treatment with NIV improves quality of life and survival in MND patients. Regular objective assessment of respiratory muscle function should therefore accompany clinical assessment.
Changes that occur during sleep in healthy subjects and in MND
The normal physiology of sleep offers a stress to the load-capacity-drive relationship of the respiratory system which can be accentuated in neuromuscular disease. In the healthy population during sleep, tidal volume and respiratory rate decrease (26) and activity of pharyngeal dilator muscles reduce (27). During the rapid eye movement (REM) stage of sleep, there is almost complete loss of tone of the intercostal muscles, however, the activity of the diaphragm is relatively preserved (28). The diaphragm is thus crucial in maintaining ventilation during sleep, particularly during periods of REM. During both non-REM and REM sleep, chemosensitivity is altered resulting in impaired ventilator responses to hypercapnia and hypoxia (29). These changes are more profound during REM sleep, falling to less than one third of the sensitivity at wakefulness (30). As a result of these changes in muscle activity during sleep, there is a mean maximal increase in PaCO2 by 6 mmHg in healthy subjects (31). These normal physiological changes contribute to SDB in MND.
In MND, loss of motor neurones leads to diaphragmatic, intercostal, accessory and upper airway dilator muscle weakness. These features adversely impact upon respiratory function. Respiratory dysfunction during sleep precedes daytime features of chronic respiratory failure since ventilation is more vulnerable in the sleeping state (32).
SDB in MND
SDB (SDB) has been reported in 17–76% of patients with MND (33). Respiratory muscle weakness, particularly diaphragm weakness, is the predominant mechanism for SDB in MND. SDB occurs early in the course of MND and precedes daytime symptoms and chronic respiratory failure (10). At the time of diagnosis of SDB, MND patients often have normal respiratory muscle strength (7,9,34), however, the severity of SDB is usually not significant until there is phrenic nerve involvement with diaphragm paralysis (8,12).
Clinical features
The daytime symptoms of SDB that patients experience are those relating to nocturnal hypoventilation and sleep fragmentation. MND patients commonly report difficulty initiating and maintaining sleep, dyspnoea and orthopnoea and experience unrefreshing sleep, daytime somnolence and fatigue, difficulty concentrating, poor memory and morning headaches (8,33). SDB is also associated with significantly reduced quality of life scores amongst MND patients (4). However, symptom load can be variable. A study from Arnulf and colleagues identified that the Epworth Sleepiness Scale was normal in the entire cohort of 24 MND patients and the scores were similar between the group with evidence of diaphragm dysfunction and the group without (12).
Altered sleep architecture
Patients with MND demonstrate altered sleep architecture on overnight polysomnography (PSG). When compared with healthy controls, MND patients have reduced total sleep time and reduced sleep efficiency, increased time spent awake after sleep onset, increased duration of stage 1 sleep and more frequent arousals and stage changes per hour (8,35). Amongst MND patients, those with evidence of diaphragm dysfunction have a higher frequency of awakening and a significant reduction in duration of REM sleep, with some patients having minimal or no REM sleep observed (12,36). Survival is shorter with shorter duration of REM sleep (12). Absence of REM sleep is uncommon and not usually seen in other neuromuscular diseases. It may occur in MND a result of involvement of both upper and lower motor neurones (12) and as a potentially protective mechanism against nocturnal hypoventilation.
Preservation of respiratory muscle tone during REM sleep
Studies have demonstrated that, amongst MND patients with reduced REM sleep undergoing overnight PSG, there is preservation of phasic inspiratory sternocleidomastoid and genioglossus tone during REM sleep, with no such preservation of tone in non-respiratory muscles such as tibialis anterior and levator menti (12,37). It has been postulated that MND patients die from respiratory failure when disease progression results in the inability to maintain tone of accessory muscles of respiration during REM sleep (12).
