Combining radiation plus immunotherapy to improve systemic immune response
Introduction
Due to our increased understanding of the complexities of the relationship between cancer, the immune system, and the stroma within the tumor microenvironment, the goal of many new cancer therapeutics is no longer to directly kill cancer cells but instead harness the body’s ability to generate a tumor-specific immune response. By doing so, cancer therapy may not only treat local disease but may also possess the ability to decrease, or even eliminate metastatic disease entirely. By combining immunotherapy with RT, some patients demonstrate a reduction in disease burden outside the irradiated site, known as the abscopal effect. There are numerous novel clinical trials that are combining RT (traditionally used for local control) with immunotherapy to enhance T cell activation and improve both local and distant control. Additionally, recent advances in radiation oncology technologies have allowed for the treatment of multiple sites, which has resulted in improved outcomes in oligometastatic disease. Aside from improved outcomes via the direct reduction in tumor burden in the irradiated sites, evidence suggests that the overall reduction in tumor burden may allow for improved systemic immune response. Emerging evidence suggests these treatment modalities synergize well. Not only do these combinatorial therapies hold potential to cure metastatic disease, but also to prevent recurrence by generating tumor-specific immune memory.
RT—treatment for local control
In the early 2000s, the revolution in computer-driven radiotherapy technology enabled precise direction of radiation beams to specific tumor targets. The advent of 4-dimensional computed tomography (CT), MRI and on-board image-guided intensity-modulated radiotherapy (IMRT), stereotactic ablative radiotherapy (SABR), particle therapy have equipped radiation oncologists with novel tools to tightly conform ablative radiation doses to targets while avoiding inadvertent irradiation of surrounding normal tissues and critical organs. SABR, also called stereotactic body radiation therapy (SBRT), as a non-invasive curative therapy, achieves >90% local control, improves survival with minimal toxicity and has become standard therapy in medically inoperable peripherally located stage I non-small cell lung cancer (NSCLC) (1,2). Particularly for elderly patients, SBRT’s effectiveness based on lung cancer-specific survival and progression-free survival is the same in the elderly (>75 years old) as it is the average age population (<75 years old). SBRT is well tolerated with a low risk of toxicity (3). Compared to historical outcomes with surgery in the elderly, SBRT outcome is considered comparable for stage I disease but has less morbidity (3).
The pattern of failure study showed that the dominant failure of SBRT in stage I NSCLC is distant metastasis (10% to 20%), followed by regional lymph node recurrence (10% to 15%) and then local failure (5% to 10%) (4). Up to 1 in 6 patients who received SBRT for early-stage NSCLC may develop isolated local-regional recurrence that could be salvaged with definitive treatment (5). The first long-term results for the largest group of salvaged patients with local-regional recurrence after SBRT (n=103) was reported in ASCO 2017 annual meeting (Brooks et al.), 912 patients with clinically early-stage I–II NSCLC from MD Anderson Cancer Center (MDACC) were treated with SBRT with isolated local recurrence (LR, n=49) or regional recurrence (RR, n=53). Salvage was performed in 79.6% of LR and 90.6% of RR patients. Median follow-up from time of initial SBRT was 57.2 months. Five-year OS was 52% for LR and 27.8% for RR patients. Of LR and RR patients, those receiving salvage had significantly better 5-year OS compared to those not receiving salvage (57.9% LR, 31.1% RR, 0% no salvage; P=0.006). Five-year OS for LR salvaged patients was not statistically different from patients with NR (53.5% NR, P=0.92) and 5-year OS for salvaged RR was lower than that of NR (P=0.022). Sixty percent patients never recurred after salvage, but subsequent DM occurred in 27.6% of local-regional recurrent patients at a median of 10.5 months. No salvaged patient experienced grade 5 toxicity.
There is debate about the optimal treatment for operable stage I NSCLC. Majority of the population-based retrospective propensity-matched studies have indicated that SBRT has effectiveness comparable to that of surgery for this population, with reported 3-year overall survival rates of 48–91% and local control rates of 85–96% that is significantly better than conventional radiotherapy (5). A pooled analysis of two prospective randomized trials for operable patients showed a better overall survival rate at 3 years for SBRT than for surgery (6); however, the efficacy, pattern of failure, and toxicity reported were mostly based upon relatively short follow-ups and a small number of patients; therefore, larger studies with longer follow up are needed and are ongoing around the world. Recently, a phase II prospective study investigating SBRT for early-stage NSCLC with median follow-up of 7 years demonstrated outstanding OS of 47% with low rates of local (8%), regional (14%) and distant failure (14%) 7 years after SBRT, comparable to those of surgery but with lower toxicity (7). Secondary malignancy remains one of the most common issues with longer follow-up (21%), which is consistent with surgical data.
There are two major limitations of SBRT in treating early stage NSCLC. First, critical nearby normal tissue dose constraints such as esophagus, bronchial tree, brachial plexus, heart, major vessels etc. may limit the ablative dose that could be safely delivered (8); second, the efficacy of SBRT is reduced and toxicity is increased with increasing size of the lesion, particularly when the lesion is >5 cm (9). Most of SBRT’s outstanding clinical outcomes reported in the literature are based on lesions less than 5 cm, typically <3 cm, and are not close/next to critical normal structures.
