Screening for early stage lung cancer and its correlation with lung nodule detection
Introduction
Lung cancer is the leading cause of cancer-related deaths worldwide, accounting for an estimated 1.6 million deaths each year (1). The survival of lung cancer is strongly related to the stage of diagnosis, with 5-year overall survival decreasing from stage IA disease (85%) to stage IV disease (6%) (2). However, in routine clinical practice, many early-stage lung cancer were delayed in diagnosis, due to that most patients are often asymptomatic. Lung cancer screening is designed to reduce lung cancer related mortality through diagnosing the disease at its early stage in high-risk cohorts with relatively limited harm, in order to enable those patients to receive curative operations (3,4). Early screening trials generally assessed the efficacy of chest radiography (CXR) combined with sputum cytology, and showed no influence on lung cancer mortality (5-8). However, in the last decade, technologic advances in computed tomography (CT) re-brought imaging-based screening into the focus. The National Lung Screening Trial (NLST) demonstrated a 20% reduction in lung cancer mortality for low-dose computed tomography (LDCT) compared with CXR screening, as well as a 6.7% all-cause mortality reduction (9). Although the positive results are being issued, it remains to be seen whether the positive results of NLST can be reproduced by other randomized controlled screening trials. Liquid biopsy is a noninvasive approach to screening and early diagnosis of lung cancer (10,11). It may become one of the complementary or alternative approaches to thoracic imagery for the early diagnosis and screening for lung cancer. Also, how to balance the benefits and harms of the screening is still a controversial issue. Great concerns have arose from lung nodules detected from screening, such as false-positive results, overdiagnosis, false-negative results, physical and psychological problems. This paper briefly reviews the latest progress in lung cancer screening and the main unanswered questions on lung nodule detection, to discuss optimal strategies for implementation of lung cancer detection.
How to conduct screening
Screening by thoracic imagery
The NLST enrolled high-risk asymptomatic individuals: age, 55–74 years; current or former smokers who quit within 15 years with at least 30 pack-years of smoking history. From August 2002 through April 2004, 53,454 individuals were randomly assigned to annual LDCT screening scans versus CXR for three consecutive years. After a median follow-up of 6.5 years, the study showed that screening lead to a significant reduction of 20% and 6.7% in lung cancer and overall mortality, respectively. LDCT showed better performance for the detection of early-stage lung cancer, with 57% of screening-detected lung cancer cases of stages I or II, compared to only 39% in the CXR arm (9). This has led to the American Cancer Society’s guidelines now recommending LDCT screening in high risk individuals aged 55 to 80 years who have a 30-year pack smoking history and currently smoke or have quit within the past 15 years (12).
The impact of LDCT screening versus usual care or CXR have been also compared by several large-scale European randomized trials: Dutch-Belgian Randomized Lung Cancer Screening (NELSON) trial (13); Danish Lung Cancer Screening Trial (DLCST) (14); Detection and Screening of Early Lung Cancer by Novel Imaging Technology and Molecular Essays (DANTE) (15); Multicentric Italian Lung Detection (MILD) (16); Italian Lung Study (ITALUNG) (17); German Lung Cancer Screening Intervention Trial (LUSI) (18); UK Lung Cancer Screening Trial (UKLS) (19). So far, the three published trials have reported conflicting results against NLST. The DLCST showed higher rate of all-cause mortality in the CT screening arm compared with usual care [relative risk (RR) 1.03; 95% CI: 0.66–1.60] (20,21). In the DANTE trial, CT screening demonstrated no significant effect on reduce of mortality (RR 0.99; 95% CI: 0.69–1.43) (15). The MILD trial found no effect of biennial screening compared with usual care and a negative effect of annual screening with more detected cancers but also higher all-cause mortality (RR 1.99; 95% CI: 0.80–4.96) (16). It has raised questions about whether the much smaller patient sample size and the inclusion of patients at lower risk (less exposure to tobacco) for lung cancer in these trials diluted any potential benefits of LDCT screening (Table 1). The largest European trial, NELSON, has not yet been published statistical data regarding mortality reduction. The pooled analysis of all the European data should be powerful enough to compare with NLST and consolidate the evidence about the effectiveness of LDCT screening.
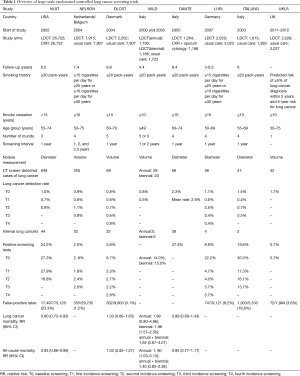
Full table
Screening by liquid biopsy
So far, European countries have not as yet recommended the use of imagery for screening for lung cancer. Considering the unjustified efficacy and high false positive rates, it is necessary to develop cost-effective and non-invasive methods that can replace or be used in conjunction with CT scans to screen for high risk cohort. Currently, histologic and cytologic results of biopsie are still the gold standard to make a definite diagnosis of lung nodules detected by CT screening. However, this approach is costly and often detects pulmonary nodules that turn out not to be cancers (22). Some researched answered this problem through searching potential biomarkers in peripheral blood samples and other accessible bodily fluids.
Circulating tumor cells (CTCs)
The two techniques most often used in the detection of CTCs are the indirect CellSearch method and the direct isolation by size of epithelial tumor cells (ISET) filtration method. Studies showed conflicting results regarding the correlation of CTC numbers and stage of cancer (23-26). Using the CellSearch system, the detection rates of stages I, II and III non-small cell lung cancers (NSCLCs) with ≥1 CTC was 36%, 45% and 40% respectively, compared with the using the positivity rates of 48%, 60% and 45% using ISET technology (25). CellSearch utilizes an epithelial cell adhesion molecule based enrichment step and detects CTCs as intact enucleated cells, staining negative for the leucocyte marker CD45 and positive for cytokeratins (27). However, the detection rates of the method were relatively low, particularly in early stage disease, owing to few CTCs undergo epithelial to mesenchymal transition and downregulate their epithelial markers. Studies have shown that CTCs from patients with lung cancer can be isolated and characterized by ISET filtration method before surgery, even from asymptomatic patients with stage I lung cancer (28,29). A recent study showed that CTCs can be isolated from patients with a high risk of developing lung cancer (smokers with chronic obstructive pulmonary disease) but without nodules detected on a thoracic CT scan (30). Of interest, the annual CT-scan surveillance of the CTC-positive patients detected lung nodules 1 to 4 years after CTC test. As a follow-up to this initial study, a multicenter study aims to include 600 patients aged over 55 years, with heavy smoke history and chronic obstructive pulmonary disease. These patients will be screened annually for 3 years with CTCs detection using ISET method and CT scan. It was also reported that a CTC count of >25 showed great sensitivity and specificity to differentiate lung cancers from benign diseases (31). CTCs may be the promising marker to predict malignancy of the lung nodules and a good tool for lung cancer detection with potential for clinical applicability.