Sleep studies of patients with diaphragm dysfunction, not specifically caused by MND, also demonstrate activation of accessory muscles of respiration throughout all stages of REM sleep (38,39). Amongst these patients, neural respiratory drive to the diaphragm, measured using trans-oesophageal electromyography, is significantly increased during non-REM and REM sleep compared to healthy controls, in whom neural respiratory drive remains the same throughout all sleep stages (39). This raised neural respiratory drive to the diaphragm is considered to be a compensatory mechanism to preserve REM sleep and protect against nocturnal hypoventilation and obstructive sleep apnoea (OSA) during REM sleep in patients with diaphragm dysfunction (38,39). These finding may apply to patients with MND who have evidence of diaphragm dysfunction.
Nocturnal hypoventilation
Nocturnal hypoventilation is frequently reported in sleep studies of MND patients (8,40,41) and is likely a consequence of diaphragm, intercostal and accessory neck muscle weakness which is exacerbated in REM sleep (8,20). Overnight PSG performed on MND patients commonly highlights periods of non-obstructive hypopnoeas and central apnoeas which cause hypoventilation (8,42). Nocturnal hypercapnia has been shown to occur in 60% of one cohort of MND patients undergoing sleep studies. In 39.3% of patients in this cohort, the transcutaneous carbon dioxide tension (tcCO2) rose by more than 10 mmHg above baseline. Hypercapnia was observed to be more prominent or occur exclusively during REM sleep (35) (Figure 1). The apnoea-hypopnoea index (AHI) in MND patients is higher compared to healthy controls (8,42,43) and is significantly higher during REM sleep with associated significant falls in arterial oxygen saturation (8). The AHI has been shown to decrease with disease duration. Patients with a diagnosis of MND for less than 1 year have been shown to have a mean AHI of 23.4±14.6 events/hour (mean ± standard deviation), falling to 18.1±13.9 events/hour in those diagnosed with MND for 1–2 years, falling further to 15.8±15.2 events/hour in those diagnosed with MND for more than 2 years (43).
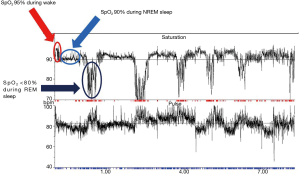
OSA
OSA is reported variably in patients with MND. It is reasonable to hypothesise that MND patients with bulbar muscle weakness are predisposed to OSA during REM sleep due to reduced tone of upper airway dilator muscles, however, this is not consistently observed. Several studies describe sleep apnoea as the most common form of SDB amongst their cohort of MND patients (20,35), with obstructive or mixed central/obstructive apnoeas being the predominant forms (10,20,35). In these studies, although obstructive apnoeas were not associated with nocturnal hypoventilation (20,44) a higher obstructive AHI was associated with shorter time to death or tracheostomy (44). In contrast, OSA in other MND cohorts is reported as rare in both non-REM and REM sleep, with other forms of SDB being common and significant (8,12,42,43). These discrepancies are likely to relate to the timing of the sleep study in the course of the disease as well as differing distribution of weakness.
There is also variation in the reported significance of bulbar dysfunction in the aetiology of OSA in MND. Several studies report no association between bulbar weakness and SDB (8,20) while others observe that MND patients who do experience OSA often have evidence of bulbar dysfunction (10,42,43). It can be postulated that bulbar dysfunction may cause partial upper airways obstruction and that global muscle weakness limits patients’ ability to change sleeping position so they sleep predominantly supine, both of which may induce apnoeas (10). However, with worsening respiratory muscle weakness, the diaphragm may be increasingly unable to generate sufficient negative intrathoracic pressure to fully occlude the upper airways (8,12). Stiffening of upper airway muscles secondary to spasticity and increased stability of the upper airways due to significant weight loss may also be contributing factors as to why OSA may not be observed in some patients (12).