Finally, we need to keep in mind that cancer is a biological disease, not just a technological challenge. As our ability to control local tumors improves with the use of new technology, the importance of systemic disease control increases—after all, in most cases it is metastatic disease that kills the patient. During the past decade, the development of genomic profile—based targeted therapies and immune checkpoint pathways—have revolutionized the management of stage IV lung cancer. More and more data indicates that cancer cells killed by radiation release tumor-associated antigens and immunoregulatory cytokines (10), thereby functioning as a kind of in situ vaccine against cancer; these cytokines also activate systemic, tumor-specific immune responses to eradicate tumors even outside the radiation field (the abscopal effect). These effects seem to be more prominent when the radiation administered with immunotherapy involves giving high (ablative) doses. This type of therapy for which we coined the term “I-SABR” (immunotherapy and SABR) (11) has many protocols underway for both early-stage disease and advanced cancer.
In summary: SABR/SBRT, a novel non-invasive approach with low toxicity, achieves outstanding clinical outcomes and is the standard treatment in medically inoperable stage I NSCLC. It remains controversial whether SBRT should be used for operable early stage NSCLC and more randomized studies are ongoing. The dominant pattern of failure after SBRT is distant metastasis, followed by regional or intra-lobar failure. Patients with isolated local/regional recurrence should be salvaged aggressively and long-term surveillance is crucial to detect early recurrence and secondary lung cancer. Combined SBRT with systemic therapy such as immunotherapy may further improve the efficacy and cure rate of many patients.
Immunotherapy—treatment for metastatic disease
Advances in immuno-oncology have resulted in several promising therapeutics for lung cancer, including immune checkpoint inhibitors, agents that target key cellular checkpoints critical for cancer cells to proliferate and evade immune detection and destruction. There are currently two checkpoints inhibitors that have passed all stages of FDA approval for the treatment of cancer in the United States. The first, anti-cytotoxic T-lymphocyte-associated protein 4 (CTLA-4), is a protein receptor expressed on the surface of T cells and serves as an inhibitor of T cell activation by competing with CD28, a co-stimulatory protein receptor. During immune states requiring increased T cell activation, CTLA-4 expression is increased, thus serving as a negative feedback mechanism. However, in the case of cancer treatment, this decrease in immune activation is undesirable. Antagonistic antibodies such as ipilimumab (Bristol Myers Squibb) have shown potential in several cancer types, most notably of which is metastatic melanoma. The second clinically approved immunotherapy antagonizes programmed cell death protein 1 (PD-1) and programmed cell death ligand 1 (PD-L1). Similar to CTLA-4, the activation of PD-1, when bound to PD-L1, leads to diminished immune response and increased apoptosis of T cells to prevent autoimmune reactions. Anti-PD-1 and anti-PD-L1 drugs nivolumab (Bristol Myers Squibb) and pembrolizumab (Merck) are currently in use for metastatic melanoma, advanced NSCLC, and head and neck squamous cell carcinomas.
Although immunotherapies targeting PD1 and CTLA4 have demonstrated clear benefit for some patients, most patients do not respond, and many that do later develop resistance (12-15). Clinical trials investigating anti-PD-1 for patients with metastatic lung cancer have shown enormous variations in response rates, and PD-L1 expression has not proven to be a reliable predictor of response (16,17). Despite these encouraging responses the vast majority of patients either do not respond to therapy or continue to progress after an initial response, thereby pushing us to evaluate combination therapies with traditional modalities to expand the benefit of immunotherapy to a great number of patients.
Combining RT and immunotherapy: combining local and systemic therapy
At the cellular level, investigators have determined mechanisms by which radiation to a primary tumor mass may result in the reduction of tumor burden at a secondary site. Most models have implemented the combination of radiation and either anti-CTLA-4 or anti-PD-L1 to prime the immune system (17-19).
In an effort to expand the benefit of immunotherapy, numerous trials are testing the combining radiation with immunotherapies, either concurrently or adjuvantly. A key principle of the synergism between RT and immunoncology (IO) is that the combination may induce a systemic response from the local treatment of RT. Known as the abscopal effect, this phenomenon has been seen in both preclinical and clinical settings (19). In some individuals, RT increases the expression of MHC I (20) and may stimulate T-cell priming for the creation of tumor-specific antigens which are then able to travel to and target distant sites of disease. Immunotherapy enhances the overall immune response and is believed to increase the likelihood of achieving abscopal response.
Researchers at the New York University Medical Center used a murine model of breast and colon cancer to understand the abscopal effect at a cellular level (18). Tumors were implanted in one flank on Day 0, and the contralateral flank on Day 2. RT was delivered to the primary tumor twelve days following implantation, and anti-CTLA-4 antibody was administered 2 days following radiation. Fractionated radiation, but not single fraction radiation, to the primary tumor mass induced regression of the secondary tumor when combined with an immunomodulatory agent (anti-CTLA-4). Moreover, this effect was realized when immunotherapy was initiated during the course of radiation, as opposed to following the completion of radiation. Analysis of the primary and secondary tumor tissue demonstrated the presence of tumor infiltrating lymphocytes (TILs), both CD4+ and CD8+. The expression of IFN-γ was found to correlate positively with the rejection of secondary tumor. The role of IFN-γ as a key signaling molecule was strengthened by the observation that CD8+ lymphocytes with tumor specific IFN-γ expression were more frequently identified in rejected secondary specimens.