Circulating nucleic acids
Compared with healthy controls or patients with benign diseases, serum or plasma DNA concentrations were significantly higher in lung cancer patients, seeing the possibility of applying DNA testing in screening assays (32-34). Catarino et al. reported that high DNA concentrations greater than 20.0 ng/mL showed great discriminating ability of lung cancer patients with a specificity of 83% and sensitivity of 79% (35). In the study of Newman et al., circulating tumor DNA (ctDNA) levels in NSCLC patients were found to be closely connected with tumor volume. They detected ctDNA in 50% of patients with stage I NSCLC and in 100% of patients with stage II–IV NSCLC, with 96% specificity for mutant allele fractions down to approximately 0.02% (36). However, when the technology put into clinical use, the detection has failed to show sufficient sensitivity yet. In a meta-analysis evaluating the diagnostic accuracy of ctDNA, the authors stated that ctDNA alone was not recommended for lung cancer screening on account of the disappointing discrimination power, with a pooled specificity of 77% and sensitivity of 80% (37). Unfortunately in another study, assessment of DNA level in plasma contributed little to improving the diagnostic accuracy of screening by thoracic CT in cohorts with heavy smoke history (38). Recent years, investigation into the methylation of free plasma DNA becomes an interesting option for lung cancer screening in a high-risk population, while the relatively low level may not be detected depending on the different techniques used and false positives are sometimes possible (39-41).
Currently, detection of circulating RNA typically requires capture of substances containing RNAs which are actively secreted from cells, due to the rapid degradation of RNAs in the circulation (42-45). Zander et al. found that the whole blood RNA allow for discrimination between NSCLC patients and healthy controls. It was also shown great efficacy in the further independent dataset of stage I NSCLC patients with the AUC of 0.977 (P<0.001) (46). However, there is still a long way to go for the RNA-stabilized whole blood-based technologies applying to the clinical use. It has been proven that small noncoding RNAs, including micro-RNAs, shows great stability in the circulation with the state of microRNA-processing proteins (47). The micro-RNAs have oncogenic and tumor suppressor gene function and exhibit specific expression profiles in lung cancer, and thus have been proposed as diagnostic and prognostic markers (48-51). In particular, the work of Montani et al. identified a signature of several plasma microRNA that showed an excellent predictive value for lung cancer in a high-risk population (52). Large validation study indicating that a specific micro-RNA plasma signature could reduce the false-positive rate of thoracic LDCT, thereby improving the efficacy of lung cancer screening (53).
Circulating proteins
Autoantibodies may be detected before the appearance of underlying cancers and testing for its level may facilitate earlier diagnosis to complement CT-based screening protocols (54,55). In the study of Boyle et al., the panel of autoantibodies can identify 40% of primary lung cancers, with a specificity of 90% against baseline-matched controls. The similar specificity was also found in patients with benign disease (56). Wang et al. discovered autoantibodies associated with lung adenocarcinoma that has the potential to differentiate cancer from CT-positive benign diseases (57). There were other protein signatures have been described for early screening for lung cancer (58-61). However, the patients involved in these studies mainly had stage III/IV lung cancer, and the control subjects were not matched by smoking, thus weakening them use as early detection markers. Moreover, all the aforementioned reported biomarkers require further independent validation in larger sample sets.
Who should be selected for screening
Studies indicated that cost-effectiveness estimates for LDCT screening for lung cancer differ from $18,452 to $66,480 per life-year gained and $27,756 to $243,077 per quality-adjusted life-year (QALY) gained (62-64). Although the threshold value for cost-effectiveness of lung cancer screening varies across countries and programmes, an incremental cost-effectiveness ratio below the threshold of $100,000 per QALY gained was reported in most studies (65). Cost-effectiveness mainly depends on the lung cancer risk of screened cohorts. The cost per QALY varied markedly according to subgroups, suggesting that screening was more cost-effective for current smokers, and those at higher risk and in women (66). It indicates that the risk-based selection of individuals eligible for lung cancer screening may be the key to improve the cost-effectiveness ratio (67).
In the USA, about 26% of lung cancer patients and 8.6 million of the general population would have met the NLST eligibility criteria (68). Based on the Prostate Lung Colorectal Ovarian (PLCO) cancer screening trial, it was suggested that most never-smokers would not benefit from screening instead of negative effect (69,70). Several studies showed that an adjunct smoking cessation program has the potential to improve outcomes and brings additional benefits (64,71-73). This emphasizes the importance of integrated smoking cessation within a computed tomography screening programme (74). Although tobacco use, including active smoking and passive exposure, is the major risk factor for lung cancer worldwide, an estimated 15% of lung cancer in men and 53% of lung cancer in women (25% of all cases) are not attributable to tobacco use (75). Epidemiological studies identified that lung cancer in never smokers occurs more frequently in the female sex than male. It is found a particularly high proportion of female lung cancer cases in never smokers in Asia (76). Although never-smokers have not included in most CT screening trials, model analyses show that those with RR of 15 to 35 have similar to better trade-offs between benefits and harms compared with ever-smokers eligible for lung cancer screening according to the U.S. Preventive Services Task Force (USPSTF) guidelines (77). Striking differences in the epidemiological, environmental exposures and molecular characteristics arising in non-smoker groups have not been identified yet. These strongly highlight the need for additional researches in smoking as well as non-smoking related risk factors for lung cancer in different ethnic groups. Also, a study assessed risk-based analyses based on the NLST to assist the identification of targeted population who could benefit the most. It was showed that 60% of the participants accounted for 88% of the averted lung cancer deaths, while 20% of participants at lowest risk accounted for only 1% of prevented lung-cancer deaths (78). The PLCOm2012 model included numerous risk factors to select high-risk individuals, and showed a significantly improved sensitivity and positive predictive value for selection of lung cancer screening cohort, without loss of specificity (79). Moreover, based on the Liverpool Lung Project model, the UKLS target a population with 5-year lung cancer risk >5%, and it came out the highest lung cancer detection rate (1.7%) among lung cancer screening trials (19). These evidences suggested that selection of screening cohort based on the risk model may be superior to age and pack-years alone.