SDB in MND patients with preserved respiratory muscle function
Mild SDB has been demonstrated in MND patients with preserved respiratory muscle function [confirmed on FVC, VC, maximum inspiratory pressure (MIP) and maximum expiratory pressure (MEP) measurements] and with normal daytime ventilation on arterial blood gas, normal neurophysiology of respiratory muscles (demonstrated on phrenic nerve stimulation and needle EMG of the diaphragm) who do not use depressant or sedative drugs. The most common SDB identified was short cyclical clustering of mild hypoxaemia. There was no association with sleep stage, but these periods were associated with arousals and micro-arousals. Despite their normal respiratory muscle function, REM sleep was absent in over one third of this cohort (34).
In summary, patients with MND may experience various types of SDB. Patients have grossly altered sleep architecture compared to healthy patients, with reduced total sleep time, highly fragmented sleep and reduced or absent REM sleep stages. Nocturnal hypoventilation and obstructive sleep apnoeas are the most frequently observed forms of SDB amongst MND patients undergoing sleep studies. SDB occurs early in the course of MND and is predominantly caused by diaphragm weakness, although can occur in patients with normal respiratory muscle function. A multitude of other factors, including muscle cramps, difficulty becoming comfortable or turning in bed, nocturia, anxiety and depression, high secretion burden, choking and pain may also contribute to sleep disruption (8,34,45).
Effect of NIV on SDB
Commencing patients with MND on NIV should be considered when patients experience symptoms and signs of respiratory failure (including orthopnoea, dyspnoea, hypoxaemia, morning headaches, daytime somnolence, fatigue, accessory muscle use and paradoxical breathing) and daytime hypercapnia (5), or when there is evidence of respiratory muscle weakness (FVC <50% predicted, MIP <60 cmH2O, SNIP <40 cmH2O) (24). NIV initiation in the absence of respiratory symptoms is less well defined however should be considered in patients with evidence of SDB since this is widely considered to herald chronic respiratory failure which can be rapid in its onset and usually predicts poor prognosis.
NIV has been shown to improve quality of life for patients with MND. In a randomised controlled trial, Bourke and colleagues randomised MND patients with orthopnoea, MIP <60% predicted or symptomatic daytime hypercapnia to NIV or standard care. NIV was associated with sustained improvements in quality of life, with the greatest improvements observed in the domains relating to sleep problems, despite an observed reduction in REM sleep (4). This supports the findings of smaller prospective studies which have demonstrated sustained improvements in patient-reported outcomes amongst MND patients, including sleep quality, duration and efficiency, reduced sleep disturbance and improved and daytime somnolence, following initiation of NIV (42,46,47).
NIV also improves survival amongst patients with MND. Bourke and colleagues observed that median survival was significantly longer in the NIV group (219 days) compared to those receiving standard care (171 days). NIV conferred the greatest benefits in both sustained improvements in quality of life and survival in those with normal or moderate bulbar dysfunction and in those reporting orthopnoea. Those with severe bulbar impairment used NIV for shorter than those with better bulbar function (3.8 vs. 9.3 hours/day respectively) and had no survival benefit with NIV, but did have improved quality of life indicators and sleep related symptoms (4). This is in accordance with other studies that have demonstrated that NIV is less well tolerated in patients with bulbar involvement (46,48,49), but may increase duration of REM sleep and improve nocturnal oxygen saturations (50).
The effects of NIV on sleep architecture are reported variably. In Bourke and colleagues’ randomised controlled trial of MND patients, no differences were observed in sleep architecture, AHI or oximetry results between the NIV and control groups (4). However, in an observational cohort study examining MND patients before and 1 month after initiation of NIV, patients with no significant bulbar involvement demonstrated improvements in sleep efficiency, duration of N3 stage and REM sleep, arousal-awakening index, oxygenation and transcutaneous carbon dioxide following NIV titration (50).