Later, researchers from this same group demonstrated that the cytoplasm plays a key inhibitory role in immune upregulation following radiation (21). It is known that radiation results in the degradation of DNA which normally accumulates in the cytosol following radiation. The DNA sensor cGAS and downstream effector STING use this cytosolic DNA signal to promote the stimulation of IFN-β by cancer cells, which in turn activates dendritic cells critical to the priming of the CD8+ effector cells. While it had been previously demonstrated that three to five fractions of radiation successfully resulted in the abscopal effect better than a single high-dose fraction, Vanpouille-Box and colleagues elucidated that the potential mechanism for this effect may relate to the presence of cytosolic DNA. They determined that the activation of a DNA exonuclease, Trex1, occurred with radiation doses above 12 to 18 Gy in a single fraction, and did not happen when using three to five fractions of radiation below this dose threshold. The activation of Trex1 resulted in the degradation of the cytosolic DNA that was requisite to the downstream activation of CD8+ cells, as outlined above. Therefore, radiation can both up- and down-regulate the activation of the immune system via intracellular signaling.
Research by Wang and colleagues demonstrated that RT can abrogate resistance to anti-PD-1 immunotherapy via increased expression of MHC I. They created an anti-PD-1 resistant murine model by passaging 344SQ parental (Kras-mutated, p53-deficient) murine lung cancer cells in a host through multiple rounds of anti-PD-1 antibodies (17). While the 344SQ_P cells demonstrated the presence of MHC I, it was entirely absent in the 344SQ_R cell line. To test the hypothesis that the anti-PD-1 resistance could be resensitize through RT, mice inoculated with 344SQ_R tumors received 36 Gy cumulative dose across three fractions. After the 6th-day post treatment, mice were sacrificed and the tumor cells were analyzed using flow cytometry. Radiation significantly increased both MHC I and II. Next, the researchers treated 344SQ_R mice with RT followed by anti-PD-1 therapy. The mice demonstrated tumor regression in both primary (irradiated) and secondary (non-irradiated) lesions, demonstrating the ability for RT to abrogate resistance in an anti-PD-1 resistant tumor model.
To further investigate optimal timing of treatments, we currently have a phase II clinical trial underway for patients with lung and liver cancers to receive ipilimumab with either concurrent or sequential RT (NCT02239900). The sequential groups receive SBRT at the beginning of the second cycle of ipilimumab while the concurrent groups receive SBRT immediately after the first day of the first cycle of ipilimumab.
Despite the significant interest in combinatorial therapies, many patients still show minimal to no response to treatment. Improving our understanding of the complex mechanisms of resistance, optimal timing and dosing of radiation with immunotherapy, along with biological insights as to what mechanisms impair abscopal responses will be critical for determining the most reproducible efficacious strategy.
Treatment of oligometastatic disease: achieving local control for patients with limited systemic disease
Local consolidative therapy (LCT) in the context of oligometastatic disease
Currently, there is still much debate if patients with limited metastatic disease benefit from local therapy. Over the past decade, several retrospective and single arm prospective trials have suggested a benefit with aggressive LCT (defined as surgery and/or RT) in the setting of oligometastatic NSCLC. Much of this progress has been due to several recent scientific and technologic developments. First, maintenance therapy has been shown to be of benefit in patients with a clinical benefit after frontline treatment in the setting of metastatic NSCLC (22-24). Second, there has been an advent of targeted therapy agents for patients with driver mutations, a development that has improved outcomes substantially for subsets of the population. Finally, as previously mentioned, SBRT has been shown to be a viable option for robust control of individual malignant lesions, with acceptable toxicity.
To this end, data has continued to mount supporting LCT for patients with limited metastatic NSCLC who do not progress after first-line systemic therapy. An example of this evidence includes a propensity-matched retrospective analysis demonstrating that patients treated with comprehensive LCT experience longer PFS and OS than those that receive less aggressive treatment (25). In another study, investigators combined data from multiple institutions to determine which, if any, patients with oligometastatic disease, defined as 5 or fewer lesions, treated with LCT had long-term survival. The authors found that patients with metachronous disease and N0 lymph node status had a 5-year OS of almost 50%, analogous to patients with stage I disease (26). And in a phase I prospective dose escalation trial of patients with oligometastatic disease in multiple primary sites, it was found that while 80% of patients treated with SBRT experienced recurrent disease, 50% of patients could then receive RT to an additional site. Furthermore, the treatment was well tolerated overall, with patients with fewer lesions having superior survival. Thus, this study also concluded that SBRT was an appropriate treatment option as LCT for patients with oligometastatic disease (27).
The strongest available data to our knowledge to support LCT in the context of oligometastatic NSCLC was from a recent prospective, multi-institutional randomized trial comparing LCT with maintenance therapy/observation (MT/O) in patients with stage IV NSCLC and 3 or less metastatic lesions who did not progress after front-line systemic therapy (FLST). Our hypothesis was that consolidative therapy would improve progression-free survival in this setting. Appropriate FLST was defined as either ≥4 cycles of platinum doublet therapy or ≥3 months of EGFR/ALK inhibitor for patients with EGFR mutations/ALK rearrangements, respectively. Patients were randomized to either LCT [(chemo) radiation or surgical resection of all sites] +/− ongoing MT/O vs. MT/O alone. The MT/O was physician choice (from predefined standard-of-care options). Forty-nine patients were randomized (25 in LCT arm (RT =20, surgery + RT =3, surgery =1, early progression, no LCT =1), 8 of whom had EGFR/ALK alterations. The median PFS in the LCT arm was 11.9 months (95% CI, 5.4 months, NR), compared to 3.9 months (95% CI, 2.2–6.6 months) in the no LCT arm (HR =0.35; P=0.007). Importantly, time to the appearance of a new lesion was longer among patients in the LCT group than among patients in the MT/O group (11.9 vs. 5.7 months; P=0.0497) (28). We find it intriguing that time to new lesions was changed. Speculating on a potential mechanism, perhaps the treated gross tumors had reduced ability to seed distant sites, or perhaps the treated tumors activated a systemic T cell response which reduced the microscopic disease burden (Figure 1).