When to conduct next screening
The screening interval has a direct influence on the balance of benefits and harms. Despite the mortality reduction found in the NLST was based on yearly screening, whether it is necessary to conduct annual LDCT in all eligible individuals remains uncertain (80,81). Retrospective analyses of the NLST suggest that participants with negative prevalence screen or subsequent negative screens might not be in need of annual screening, on account of the relatively lower incidence of lung cancer and lung cancer-specific mortality than all screened cohorts (80). In the hypothetical analysis, if participants with a negative baseline screen had not received the first incidence screening, it would have increased lung cancer mortality rate from 186 to 212 per 100,000 person-years. Nevertheless, this change of first incidence screenings could save about 73% of CT screens (19,066 of 26,231 participants), which could lead to reduction of false-positive results and health-care costs. In the MILD, the biennial screening showed the same efficacy as annual screening, according to the comparable stage distributions between two screening arms (82). Recent NELSON data showed that an interval of 2.5 years led to more interval cancers and significantly higher proportion of advanced stage lung cancers (stage IIIB–IV) (83). In the NELSON, it was also found that, when the baseline screening test result turned out negative, the probability of a lung cancer diagnosis in the next 2 years after the prevalence screen was only 0.4%. The results suggested that a screening interval of at least 2 years might be safe to apply in these individuals (84). However, for the nodule with volume of 1,000 mm3 or greater, the lung cancer probability is of 25.7%, and 31.6% for the nodule with diameter of 30 mm or greater. Immediate diagnostic work-up is suggested instead for these large nodules. Although annual screening is now recommended for screening, these results imply that the importance of using information obtained from baseline screening to optimize the intervals for different risk cohorts. Thus, it is likely, in the future, that screening programmes will adopt a combination of 1- and 2-year intervals according to risk.
What are the problems arise from nodule detection
False positive results
Based on a systematic review, it seems that suspicious non-calcified lung nodules were detected in approximately 20% of the LDCT scans performed and required further work-up for lung cancer diagnosis (85). Unfortunately, more than 90% of these screening findings turn out benign diseases and are thought as false positive screening results. These false positive results may lead to subsequent unnecessary diagnostic evaluation, for instance, fine-needle aspiration, bronchoscopy, even operation; these procedures accompany with risks of complications, negative psychological consequence, and increased health-care costs. The Interpretation criteria have a great influence on the screening test performance. The NLST set positive results as indeterminate nodules of a minimum of 4mm in diameter or other abnormality suspicious for lung cancer (86). Using this protocol, it turned out that at least one positive test result was found in 39.1% of the participants across the three rounds of yearly CT screens. However, of those with a positive result, 96.4% were proven as false-positive, of which 72.1% asked for downstream diagnostic evaluation. Overall, only 3.6% of all CT screens in the NLST resulted in a diagnosis of lung cancer, and 23.3% were false-positive. Although the further work-up for these positive screens brought out few medical complications (2.7%), there would be a substantial reduction of healthcare costs by improving the threshold of LDCT screening results (9,87). NELSON has conducted a two-step nodule management based on volume measurement to evaluate whether CT findings were positive. At baseline screening, nodules less than 50 µL in volume or 5 mm in diameter were considered as negative, nodules greater than 500 µL in volume or 10 mm in diameter were assumed to positive results and underwent definitive work-up procedures, and nodules varying between 5 and 10 mm in diameter were defined as indeterminate and suggested to undertake reassessment with LDCT 3 months later. Volumetric analysis of nodule growth at the follow-up scan was made to work out whether the scan was classified as positive or negative (88,89). Thanks to this interpretation strategy, 59.4% of participants with an initial positive test came out false-positive, resulting with an overall low false positive rate of 1.2% in four screening rounds (83). Considering the favorable positive predictive value, the two-step strategy used in the NELSON provides a much more effective management of screened results. In 2014, the American College of Radiology released Lung-RADS, a classification system for LDCT lung cancer screening. The criteria are different from the NLST protocol, mainly with the increase of threshold for positive results (from 4 to 6 mm diameter) and the use of growth for pre-existing nodules. When the criteria were applied to the NLST, the false-positive result rate was substantially reduced, whereas it also resulted in decreased sensitivity (90). Currently, it remains unknown how to balance the sensitivity of lung cancer detection and the false-positive rate (91). Therefore, an effective and noninvasive method to distinguish malignant nodules from benign ones is highly required.
Overdiagnosis
Another potential negative effect of screening arises from overdiagnosis, since the unnecessary diagnosis of an indolent cancer which would stay asymptomatic and not cause death. It may arise from either of the two scenarios: the indolent cancer progresses sufficiently slowly that it will not lead to death, or that the individual dies of competing risk such as a comorbidity or an unexpected event. Due to the biologically heterogeneous behavior of cancer, it should be taken into consideration that which cancers need to be early diagnosed in screening programs (92). These overdiagnosis cases are likely to be associated with additional cost, anxiety, even morbidity caused by treatment. Since all the diagnosed lung cancer patients undergo treatment, there is no available data of detected but untreated lung cancers, which generally would make up the overdiagnosis cohorts. Recently, it is possible to estimate an approximate overdiagnosis rate, through complex mathematical modeling studies based on lead time or tumor doubling times (93). Based on the excess incidence analyses of NLST, it was suggested that 18.5% of lung cancers diagnosed by CT screening were overtreated (94). Data from micro-simulation modelling suggested that the overdiagnosed rate was 8.6% of lung cancers detected by the NLST screening (95). As the screening protocol recommended by the USPSTF, an estimated 9.9% of all lung cancers detected by screening are overdiagnosed (96), which is relatively low compared with other screening programmes (67). Lindell et al. estimated that approximately 25% of cases may be indolent, by evaluating volume-doubling times on sequential LDCT (97). Several studies suggested to predict long-term indolence dependence on volumetric doubling time calculated during a limited period of observation of tumor growth (98-100). However, tumor growth may not always be linear and could instead be sigmoid shaped or accelerate after a dormant period of no growth (101). Unfortunately, it remains unclear the mechanisms to point out the exact time of this transformation.