Conclusions
MND is a progressive neuromuscular disease caused by loss of upper and lower motor neurones, with affected patients usually dying from respiratory failure. SDB arises in MND largely as a result of respiratory muscle weakness and commonly precedes chronic respiratory failure. Patients may experience altered sleep architecture, particularly reduced or absent REM sleep, restless legs, periodic limb movements, nocturnal hypoventilation and/or obstructive sleep apnoea. More research is needed to evaluate types of SDB with disease progression. SDB and respiratory failure in MND are managed with NIV. Tests of respiratory muscle function should be undertaken frequently in MND patients to facilitate early intervention with NIV, which improves patients’ quality of life and prolongs survival. More research is needed to investigate timing of NIV initiation.
Acknowledgements
Dr. RF D’Cruz is supported by the National Institute for Health Research (NIHR) Biomedical Research Centre at Guy’s and St Thomas’ NHS Foundation Trust and King’s College London.
Footnote
Conflicts of Interest: The authors have no conflicts of interest to declare.
References
- Chiò A, Mora G, Calvo A, et al. Epidemiology of ALS in Italy: a 10-year prospective population-based study. Neurology 2009;72:725-31. [Crossref] [PubMed]
- Alonso A, Logroscino G, Jick SS, et al. Incidence and lifetime risk of motor neuron disease in the United Kingdom: a population-based study. Eur J Neurol 2009;16:745-51. [Crossref] [PubMed]
- Mehta P, Kaye W, Bryan L, et al. Prevalence of Amytrophic Lateral Sclerosis - United States, 2012-2013. MMWR Surveill Summ 2016;65:1-12. [Crossref] [PubMed]
- Bourke SC, Tomlinson M, Williams TL, et al. Effects of non-invasive ventilation on survival and quality of life in patients with amyotrophic lateral sclerosis: a randomised controlled trial. Lancet Neurol 2006;5:140-7. [Crossref] [PubMed]
- Vrijsen B, Chatwin M, Contal O, et al. Hot Topics in Noninvasive Ventilation: Report of a Working Group at the International Symposium on Sleep-Disordered Breathing in Leuven, Belgium. Respir Care 2015;60:1337-62. [Crossref] [PubMed]
- de Carvalho M, Matias T, Coelho F, et al. Motor neuron disease presenting with respiratory failure. J Neurol Sci 1996;139:117-22. [Crossref] [PubMed]
- Carratù P, Cassano A, Gadaleta F, et al. Association between low sniff nasal-inspiratory pressure (SNIP) and sleep disordered breathing in amyotrophic lateral sclerosis: Preliminary results. Amyotroph Lateral Scler 2011;12:458-63. [Crossref] [PubMed]
- Ferguson KA, Strong MJ, Ahmad D, et al. Sleep-disordered breathing in amytrophic lateral sclerosis. Chest 1996;110:664-9. [Crossref] [PubMed]
- Bourke SC, Shaw PJ, Gibson GJ. Respiratory function vs sleep-disordered breathing as predictors of QOL in ALS. Neurology 2001;57:2040-4. [Crossref] [PubMed]
- Kimura K, Tachibana N, Kimura J, et al. Sleep-disordered breathing at an early stage of amyotrophic lateral sclerosis. J Neurol Sci 1999;164:37-43. [Crossref] [PubMed]
- National Institute for Health and Care Excellence. Motor neuron disease: assessment and management. NICE guideline [NG42] 2016. Available online: https://www.nice.org.uk/guidance/ng42
- Arnulf I, Similowski T, Salachas F, et al. Sleep disorders and diaphragmatic function in patients with amytrophic lateral sclerosis. Am J Respir Crit Care Med 2000;161:849-56. [Crossref] [PubMed]