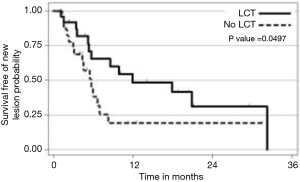
Immunotherapy plus LCT in the context of oligometastatic disease
As has occurred in many other malignancies and disease subtypes, the advent of checkpoint inhibitors has generated a paradigm shift and re-evaluation of standard therapy treatment options, similar to that which occurred with the development of targeted therapies, maintenance chemotherapy, and SBRT. Numerous ongoing trials are examining the effect and safety of combining RT and immunotherapy, though there are no reported outcomes to our knowledge of the effect of immunotherapy specifically on patients with oligometastatic disease. However, trials are now ongoing. For instance, a phase II trial of 42 patients at the University of Pennsylvania is examining the benefit of pembrolizumab after comprehensive LCT for oligometastatic NSCLC (NCT02316002). MD Anderson Cancer Center is performing a study addressing this question with the dual checkpoint blockade of nivolumab and ipilimumab. In this trial, patients with oligometastatic or polymetastatic NSCLC receive first line ipilimumab (1 mg/kg Q6 weeks) and nivolumab (3 mg/kg Q2 weeks) for 12 weeks. Patients who do not progress after this treatment are then randomized to continued checkpoint blockade or LCT to all clinically feasible sites followed by immunotherapy. The primary outcome of the study is overall survival, both in the full and oligometastatic populations. The results of this study should be informative both in defining the role of LCT in patients receiving immunotherapy, as well as determining which patient subsets may experience a synergistic effect with the combination of the two treatments.
The first randomized, double-blind, phase III trial investigating the combination of RT and immunotherapy in NSCLC was recently published (29). Researchers compared PD-L1 antagonist, Durvalumab, to placebo in patients with locally advanced, stage III NSCLC. Prior to receiving Durvalumab as consolidation therapy, all patients had not progressed after receiving definitive RT with platinum-based chemotherapy. Median progression free survival was significantly greater in the Durvalumab group compared to placebo (16.8 vs. 5.6 months). Twelve-month PFS was reported 55.9% vs. 35.3% in the same groups, respectively. Furthermore, response rate, median duration of response, and median time to death or distant metastasis were all significantly greater in patients who received consolidative Durvalumab compared to placebo, without significant differences in toxicity between groups. Studies such as these will help to define the standard of care going forward for patients with limited metastases.
Relationship between tumor burden and stroma with radiation and immunotherapy
Although RT and immunotherapy have demonstrated promising synergism, the mechanisms by which they synergize have not been fully elucidated. To study this, researchers are beginning to identify the critical relationships between both overall tumor bulk and composition of tumor stroma in cancer outcomes (30,31).
Negative effects of tumor stroma on outcomes
The main component of the tumor microenvironment in the majority of cancers, including NSCLC (32), is stromal tissue. Importantly, tumor stroma, consisting largely of cancer associated fibroblasts (CAFs), is phenotypically different and often highly metabolically active (33). The abnormal stroma within the tumor microenvironment can support malignant proliferation of cancer cells, prevent penetration of systemic therapies, induces radioresistance via hypoxia and upregulation of HIF-1α and enable tumor cells to eventually metastasize (33-35).
In a study examining resected tissue of patients with NSCLC, Zhang and colleagues highlighted the prognostic value of tumor stroma ratio (TSR) (32). TSR is defined as the ratio of tumor volume to stroma volume and was assessed in this study through hematoxylin and eosin staining of resected tissue. Patient groups were divided into stroma-rich (tissue with >50% stroma by volume) and stroma-poor (tissue with <50% stroma by volume). As predicted, the stroma-rich group had worse significantly worse outcomes. Multivariate analysis of 5-year survival OS revealed statistically significant HR and DFS of 1.748 and 1.570, respectively. Low TSR has been correlated with poor outcomes in other histologies of cancer, including breast, colorectal, esophageal, hepatocellular, but not cervical cancer (31).
While the relationship between radiation and immunotherapies and tumor stroma remains poorly understood, it is well established that CAFs and low TSR are associated with radioresistance and poor systemic drug penetration to tumor sites (31,36). Shortly, we will discuss a novel therapy which holds promising initial results in circumventing these treatment challenges.
Decreasing tumor burden improves outcomes
In the setting of relapsed metastatic disease, radiation has traditionally been used for palliative purposes only. However, recent developments in image guidance and stereotactic delivery have allowed clinicians to precisely define tumor location and geometry. As a result, radiation oncologists can now deliver ablative doses to metastases with minimal damage to surrounding healthy tissues. SBRT is now being increasingly utilized in the setting of oligometastatic disease to achieve local control and extend survival (28,37,38). However, these patients tend to fail distally with an average time to failure of about 12 months. This serves as rationale for combining stereotactic radiation with systemic agents. As discussed above, the synergism between radiation and immunotherapy is thought to hinge mostly on radiation-mediated release of antigens to stimulate the immune system. Sub-lethal radiation damage and its effect on the immune system has been studied extensively in in vitro studies (39).