False negative results
Diagnostic failures in observer performance may explain for the false negative results, as in the case of inaccurate interpretation or recognition error (102). Satisfaction of search error could be another type of false negative errors that occurs when multiple abnormalities are present on an examination, and the radiologist fails to report at least one of them (103). Attention to technical parameters, such as thin-slice thickness (≤1.25 mm), is an achievable way to avoid errors (104). Li et al. concluded that LDCT fails to identify small faint tumors mainly superimposed to normal anatomical structures or located in the context of other lung disease (105). Recently, analysis of NELSON demonstrated that human errors, interpretation errors, and detection errors counted for the main causes of failure in detecting lung cancers, owing to the location of lesions (endobronchial, pleural-attached, or adjacent to thickened bullous structure) (106). It was also reported that centrally located or endobronchial lesions were blind spots for radiologists to interpret CT scans (107). It should be noted that the current study only reports on missed cancers identified after screening had finished or cancers found at screening intervals, whereas lacks informative data of cancers which were diagnosed at subsequent screening rounds with an identifiable appearance on a previous CT (108). Moreover, the development of multislice CT technology has increased the sensitivity of nodule detection, as well as the workload of radiologists, which can result in errors of manual measurement (109). Recent years, the computer-aided detection (CAD) system has become a helpful method for the detection of small lung lesions on CT scans and can assist radiologist to identify early-stage lung cancer (110,111). Although high false-positive rate is also increased by the software, which limit its wider use to clinical routine. Efforts have been made for improving CAD algorithms to further reduce false-positivity (112).
Physical and psychological problems
The effective dose of radiation of LDCT is estimated to be 1.5 mSv per examination, but there is substantial variation in actual clinical practice. For men, the median cumulative effective dose was 9.3 mSv after 10 years of lung cancer screening; the dose was 13.0 mSv for women (113). Although it may be acceptable for individual risk, the large cohorts exposed to ionizing radiation could turn into considerable amount of radiation-induced cancers. Screening exposure to radiation associated with not only the screening procedure, but also the related further investigations. Analysis of ITALUNG data demonstrated that 22.6% of the dose was owing to further investigations and 77.4% to annual LDCT (114). In the NLST, the mean effective dose of screened individuals over 3 years was an estimated 8 mSv, which corresponds to potentially causing one radiation-induced death per 2,500 subjects screened (85). Considering one lung cancer death avoided per 320 subjects screened, it indicates that the benefits of screening surpass the risks from radiation exposure. In spite of the apparently favorable risk-benefit ratio, other screening regimens may be not achieve the same positive result. The enhancement of technology and optimization of screening implement may work out this problem in the future.
The screening also brings negative effects on the quality of life, especially emotional stress, which mainly arise from false-positives and overdiagnosed cases (115). In the NELSON, compared with patients with a negative screening result or even without screening, it was found a significant increase of distress in patient who had an indeterminate result and was required a follow-up CT at two months after the initial LDCT screening. Luckily, this short term emotional distress is reversible over time and disappeared at 2 years (116). Analysis of DLCST demonstrated that lung cancer CT-screening trials induced negative psychological consequences in both the CT group and the control group. Of interest, the CT group experienced less negative psychosocial consequences compared with the control group, and reassurance among those with normal screening results might account for this (117,118). During the whole screening process, lots of parts will definitely affect people in terms of anxiety and lung cancer-specific distress. Strengthen health education and optimize the screening strategy may contribute to relieve stress of trial participants. Further efforts should be made to minimize the effect of screening on health-related quality of life.
Conclusions
To date only one randomised controlled trial, the NLST, has shown a reduction in lung cancer mortality. Computed tomography screening is therefore the only population based intervention shown to achieve this other than smoking cessation. Guidelines and practices will likely evolve as more data become available on optimal strategies for screening. Risk prediction models show its potential to optimize selection of the most beneficial individuals and the appropriate screening intervals. However, owing to the large numbers of benign lung nodules unavoidably detected from screening, it is necessary to work out how to substantially reduce the false-positive rates, without affecting the reductions in lung cancer mortality. A large number of studies are presently looking for the ideal approach. Liquid biopsy seems to be the most promising one, and is noninvasive and can be easily repeated in contrast to tissue biopsy. However, despite several recent discoveries showed promise, none of the previously described circulating biomarkers that aim to detect lung cancer at an early stage is presently being used routinely in the clinic. The absence of technical standardization is a hurdle to the generalization to daily routine practice. The complementarity between the different medical expertise of radiologists, biologists, pathologists, clinicians and surgeons will certainly be one of the keys to the framework of early diagnosis and screening of lung cancer.
Acknowledgements
Funding: This work was supported by Shanghai Municipal Commission of Health and Family Planning (Grant No. 2013ZYJB0402). The funders played no role in the study design, data collection and analysis, decision to publish, or preparation of the manuscript.
Footnote
Conflicts of Interest: The authors have no conflicts of interest to declare.