- Schoser B, Fong E, Geberhiwot T, et al. Maximum inspiratory pressure as a clinically meaningful trial endpoint for neuromuscular diseases: a comprehensive review of the literature. Orphanet J Rare Dis 2017;12:52. [Crossref] [PubMed]
- Fromageot C, Lofaso F, Annane D, et al. Supine fall in lung volumes in the assessment of diaphragmatic weakness in neuromuscular disorders. Arch Phys Med Rehabil 2001;82:123-8. [Crossref] [PubMed]
- Maitre B, Similowski T, Derenne JP. Physical examination of the adult patient with respiratory diseases: inspection and palpation. Eur Respir J 1995;8:1584-93. [PubMed]
- Misuri G, Lanini B, Gigliotti F, et al. Mechanism of CO2 retention in patients with neuromuscular disease. Chest 2000;117:447-53. [Crossref] [PubMed]
- Allen SM, Hunt B, Green M. Fall in vital capacity with posture. Br J Dis Chest 1985;79:267-71. [Crossref] [PubMed]
- Kreitzer SM, Saunders NA, Tyler HR, et al. Respiratory muscle function in amyotrophic lateral sclerosis. Am Rev Respir Dis 1978;117:437-47. [PubMed]
- Polkey MI, Lyall RA, Yang K, et al. Respiratory Muscle Strength as a Predictive Biomarker for Survival in Amyotrophic Lateral Sclerosis. Am J Respir Crit Care Med 2017;195:86-95. [Crossref] [PubMed]
- Gay PC, Westbrook PR, Daube JR, et al. Effects of alterations in pulmonary function and sleep variables on survival in patients with amyotrophic lateral sclerosis. Mayo Clin Proc 1991;66:686-94. [Crossref] [PubMed]
- Chaudri MB, Liu C, Watson L, et al. Sniff nasal inspiratory pressure as a marker of respiratory function in motor neuron disease. Eur Respir J 2000;15:539-42. [Crossref] [PubMed]
- Fitting JW, Paillex R, Hirt L, et al. Sniff nasal pressure: a sensitive respiratory test to assess progression of amyotrophic lateral sclerosis. Ann Neurol 1999;46:887-93. [Crossref] [PubMed]
- Capozzo R, Quaranta VN, Pellegrini F, et al. Sniff nasal inspiratory pressure as a prognostic factor of tracheostomy or death in amyotrophic lateral sclerosis. J Neurol 2015;262:593-603. [Crossref] [PubMed]
- Benditt JO, Boitano LJ. Pulmonary issues in patients with chronic neuromuscular disease. Am J Respir Crit Care Med 2013;187:1046-55. [Crossref] [PubMed]
- Hamnegård CH, Wragg SD, Mills GH, et al. Clinical assessment of diaphragm strength by cervical magnetic stimulation of the phrenic nerves. Thorax 1996;51:1239-42. [Crossref] [PubMed]
- Douglas NJ, White DP, Pickett JK, et al. Respiration during sleep in normal man. Thorax 1982;37:840-4. [Crossref] [PubMed]
- Wiegand L, Zwillich CW, White DP. Collapsibility of the human upper airway during normal sleep. J Appl Physiol 1989;66:1800-8. [Crossref] [PubMed]
- Kubin L, Davies RO, Pack AI. Control of Upper Airway Motoneurons During REM Sleep. News Physiol Sci 1998;13:91-7. [PubMed]
- Douglas NJ, White DP, Weil JV, et al. Hypercapnic ventilatory response in sleeping adults. Am Rev Respir Dis 1982;126:758-62. [PubMed]
- Aboussouan LS, Mireles-Cabodevila E. Sleep-Disordered Breathing in Neuromuscular Disease: Diagnostic and Therapeutic Challenges. Chest 2017;152:880-92. [Crossref] [PubMed]
- Midgren B, Hansson L. Changes in transcutaneous PCO2 with sleep in normal subjects and in patients with chronic respiratory diseases. Eur J Respir Dis 1987;71:388-94. [PubMed]