Recently published findings suggest that there may be yet another mechanism by which treatment of oligometastatic disease with stereotactic radiation can potentiate immunomodulation. Huang and colleagues performed characterized factors influencing re-invigoration of exhausted T-cells in patients with metastatic melanoma receiving pembrolizumab (30). One of their findings was the importance of the overall tumor burden on the clinical outcomes in these patients. They found that the ratio of the degree of T-cell reinvigoration to tumor burden was directly proportional to progression-free survival (Figure 2).
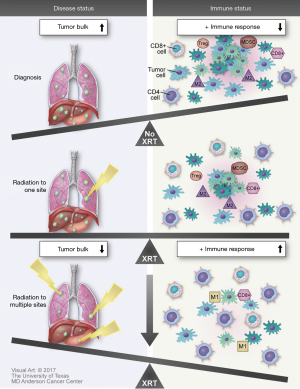
Various in vitro studies of chronic viral infections have found that antigen burden independently increases expression of inhibitory receptors on exhausted T cell (40,41). Comparison of transcriptional profiling in T effector cells from patients with malignancy vs. patients with chronic LCMV infections revealed almost identical molecular signatures (42). TILs typically have reduced effector function (43). Perhaps reduction in tumor burden leads to a decrease in the inhibitory signaling of these lymphocytes, thereby increasing the re-invigoration of circulating T-cells.
The significance of tumor burden for immunotherapy is supported by studies showing that combining RT with immunotherapy even in sequential order is beneficial. For instance, in a post-hoc analysis of patients enrolled in the phase I KEYNOTE-001 trial, Shaverdian and colleagues found that previous treatment with radiotherapy in patients with advanced NSCLC had longer progression free and overall survival when treated with pembrolizumab. The authors hypothesized that radiation-induced antigen release was the primary driver behind the enhanced clinical results in the radiotherapy group. However, the fact that radiotherapy could be delivered at any time point before the first cycle of pembrolizumab may be a testament to the tumor burden reduction hypothesis.
Macroscopic versus microscopic abscopal effects
As previously mentioned, combining RT with immunotherapy to harness abscopal effects holds potential to cure metastatic disease. Research is now beginning to investigate the mechanisms into reduction of tumor burden outside the irradiated site. However, it is important to also look beyond the benefits of macroscopic reduction in tumor volume. Largely undiscussed is the concept of microscopic abscopal effects. RT and immunotherapy create a robust, systemic, tumor specific immune response that can target metastatic microscopic disease. In doing so, the microscopic abscopal effects prevent further seeding of metastatic disease and may reduce the likelihood of recurrence, resulting in the clinical endpoint of progression free survival. Microscopic abscopal responses are difficult to distinguish but likely happen at a much greater frequency, due to lack of inhibitory stroma which blunts T cell penetration to the cancer cells. Large randomized trials will be integral to proving this. The PACFIC trial, which adds in immunotherapy after all the gross disease has been irritated in stage III lung cancer, is a great illustration of the potential benefits of how microscopic abscopal can lead to improved progression free survival. This is a concept that can likely be expanded to many histologies.
There are several methods aside from combination of immunotherapy with RT that may enhance abscopal effects. Irradiation of multiple lesions is likely to increase antigen presentation and T-cell priming systemically. Treatment with immunotherapies may further boost these effects, and also reduces undesired immunosuppressive cell populations such as myeloid derived suppressor cells (MDSCs) and Tregs. Together, these effects serve to further potentiate both macroscopic and microscopic abscopal response to therapy. Furthermore, providing RT to multiple tumors in a single patient may not only improve outcomes by reducing disease burden but also stimulate a more robust immune response. In theory, irradiation of multiple sites may lead to increased antigen stimulation, presentation, diversity and ultimately a greater immune response to not only metastatic lesions but also microscopic abscopal disease. Future studies examining multiple lesions in a single patient should consider analysis of the relationship between radiation exposure to TCR and neoantigen diversity, as well as long-term health outcomes.
Potential obstacles in reducing tumor burden
As the field advances, we will struggle with how many sites of disease can and should be targeted with radiation. One of the major obstacles we face is a radiation treatment work flow that is slow and cumbersome and optimized for treating only one isocenter at a time. Currently, each SBRT performed with onboard imaging takes around 20–30 minutes to treat. As such we are limited to around three isocenters per patient per day, making it unrealistic to treat patients with 4–20 sites of metastatic disease.
Advances in auto planning are already starting to take root and technologies such as VMAT have been shown to improve dose conformality reducing dose to organs at risk (OAR) (17). Clinical outcome data is limited in this area and most studies are from a dosimetric planning perspective. What is clear is the ability for VMAT to reduce treatment delivery time (17). However, this alone will not be enough to realistically treat ten isocenters with SBRT. New linear accelerators are being developed which can essentially aim radiation using PET as a fiducial in real time. Improved software will one day allow for autonomous contouring, planning, and delivery of SBRT all in one day (as we do for Gamma knife) making it possible for many isocenters to be addressed in one session. Such advancements will allow us to significantly push the limits of how much disease burden can be safely irritated.