References
- Ferlay J, Soerjomataram I, Dikshit R, et al. Cancer incidence and mortality worldwide: sources, methods and major patterns in GLOBOCAN 2012. Int J Cancer 2015;136:E359-86. [Crossref] [PubMed]
- Goldstraw P, Chansky K, Crowley J, et al. The IASLC Lung Cancer Staging Project: Proposals for Revision of the TNM Stage Groupings in the Forthcoming (Eighth) Edition of the TNM Classification for Lung Cancer. J Thorac Oncol 2016;11:39-51. [Crossref] [PubMed]
- Patz EF, Goodman PC, Bepler G. Screening for lung cancer. N Engl J Med 2000;343:1627-33. [Crossref] [PubMed]
- Henschke CI, Yankelevitz DF, Libby DM, et al. Survival of patients with stage I lung cancer detected on CT screening. N Engl J Med 2006;355:1763-71. [Crossref] [PubMed]
- Hocking WG, Tammemagi MC, Commins J, et al. Diagnostic evaluation following a positive lung screening chest radiograph in the Prostate, Lung, Colorectal, Ovarian (PLCO) Cancer Screening Trial. Lung Cancer 2013;82:238-44. [Crossref] [PubMed]
- Marcus PM, Bergstralh EJ, Fagerstrom RM, et al. Lung cancer mortality in the Mayo Lung Project: impact of extended follow-up. J Natl Cancer Inst 2000;92:1308-16. [Crossref] [PubMed]
- Kubik AK, Parkin DM, Zatloukal P. Czech Study on Lung Cancer Screening: post-trial follow-up of lung cancer deaths up to year 15 since enrollment. Cancer 2000;89:2363-8. [Crossref] [PubMed]
- Melamed MR. Lung cancer screening results in the National Cancer Institute New York study. Cancer 2000;89:2356-62. [Crossref] [PubMed]
- National Lung Screening Trial Research Team, Aberle DR, Adams AM, et al. Reduced lung-cancer mortality with low-dose computed tomographic screening. N Engl J Med 2011;365:395-409. [Crossref] [PubMed]
- Matikas A, Syrigos KN, Agelaki S. Circulating Biomarkers in Non-Small-Cell Lung Cancer: Current Status and Future Challenges. Clin Lung Cancer 2016;17:507-16. [Crossref] [PubMed]
- Levy B, Hu ZI, Cordova KN, et al. Clinical Utility of Liquid Diagnostic Platforms in Non-Small Cell Lung Cancer. Oncologist 2016;21:1121-30. [Crossref] [PubMed]
- Moyer VA. Screening for lung cancer: U.S. Preventive Services Task Force recommendation statement. Ann Intern Med 2014;160:330-8. [PubMed]
- van Iersel CA, de Koning HJ, Draisma G, et al. Risk-based selection from the general population in a screening trial: selection criteria, recruitment and power for the Dutch-Belgian randomised lung cancer multi-slice CT screening trial (NELSON). Int J Cancer 2007;120:868-74. [Crossref] [PubMed]
- Pedersen JH, Ashraf H, Dirksen A, et al. The Danish randomized lung cancer CT screening trial--overall design and results of the prevalence round. J Thorac Oncol 2009;4:608-14. [Crossref] [PubMed]
- Infante M, Cavuto S, Lutman FR, et al. Long-Term Follow-up Results of the DANTE Trial, a Randomized Study of Lung Cancer Screening with Spiral Computed Tomography. Am J Respir Crit Care Med 2015;191:1166-75. [Crossref] [PubMed]
- Pastorino U, Rossi M, Rosato V, et al. Annual or biennial CT screening versus observation in heavy smokers: 5-year results of the MILD trial. Eur J Cancer Prev 2012;21:308-15. [Crossref] [PubMed]
- Lopes Pegna A, Picozzi G, Mascalchi M, et al. Design, recruitment and baseline results of the ITALUNG trial for lung cancer screening with low-dose CT. Lung Cancer 2009;64:34-40. [Crossref] [PubMed]
- Becker N, Delorme S, Kauczor HU. LUSI: The German component of the European trial on the efficacy of multislice-CT for the early detection of lung cancer. Onkologie 2008;31:PO320.
- Field JK, Devaraj A, Baldwin DR, et al. UK Lung Cancer Screening trial (UKLS): Prevalence data at baseline. Lung Cancer 2014;83:S24-S5. [Crossref]
- Wille MM, Dirksen A, Ashraf H, et al. Results of the Randomized Danish Lung Cancer Screening Trial with Focus on High-Risk Profiling. Am J Respir Crit Care Med 2016;193:542-51. [Crossref] [PubMed]
- Saghir Z, Dirksen A, Ashraf H, et al. CT screening for lung cancer brings forward early disease. The randomised Danish Lung Cancer Screening Trial: status after five annual screening rounds with low-dose CT. Thorax 2012;67:296-301. [Crossref] [PubMed]
- Lokhandwala T, Bittoni MA, Dann RA, et al. Costs of Diagnostic Assessment for Lung Cancer: A Medicare Claims Analysis. Clin Lung Cancer 2017;18:e27-e34. [Crossref] [PubMed]
- Funaki S, Sawabata N, Nakagiri T, et al. Novel approach for detection of isolated tumor cells in pulmonary vein using negative selection method: morphological classification and clinical implications. Eur J Cardiothorac Surg 2011;40:322-7. [PubMed]
- Krebs MG, Sloane R, Priest L, et al. Evaluation and prognostic significance of circulating tumor cells in patients with non-small-cell lung cancer. J Clin Oncol 2011;29:1556-63. [Crossref] [PubMed]
- Hofman V, Ilie MI, Long E, et al. Detection of circulating tumor cells as a prognostic factor in patients undergoing radical surgery for non-small-cell lung carcinoma: comparison of the efficacy of the CellSearch Assay and the isolation by size of epithelial tumor cell method. Int J Cancer 2011;129:1651-60. [Crossref] [PubMed]
- Krebs MG, Hou JM, Sloane R, et al. Analysis of circulating tumor cells in patients with non-small cell lung cancer using epithelial marker-dependent and -independent approaches. J Thorac Oncol 2012;7:306-15. [Crossref] [PubMed]
- Allard WJ, Matera J, Miller MC, et al. Tumor cells circulate in the peripheral blood of all major carcinomas but not in healthy subjects or patients with nonmalignant diseases. Clin Cancer Res 2004;10:6897-904. [Crossref] [PubMed]
- Hofman V, Bonnetaud C, Ilie MI, et al. Preoperative circulating tumor cell detection using the isolation by size of epithelial tumor cell method for patients with lung cancer is a new prognostic biomarker. Clin Cancer Res 2011;17:827-35. [Crossref] [PubMed]