- Ward S, Chatwin M, Heather S, et al. Randomised controlled trial of non-invasive ventilation (NIV) for nocturnal hypoventilation in neuromuscular and chest wall disease patients with daytime normocapnia. Thorax 2005;60:1019-24. [Crossref] [PubMed]
- Gaig C, Iranzo A. Sleep-disordered breathing in neurodegenerative diseases. Curr Neurol Neurosci Rep 2012;12:205-17. [Crossref] [PubMed]
- Atalaia A, De Carvalho M, Evangelista T, et al. Sleep characteristics of amyotrophic lateral sclerosis in patients with preserved diaphragmatic function. Amyotroph Lateral Scler 2007;8:101-5. [Crossref] [PubMed]
- Boentert M, Brenscheidt I, Glatz C, et al. Effects of non-invasive ventilation on objective sleep and nocturnal respiration in patients with amyotrophic lateral sclerosis. J Neurol 2015;262:2073-82. [Crossref] [PubMed]
- Lyall RA, Donaldson N, Fleming T, et al. A prospective study of quality of life in ALS patients treated with noninvasive ventilation. Neurology 2001;57:153-6. [Crossref] [PubMed]
- Minz M, Autret A, Laffont F, et al. A study on sleep in amyotrophic lateral sclerosis. Biomedicine 1979;30:40-6. [PubMed]
- Bennett JR, Dunroy HM, Corfield DR, et al. Respiratory muscle activity during REM sleep in patients with diaphragm paralysis. Neurology 2004;62:134-7. [Crossref] [PubMed]
- Steier J, Jolley CJ, Seymour J, et al. Sleep-disordered breathing in unilateral diaphragm paralysis or severe weakness. Eur Respir J 2008;32:1479-87. [Crossref] [PubMed]
- Skatrud J, Iber C, McHugh W, et al. Determinants of hypoventilation during wakefulness and sleep in diaphragmatic paralysis. Am Rev Respir Dis 1980;121:587-93. [Crossref] [PubMed]
- White JE, Drinnan MJ, Smithson AJ, et al. Respiratory muscle activity and oxygenation during sleep in patients with muscle weakness. Eur Respir J 1995;8:807-14. [PubMed]
- Lyall RA, Donaldson N, Polkey MI, et al. Respiratory muscle strength and ventilatory failure in amyotrophic lateral sclerosis. Brain 2001;124:2000-13. [Crossref] [PubMed]
- Santos C, Braghiroli A, Mazzini L, et al. Sleep-related breathing disorders in amyotrophic lateral sclerosis. Monaldi Arch Chest Dis 2003;59:160-5. [PubMed]
- Quaranta VN, Carratu P, Damiani MF, et al. The Prognostic Role of Obstructive Sleep Apnea at the Onset of Amyotrophic Lateral Sclerosis. Neurodegener Dis 2017;17:14-21. [Crossref] [PubMed]
- Lo Coco D, Mattaliano P, Spataro R, et al. Sleep-wake disturbances in patients with amyotrophic lateral sclerosis. J Neurol Neurosurg Psychiatry 2011;82:839-42. [Crossref] [PubMed]
- Bourke SC, Bullock RE, Williams TL, et al. Noninvasive ventilation in ALS: indications and effect on quality of life. Neurology 2003;61:171-7. [Crossref] [PubMed]
- Vandoorne E, Vrijsen B, Belge C, et al. Noninvasive ventilation in amyotrophic lateral sclerosis: effects on sleep quality and quality of life. Acta Clin Belg 2016;71:389-94. [Crossref] [PubMed]
- Aboussouan LS, Khan SU, Banerjee M, et al. Objective measures of the efficacy of noninvasive positivepressure ventilation in amyotrophic lateral sclerosis. Muscle Nerve 2001;24:403-9. [Crossref] [PubMed]
- Aboussouan LS, Khan SU, Meeker DP, et al. Effect of noninvasive positive-pressure ventilation on survival in amyotrophic lateral sclerosis. Ann Intern Med 1997;127:450-3. [Crossref] [PubMed]
- Vrijsen B, Buyse B, Belge C, et al. Noninvasive ventilation improves sleep in amyotrophic lateral sclerosis: a prospective polysomnographic study. J Clin Sleep Med 2015;11:559-66. [PubMed]