Obstacles and potential methods for improving IO in patients with large tumor burden
During “tumor progression”, some cancer cells may acquire additional mutations, leading to the emergence of subclones with invasiveness, metastatic potential and response to treatment that could dramatically differ from the original cancer cells. This polyclonality represents a major obstacle for success of immunotherapy. To overcome this issue and improve immunotherapy (particularly in patients with large tumor burden, where SBRT seems inadequate), new approaches allowing a significant reduction of the tumor burden are needed. The use of nanoparticles (objects with a size comprised between 1 and 100 nm) could provide an innovative solution to address this problem (44).
A new class of nanoparticles composed of hafnium oxide has been designed to increase the radiation dose deposition from within the cancer cells. The high electron density of hafnium oxide renders feasibly efficient interactions with ionizing radiations. The size, shape and surface of these nanoparticles allow specific interactions with cancer cells. These nanoparticles form clusters in the cytoplasm, where they locally enhance the energy deposit (“hot spots”) when exposed to RT.
In vitro experiments on numerous human cancer cells lines have shown the greatest capacity of hafnium oxide nanoparticles exposed to radiation to kill cancer cells when compared to radiation alone (45). Marked antitumor efficacies were also observed in vivo with presence of these nanoparticles exposed to radiotherapy when compared to radiotherapy alone (46). Hafnium oxide nanoparticles are intended for a single intratumoral injection and persist within the tumor structure during all radiotherapy sessions. Promising results with favorable tumor shrinkage have been observed in a recent human study that treated patients with locally advanced soft tissue sarcomas (STS)—which are mostly large tumors (47). The product is currently being evaluated in a phase II/III trial in STS and for a first-in-human study for patients with head & neck, liver, rectal and prostate cancers.
It is now well established that radiotherapy has the ability to generate an antitumor response (48). Hafnium oxide nanoparticles have the same mode of action than radiotherapy and trigger hot spot of energy deposit within the cancer cells when exposed to ionizing radiations, enhancing cancer cell killing. Possibly, this could lead to a stronger antitumor immune response compared to irradiation alone. Results from non-clinical studies and preliminary data in patients with STS seem to confirm this hypothesis, but further evaluations are needed.
Another potential challenge in achieving abscopal response in the treatment of lung cancer involves intra-tumor heterogeneity (ITH). Recent research has revealed that within single patients and even single tumors, there can be significantly different genetic profiles, T cell receptor (TCR) repertoires, and neoantigens (49). Importantly, heterogeneity of these characteristics has been associated with poorer response to treatments, increased risk of relapse, and shorter disease-free survival.
Conclusions
Over past decade, we have achieved significant advancements in the understanding of the immune landscape and its interplay in lung cancer. Regardless, mortality remains high, control of metastatic disease remains poor, and the ability to consistently prevent recurrence remains elusive. Technologies such as SBRT have created a favorable option in early stage, localized lung cancers and provide a non-invasive alternative to surgery. However, SBRT still has limitations. It is possible that better understanding of relationship between tumor burden, RT for “priming” the immune system, and immunotherapies will allow for lower dose and broader targeting of multiple tumor sites rather than focusing entirely on local control of one or two sites of disease. Combination of SBRT with immunotherapy in early stage lung cancers may decrease patterns of failure such as distant metastases or local recurrence. Existing and future clinical trials may consider adding an arm focusing on stage I/II lung cancers.
With numerous immunotherapies in development and countless preclinical studies delving into the mechanisms of treatment resistance and the critical cellular and metabolic pathways involved, there is much to be excited about. Anti-CTLA4 and anti-PD-1/PD-L1 drugs have paved the way for immuno-oncology as a field. To tackle obstacles in the control of late stage cancers and recurrence, though, will require more than trial and error of various timing and dosage strategies of radiation and immunotherapies. Recognition of abscopal effects in preclinical models and the clinical setting has allowed for researchers to begin uncovering the integral players in these pathways. Expanding knowledge of cellular pathways such as STING and Trex1, checkpoints for cell and immune activation/suppression, tumor-specific memory and tumor specific antigen stimulation will not only allow for optimal timing and sequence of treatments but also allow for likely development of novel therapies. The next step for research in this area is profiling and identifying checkpoints and other immunologic mechanisms by which tumors are able to evade abscopal responses with specific focus on each type of cancer.
Acknowledgements
None.
Footnote
Conflicts of Interest: S Paris is an employee for Nanobiotix. The other authors have no conflicts of interest to declare.