- Hofman V, Long E, Ilie M, et al. Morphological analysis of circulating tumour cells in patients undergoing surgery for non-small cell lung carcinoma using the isolation by size of epithelial tumour cell (ISET) method. Cytopathology 2012;23:30-8. [Crossref] [PubMed]
- Ilie M, Hofman V, Long-Mira E, et al. "Sentinel" circulating tumor cells allow early diagnosis of lung cancer in patients with chronic obstructive pulmonary disease. PLoS One 2014;9:e111597. [Crossref] [PubMed]
- Fiorelli A, Accardo M, Carelli E, et al. Circulating Tumor Cells in Diagnosing Lung Cancer: Clinical and Morphologic Analysis. Ann Thorac Surg 2015;99:1899-905. [Crossref] [PubMed]
- Sozzi G, Conte D, Leon M, et al. Quantification of free circulating DNA as a diagnostic marker in lung cancer. J Clin Oncol 2003;21:3902-8. [Crossref] [PubMed]
- Paci M, Maramotti S, Bellesia E, et al. Circulating plasma DNA as diagnostic biomarker in non-small cell lung cancer. Lung Cancer 2009;64:92-7. [Crossref] [PubMed]
- Xia S, Huang CC, Le M, et al. Genomic variations in plasma cell free DNA differentiate early stage lung cancers from normal controls. Lung Cancer 2015;90:78-84. [Crossref] [PubMed]
- Catarino R, Coelho A, Araujo A, et al. Circulating DNA: diagnostic tool and predictive marker for overall survival of NSCLC patients. PLoS One 2012;7:e38559. [Crossref] [PubMed]
- Newman AM, Bratman SV, To J, et al. An ultrasensitive method for quantitating circulating tumor DNA with broad patient coverage. Nat Med 2014;20:548-54. [Crossref] [PubMed]
- Zhang R, Shao F, Wu X, et al. Value of quantitative analysis of circulating cell free DNA as a screening tool for lung cancer: a meta-analysis. Lung Cancer 2010;69:225-31. [Crossref] [PubMed]
- Sozzi G, Roz L, Conte D, et al. Plasma DNA quantification in lung cancer computed tomography screening: five-year results of a prospective study. Am J Respir Crit Care Med 2009;179:69-74. [Crossref] [PubMed]
- Lehmann-Werman R, Neiman D, Zemmour H, et al. Identification of tissue-specific cell death using methylation patterns of circulating DNA. Proc Natl Acad Sci U S A 2016;113:E1826-34. [Crossref] [PubMed]
- Hulbert A, Jusue-Torres I, Stark A, et al. Early Detection of Lung Cancer Using DNA Promoter Hypermethylation in Plasma and Sputum. Clin Cancer Res 2017;23:1998-2005. [Crossref] [PubMed]
- Hubers AJ, Heideman DA, Duin S, et al. DNA hypermethylation analysis in sputum of asymptomatic subjects at risk for lung cancer participating in the NELSON trial: argument for maximum screening interval of 2 years. J Clin Pathol 2017;70:250-4. [Crossref] [PubMed]
- Kossenkov AV, Dawany N, Evans TL, et al. Peripheral immune cell gene expression predicts survival of patients with non-small cell lung cancer. PLoS One 2012;7:e34392. [Crossref] [PubMed]
- Showe MK, Vachani A, Kossenkov AV, et al. Gene expression profiles in peripheral blood mononuclear cells can distinguish patients with non-small cell lung cancer from patients with nonmalignant lung disease. Cancer Res 2009;69:9202-10. [Crossref] [PubMed]
- Chian CF, Hwang YT, Terng HJ, et al. Panels of tumor-derived RNA markers in peripheral blood of patients with non-small cell lung cancer: their dependence on age, gender and clinical stages. Oncotarget 2016;7:50582-95. [Crossref] [PubMed]
- Sheu CC, Chang MY, Chang HC, et al. Combined detection of CEA, CK-19 and c-met mRNAs in peripheral blood: a highly sensitive panel for potential molecular diagnosis of non-small cell lung cancer. Oncology 2006;70:203-11. [Crossref] [PubMed]
- Zander T, Hofmann A, Staratschek-Jox A, et al. Blood-based gene expression signatures in non-small cell lung cancer. Clin Cancer Res 2011;17:3360-7. [Crossref] [PubMed]
- Arroyo JD, Chevillet JR, Kroh EM, et al. Argonaute2 complexes carry a population of circulating microRNAs independent of vesicles in human plasma. Proc Natl Acad Sci U S A 2011;108:5003-8. [Crossref] [PubMed]
- Yanaihara N, Caplen N, Bowman E, et al. Unique microRNA molecular profiles in lung cancer diagnosis and prognosis. Cancer Cell 2006;9:189-98. [Crossref] [PubMed]
- Cazzoli R, Buttitta F, Di Nicola M, et al. microRNAs derived from circulating exosomes as noninvasive biomarkers for screening and diagnosing lung cancer. J Thorac Oncol 2013;8:1156-62. [Crossref] [PubMed]
- Boeri M, Verri C, Conte D, et al. MicroRNA signatures in tissues and plasma predict development and prognosis of computed tomography detected lung cancer. Proc Natl Acad Sci U S A 2011;108:3713-8. [Crossref] [PubMed]
- Tang D, Shen Y, Wang M, et al. Identification of plasma microRNAs as novel noninvasive biomarkers for early detection of lung cancer. Eur J Cancer Prev 2013;22:540-8. [Crossref] [PubMed]
- Montani F, Marzi MJ, Dezi F, et al. miR-Test: a blood test for lung cancer early detection. J Natl Cancer Inst 2015;107:djv063. [Crossref] [PubMed]
- Sozzi G, Boeri M, Rossi M, et al. Clinical utility of a plasma-based miRNA signature classifier within computed tomography lung cancer screening: a correlative MILD trial study. J Clin Oncol 2014;32:768-73. [Crossref] [PubMed]
- Farlow EC, Patel K, Basu S, et al. Development of a multiplexed tumor-associated autoantibody-based blood test for the detection of non-small cell lung cancer. Clin Cancer Res 2010;16:3452-62. [Crossref] [PubMed]
- Wu L, Chang W, Zhao J, et al. Development of autoantibody signatures as novel diagnostic biomarkers of non-small cell lung cancer. Clin Cancer Res 2010;16:3760-8. [PubMed]
- Boyle P, Chapman CJ, Holdenrieder S, et al. Clinical validation of an autoantibody test for lung cancer. Ann Oncol 2011;22:383-9. [Crossref] [PubMed]
- Wang J, Shivakumar S, Barker K, et al. Comparative Study of Autoantibody Responses between Lung Adenocarcinoma and Benign Pulmonary Nodules. J Thorac Oncol 2016;11:334-45. [Crossref] [PubMed]
- Louis E, Adriaensens P, Guedens W, et al. Detection of Lung Cancer through Metabolic Changes Measured in Blood Plasma. J Thorac Oncol 2016;11:516-23. [Crossref] [PubMed]
- Bigbee WL, Gopalakrishnan V, Weissfeld JL, et al. A multiplexed serum biomarker immunoassay panel discriminates clinical lung cancer patients from high-risk individuals found to be cancer-free by CT screening. J Thorac Oncol 2012;7:698-708. [Crossref] [PubMed]
- Vachani A, Pass HI, Rom WN, et al. Validation of a multiprotein plasma classifier to identify benign lung nodules. J Thorac Oncol 2015;10:629-37. [Crossref] [PubMed]
- Jung YJ, Katilius E, Ostroff RM, et al. Development of a Protein Biomarker Panel to Detect Non-Small-Cell Lung Cancer in Korea. Clin Lung Cancer 2017;18:e99-e107. [Crossref] [PubMed]
- Raymakers AJN, Mayo J, Lam S, et al. Cost-Effectiveness Analyses of Lung Cancer Screening Strategies Using Low-Dose Computed Tomography: a Systematic Review. Appl Health Econ Health Policy 2016;14:409-18. [Crossref] [PubMed]
- Black WC, Gareen IF, Soneji SS, et al. Cost-effectiveness of CT screening in the National Lung Screening Trial. N Engl J Med 2014;371:1793-802. [Crossref] [PubMed]
- Goffin JR, Flanagan WM, Miller AB, et al. Cost-effectiveness of Lung Cancer Screening in Canada. JAMA Oncol 2015;1:807-13. [Crossref] [PubMed]
- Puggina A, Broumas A, Ricciardi W, et al. Cost-effectiveness of screening for lung cancer with low-dose computed tomography: a systematic literature review. Eur J Public Health 2016;26:168-75. [Crossref] [PubMed]
- Kazerooni EA, Armstrong MR, Amorosa JK, et al. ACR CT Accreditation Program and the Lung Cancer Screening Program Designation. J Am Coll Radiol 2016;13:R30-4. [Crossref] [PubMed]
- Tammemagi MC. Application of risk prediction models to lung cancer screening: a review. J Thorac Imaging 2015;30:88-100. [Crossref] [PubMed]
- Pinsky PF, Berg CD. Applying the National Lung Screening Trial eligibility criteria to the US population: what percent of the population and of incident lung cancers would be covered? J Med Screen 2012;19:154-6. [Crossref] [PubMed]
- Tammemagi MC, Church TR, Hocking WG, et al. Evaluation of the lung cancer risks at which to screen ever- and never-smokers: screening rules applied to the PLCO and NLST cohorts. PLoS Med 2014;11:e1001764. [Crossref] [PubMed]
- Oken MM, Hocking WG, Kvale PA, et al. Screening by chest radiograph and lung cancer mortality: the Prostate, Lung, Colorectal, and Ovarian (PLCO) randomized trial. Jama 2011;306:1865-73. [Crossref] [PubMed]
- Villanti AC, Jiang Y, Abrams DB, et al. A cost-utility analysis of lung cancer screening and the additional benefits of incorporating smoking cessation interventions. PLoS One 2013;8:e71379. [Crossref] [PubMed]
- McMahon PM, Kong CY, Bouzan C, et al. Cost-effectiveness of computed tomography screening for lung cancer in the United States. J Thorac Oncol 2011;6:1841-8. [Crossref] [PubMed]
- Ten Haaf K, Tammemagi MC, Bondy SJ, et al. Performance and Cost-Effectiveness of Computed Tomography Lung Cancer Screening Scenarios in a Population-Based Setting: A Microsimulation Modeling Analysis in Ontario, Canada. PLoS Med 2017;14:e1002225. [Crossref] [PubMed]
- van der Aalst CM, van Klaveren RJ, van den Bergh KA, et al. The impact of a lung cancer computed tomography screening result on smoking abstinence. Eur Respir J 2011;37:1466-73. [Crossref] [PubMed]
- Torre LA, Bray F, Siegel RL, et al. Global cancer statistics, 2012. CA Cancer J Clin 2015;65:87-108. [Crossref] [PubMed]
- Sun S, Schiller JH, Gazdar AF. Lung cancer in never smokers--a different disease. Nat Rev Cancer 2007;7:778-90. [Crossref] [PubMed]
- Ten Haaf K, de Koning HJ. Should Never-Smokers at Increased Risk for Lung Cancer Be Screened? J Thorac Oncol 2015;10:1285-91. [Crossref] [PubMed]
- Kovalchik SA, Tammemagi M, Berg CD, et al. Targeting of low-dose CT screening according to the risk of lung-cancer death. N Engl J Med 2013;369:245-54. [Crossref] [PubMed]
- Tammemagi MC, Katki HA, Hocking WG, et al. Selection criteria for lung-cancer screening. N Engl J Med 2013;368:728-36. [Crossref] [PubMed]
- Patz EF, Greco E, Gatsonis C, et al. Lung cancer incidence and mortality in National Lung Screening Trial participants who underwent low-dose CT prevalence screening: a retrospective cohort analysis of a randomised, multicentre, diagnostic screening trial. The Lancet Oncology 2016;17:590-9. [Crossref] [PubMed]
- Ma J, Ward EM, Smith R, et al. Annual number of lung cancer deaths potentially avertable by screening in the United States. Cancer 2013;119:1381-5. [Crossref] [PubMed]
- Sverzellati N, Silva M, Calareso G, et al. Low-dose computed tomography for lung cancer screening: comparison of performance between annual and biennial screen. Eur Radiol 2016;26:3821-9. [Crossref] [PubMed]
- Yousaf-Khan U, van der Aalst C, de Jong PA, et al. Final screening round of the NELSON lung cancer screening trial: the effect of a 2.5-year screening interval. Thorax 2017;72:48-56. [Crossref] [PubMed]
- Horeweg N, van Rosmalen J, Heuvelmans MA, et al. Lung cancer probability in patients with CT-detected pulmonary nodules: a prespecified analysis of data from the NELSON trial of low-dose CT screening. The Lancet Oncology 2014;15:1332-41. [Crossref] [PubMed]
- Bach PB, Mirkin JN, Oliver TK, et al. Benefits and harms of CT screening for lung cancer: a systematic review. JAMA 2012;307:2418-29. [Crossref] [PubMed]
- Team NLSTR. The National Lung Screening Trial: Overview and Study Design. Radiology 2011;258:243-53. [Crossref] [PubMed]
- Church TR, Black WC, Aberle DR, et al. Results of initial low-dose computed tomographic screening for lung cancer. N Engl J Med 2013;368:1980-91. [Crossref] [PubMed]