References
- Timmerman R, Paulus R, Galvin J, et al. Stereotactic body radiation therapy for inoperable early stage lung cancer. JAMA 2010;303:1070-6. [Crossref] [PubMed]
- Onishi H, Araki T, Shirato H, et al. Stereotactic hypofractionated high-dose irradiation for stage I nonsmall cell lung carcinoma: clinical outcomes in 245 subjects in a Japanese multiinstitutional study. Cancer 2004;101:1623-31. [Crossref] [PubMed]
- Brooks ED, Sun B, Zhao L, et al. Stereotactic Ablative Radiation Therapy is Highly Safe and Effective for Elderly Patients With Early-stage Non-Small Cell Lung Cancer. Int J Radiat Oncol Biol Phys 2017;98:900-7. [Crossref] [PubMed]
- Senthi S, Lagerwaard FJ, Haasbeek CJ, et al. Patterns of disease recurrence after stereotactic ablative radiotherapy for early stage non-small-cell lung cancer: a retrospective analysis. Lancet Oncol 2012;13:802-9. [Crossref] [PubMed]
- Shirvani SM, Jiang J, Chang JY, et al. Lobectomy, sublobar resection, and stereotactic ablative radiotherapy for early-stage non-small cell lung cancers in the elderly. JAMA Surg 2014;149:1244-53. [Crossref] [PubMed]
- Chang JY, Senan S, Paul MA, et al. Stereotactic ablative radiotherapy versus lobectomy for operable stage I non-small-cell lung cancer: a pooled analysis of two randomised trials. Lancet Oncol 2015;16:630-7. [Crossref] [PubMed]
- Sun B, Brooks ED, Komaki RU, et al. 7-year follow-up after stereotactic ablative radiotherapy for patients with stage I non-small cell lung cancer: Results of a phase 2 clinical trial. Cancer 2017;123:3031-9. [Crossref] [PubMed]
- Timmerman R, McGarry R, Yiannoutsos C, et al. Excessive toxicity when treating central tumors in a phase II study of stereotactic body radiation therapy for medically inoperable early-stage lung cancer. J Clin Oncol 2006;24:4833-9. [Crossref] [PubMed]
- Tekatli H, van 't Hof S, Nossent EJ, et al. Use of Stereotactic Ablative Radiotherapy (SABR) in Non-Small Cell Lung Cancer Measuring More Than 5 cm. J Thorac Oncol 2017;12:974-82. [Crossref] [PubMed]
- Lugade AA, Moran JP, Gerber SA, et al. Local radiation therapy of B16 melanoma tumors increases the generation of tumor antigen-specific effector cells that traffic to the tumor. J Immunol 2005;174:7516-23. [Crossref] [PubMed]
- Bernstein MB, Krishnan S, Hodge JW, et al. Immunotherapy and stereotactic ablative radiotherapy (ISABR): a curative approach? Nat Rev Clin Oncol 2016;13:516-24. [Crossref] [PubMed]
- Gettinger S, Herbst RS. B7-H1/PD-1 blockade therapy in non-small cell lung cancer: current status and future direction. Cancer J 2014;20:281-9. [Crossref] [PubMed]
- Schulze AB, Schmidt LH. PD-1 targeted Immunotherapy as first-line therapy for advanced non-small-cell lung cancer patients. J Thorac Dis 2017;9:E384-6. [Crossref] [PubMed]
- Topalian SL, Hodi FS, Brahmer JR, et al. Safety, activity, and immune correlates of anti-PD-1 antibody in cancer. N Engl J Med 2012;366:2443-54. [Crossref] [PubMed]
- Zaretsky JM, Garcia-Diaz A, Shin DS, et al. Mutations Associated with Acquired Resistance to PD-1 Blockade in Melanoma. N Engl J Med 2016;375:819-29. [Crossref] [PubMed]
- Hui R, Garon EB, Goldman JW, et al. Pembrolizumab as first-line therapy for patients with PD-L1-positive advanced non-small cell lung cancer: a phase 1 trial. Ann Oncol 2017;28:874-81. [Crossref] [PubMed]
- Wang X, Schoenhals JE, Li A, et al. Suppression of Type I IFN Signaling in Tumors Mediates Resistance to Anti-PD-1 Treatment That Can Be Overcome by Radiotherapy. Cancer Res 2017;77:839-50. [Crossref] [PubMed]
- Dewan MZ, Galloway AE, Kawashima N, et al. Fractionated but not single-dose radiotherapy induces an immune-mediated abscopal effect when combined with anti-CTLA-4 antibody. Clin Cancer Res 2009;15:5379-88. [Crossref] [PubMed]
- Schoenhals JE, Seyedin SN, Tang C, et al. Preclinical Rationale and Clinical Considerations for Radiotherapy Plus Immunotherapy: Going Beyond Local Control. Cancer J 2016;22:130-7. [Crossref] [PubMed]
- Wan S, Pestka S, Jubin RG, et al. Chemotherapeutics and radiation stimulate MHC class I expression through elevated interferon-beta signaling in breast cancer cells. PLoS One 2012;7:e32542. [Crossref] [PubMed]
- Vanpouille-Box C, Alard A, Aryankalayil MJ, et al. DNA exonuclease Trex1 regulates radiotherapy-induced tumour immunogenicity. Nat Commun 2017;8:15618. [Crossref] [PubMed]
- Cappuzzo F, Ciuleanu T, Stelmakh L, et al. Erlotinib as maintenance treatment in advanced non-small-cell lung cancer: a multicentre, randomised, placebo-controlled phase 3 study. Lancet Oncol 2010;11:521-9. [Crossref] [PubMed]
- Ciuleanu T, Brodowicz T, Zielinski C, et al. Maintenance pemetrexed plus best supportive care versus placebo plus best supportive care for non-small-cell lung cancer: a randomised, double-blind, phase 3 study. Lancet 2009;374:1432-40. [Crossref] [PubMed]