- Horeweg N, van der Aalst CM, Vliegenthart R, et al. Volumetric computed tomography screening for lung cancer: three rounds of the NELSON trial. Eur Respir J 2013;42:1659-67. [Crossref] [PubMed]
- van Klaveren RJ, Oudkerk M, Prokop M, et al. Management of lung nodules detected by volume CT scanning. N Engl J Med 2009;361:2221-9. [Crossref] [PubMed]
- Pinsky PF, Gierada DS, Black W, et al. Performance of Lung-RADS in the National Lung Screening Trial: a retrospective assessment. Ann Intern Med 2015;162:485-91. [Crossref] [PubMed]
- Gierada DS, Pinsky P, Nath H, et al. Projected outcomes using different nodule sizes to define a positive CT lung cancer screening examination. J Natl Cancer Inst 2014.106. [PubMed]
- Marcus PM, Prorok PC, Miller AB, et al. Conceptualizing overdiagnosis in cancer screening. J Natl Cancer Inst 2015;107:djv014. [Crossref] [PubMed]
- Reich JM. A critical appraisal of overdiagnosis: estimates of its magnitude and implications for lung cancer screening. Thorax 2008;63:377-83. [Crossref] [PubMed]
- Patz EF Jr, Pinsky P, Gatsonis C, et al. Overdiagnosis in low-dose computed tomography screening for lung cancer. JAMA Intern Med 2014;174:269-74. [Crossref] [PubMed]
- Ten Haaf K, de Koning HJ. Overdiagnosis in lung cancer screening: why modelling is essential. J Epidemiol Community Health 2015;69:1035-9. [Crossref] [PubMed]
- de Koning HJ, Meza R, Plevritis SK, et al. Benefits and harms of computed tomography lung cancer screening strategies: a comparative modeling study for the US Preventive Services Task Force. Ann Intern Med 2014;160:311-20. [Crossref] [PubMed]
- Lindell RM, Hartman TE, Swensen SJ, et al. 5-year lung cancer screening experience: growth curves of 18 lung cancers compared to histologic type, CT attenuation, stage, survival, and size. Chest 2009;136:1586-95. [Crossref] [PubMed]
- Gavrielides MA, Kinnard LM, Myers KJ, et al. Noncalcified lung nodules: volumetric assessment with thoracic CT. Radiology 2009;251:26-37. [Crossref] [PubMed]
- Ko JP, Suh J, Ibidapo O, et al. Lung Adenocarcinoma: Correlation of Quantitative CT Findings with Pathologic Findings. Radiology 2016;280:931-9. [Crossref] [PubMed]
- Infante M, Berghmans T, Heuvelmans MA, et al. Slow-growing lung cancer as an emerging entity: from screening to clinical management. Eur Respir J 2013;42:1706-22. [Crossref] [PubMed]
- Friberg S, Mattson S. On the growth rates of human malignant tumors: implications for medical decision making. J Surg Oncol 1997;65:284-97. [Crossref] [PubMed]
- Fardanesh M, White C. Missed lung cancer on chest radiography and computed tomography. Semin Ultrasound CT MR 2012;33:280-7. [Crossref] [PubMed]
- Berbaum KS, Schartz KM, Caldwell RT, et al. Satisfaction of search from detection of pulmonary nodules in computed tomography of the chest. Acad Radiol 2013;20:194-201. [Crossref] [PubMed]
- Fischbach F, Knollmann F, Griesshaber V, et al. Detection of pulmonary nodules by multislice computed tomography: improved detection rate with reduced slice thickness. Eur Radiol 2003;13:2378-83. [Crossref] [PubMed]
- Li F, Sone S, Abe H, et al. Lung cancers missed at low-dose helical CT screening in a general population: comparison of clinical, histopathologic, and imaging findings. Radiology 2002;225:673-83. [Crossref] [PubMed]
- Horeweg N, Scholten ET, de Jong PA, et al. Detection of lung cancer through low-dose CT screening (NELSON): a prespecified analysis of screening test performance and interval cancers. Lancet Oncol 2014;15:1342-50. [Crossref] [PubMed]
- Veronesi G, Maisonneuve P, Spaggiari L, et al. Diagnostic performance of low-dose computed tomography screening for lung cancer over five years. J Thorac Oncol 2014;9:935-9. [Crossref] [PubMed]
- Devaraj A. Missed cancers in lung cancer screening--more than meets the eye. Eur Radiol 2015;25:89-91. [Crossref] [PubMed]
- Firmino M, Morais AH, Mendoca RM, et al. Computer-aided detection system for lung cancer in computed tomography scans: review and future prospects. Biomed Eng Online 2014;13:41. [Crossref] [PubMed]
- Armato SG 3rd, Li F, Giger ML, et al. Lung cancer: performance of automated lung nodule detection applied to cancers missed in a CT screening program. Radiology 2002;225:685-92. [Crossref] [PubMed]
- Li F, Arimura H, Suzuki K, et al. Computer-aided detection of peripheral lung cancers missed at CT: ROC analyses without and with localization. Radiology 2005;237:684-90. [Crossref] [PubMed]
- Fraioli F, Serra G, Passariello R. CAD (computed-aided detection) and CADx (computer aided diagnosis) systems in identifying and characterising lung nodules on chest CT: overview of research, developments and new prospects. Radiol Med 2010;115:385-402. [Crossref] [PubMed]
- National Comprehensive Cancer Network. NCCN Clinical Practice Guidelines in Oncology: lung cancer screening. Version 2. 2018.
- Mascalchi M, Mazzoni LN, Falchini M, et al. Dose exposure in the ITALUNG trial of lung cancer screening with low-dose CT. Br J Radiol 2012;85:1134-9. [Crossref] [PubMed]
- Slatore CG, Sullivan DR, Pappas M, et al. Patient-centered outcomes among lung cancer screening recipients with computed tomography: a systematic review. J Thorac Oncol 2014;9:927-34. [Crossref] [PubMed]
- van den Bergh KA, Essink-Bot ML, Borsboom GJ, et al. Long-term effects of lung cancer computed tomography screening on health-related quality of life: the NELSON trial. Eur Respir J 2011;38:154-61. [Crossref] [PubMed]
- Rasmussen JF, Siersma V, Pedersen JH, et al. Psychosocial consequences in the Danish randomised controlled lung cancer screening trial (DLCST). Lung Cancer 2015;87:65-72. [Crossref] [PubMed]
- Aggestrup LM, Hestbech MS, Siersma V, et al. Psychosocial consequences of allocation to lung cancer screening: a randomised controlled trial. BMJ Open 2012;2:e000663. [Crossref] [PubMed]