- Fidias PM, Dakhil SR, Lyss AP, et al. Phase III study of immediate compared with delayed docetaxel after front-line therapy with gemcitabine plus carboplatin in advanced non-small-cell lung cancer. J Clin Oncol 2009;27:591-8. [Crossref] [PubMed]
- Sheu T, Heymach JV, Swisher SG, et al. Propensity score-matched analysis of comprehensive local therapy for oligometastatic non-small cell lung cancer that did not progress after front-line chemotherapy. Int J Radiat Oncol Biol Phys 2014;90:850-7. [Crossref] [PubMed]
- Ashworth AB, Senan S, Palma DA, et al. An individual patient data metaanalysis of outcomes and prognostic factors after treatment of oligometastatic non-small-cell lung cancer. Clin Lung Cancer 2014;15:346-55. [Crossref] [PubMed]
- Salama JK, Chmura SJ, Mehta N, et al. An initial report of a radiation dose-escalation trial in patients with one to five sites of metastatic disease. Clin Cancer Res 2008;14:5255-9. [Crossref] [PubMed]
- Gomez DR, Blumenschein GR Jr, Lee JJ, et al. Local consolidative therapy versus maintenance therapy or observation for patients with oligometastatic non-small-cell lung cancer without progression after first-line systemic therapy: a multicentre, randomised, controlled, phase 2 study. Lancet Oncol 2016;17:1672-82. [Crossref] [PubMed]
- Antonia SJ, Villegas A, Daniel D, et al. Durvalumab after Chemoradiotherapy in Stage III Non-Small-Cell Lung Cancer. N Engl J Med 2017;377:1919-29. [Crossref] [PubMed]
- Huang AC, Postow MA, Orlowski RJ, et al. T-cell invigoration to tumour burden ratio associated with anti-PD-1 response. Nature 2017;545:60-5. [Crossref] [PubMed]
- Wu J, Liang C, Chen M, et al. Association between tumor-stroma ratio and prognosis in solid tumor patients: a systematic review and meta-analysis. Oncotarget 2016;7:68954-65. [PubMed]
- Zhang T, Xu J, Shen H, et al. Tumor-stroma ratio is an independent predictor for survival in NSCLC. Int J Clin Exp Pathol 2015;8:11348-55. [PubMed]
- Bissell MJ, Radisky D. Putting tumours in context. Nat Rev Cancer 2001;1:46-54. [Crossref] [PubMed]
- De Wever O, Mareel M. Role of tissue stroma in cancer cell invasion. J Pathol 2003;200:429-47. [Crossref] [PubMed]
- Pietras K, Ostman A. Hallmarks of cancer: interactions with the tumor stroma. Exp Cell Res 2010;316:1324-31. [Crossref] [PubMed]
- Barker HE, Paget JT, Khan AA, et al. The tumour microenvironment after radiotherapy: mechanisms of resistance and recurrence. Nat Rev Cancer 2015;15:409-25. [Crossref] [PubMed]
- Iyengar P, Kavanagh BD, Wardak Z, et al. Phase II trial of stereotactic body radiation therapy combined with erlotinib for patients with limited but progressive metastatic non-small-cell lung cancer. J Clin Oncol 2014;32:3824-30. [Crossref] [PubMed]
- Rusthoven KE, Kavanagh BD, Burri SH, et al. Multi-institutional phase I/II trial of stereotactic body radiation therapy for lung metastases. J Clin Oncol 2009;27:1579-84. [Crossref] [PubMed]
- Cao ZA, Daniel D, Hanahan D. Sub-lethal radiation enhances anti-tumor immunotherapy in a transgenic mouse model of pancreatic cancer. BMC Cancer 2002;2:11. [Crossref] [PubMed]
- Blackburn SD, Shin H, Haining WN, et al. Coregulation of CD8+ T cell exhaustion by multiple inhibitory receptors during chronic viral infection. Nat Immunol 2009;10:29-37. [Crossref] [PubMed]
- Wherry EJ, Blattman JN, Murali-Krishna K, et al. Viral persistence alters CD8 T-cell immunodominance and tissue distribution and results in distinct stages of functional impairment. J Virol 2003;77:4911-27. [Crossref] [PubMed]
- Baitsch L, Baumgaertner P, Devevre E, et al. Exhaustion of tumor-specific CD8(+) T cells in metastases from melanoma patients. J Clin Invest 2011;121:2350-60. [Crossref] [PubMed]
- Ahmadzadeh M, Johnson LA, Heemskerk B, et al. Tumor antigen-specific CD8 T cells infiltrating the tumor express high levels of PD-1 and are functionally impaired. Blood 2009;114:1537-44. [Crossref] [PubMed]
- Pottier A, Borghi E, Levy L. The future of nanosized radiation enhancers. Br J Radiol 2015;88:20150171. [Crossref] [PubMed]
- Marill J, Anesary NM, Zhang P, et al. Hafnium oxide nanoparticles: toward an in vitro predictive biological effect? Radiat Oncol 2014;9:150. [Crossref] [PubMed]
- Maggiorella L, Barouch G, Devaux C, et al. Nanoscale radiotherapy with hafnium oxide nanoparticles. Future Oncol 2012;8:1167-81. [Crossref] [PubMed]
- Bonvalot S, Le Pechoux C, De Baere T, et al. First-in-Human Study Testing a New Radioenhancer Using Nanoparticles (NBTXR3) Activated by Radiation Therapy in Patients with Locally Advanced Soft Tissue Sarcomas. Clin Cancer Res 2017;23:908-17. [Crossref] [PubMed]
- Demaria S, Coleman CN, Formenti SC. Radiotherapy: Changing the Game in Immunotherapy. Trends Cancer 2016;2:286-94. [Crossref] [PubMed]
- Zhang J, Fujimoto J, Zhang J, et al. Intratumor heterogeneity in localized lung adenocarcinomas delineated by multiregion sequencing. Science 2014;346:256-9. [Crossref] [PubMed]