Using novel technology to augment complex video-assisted thoracoscopic single basilar segmentectomy
Introduction
Lung cancer is the most commonly diagnosed cancer and the leading cause of cancer mortality worldwide, representing about 13.2% of all new cancer cases and 25.9% of all cancer deaths (1). Surgery is the first choice for curative treatment for medically operable patients with early stage non-small cell lung cancer (NSCLC). Several trials such as the CALGB140503 (2) and the JCOG 0802-WJOG4607L (3) trials are awaiting maturation to provide contemporary data on the comparative efficacy of sublobar versus lobar resections. Until then, current clinical practice guidelines include a preference for segmental resection for patients with multifocal lung cancer, elderly or high risk operable candidates, and selected recurrent tumors or tumors less than 2 cm where segmentectomy achieves at least a 1:1 parenchymal margin and no regional nodal disease on frozen pathologic analysis (4-7). In this paper we describe the utilization of multiple novel technologies, and the proper steps to optimize resection. We will review individual basilar segmentectomy (S10). We will specifically discuss and illustrate minimal access approaches, 3D printing to augment planning, ICG technology, and inflation techniques.
Technique
As with any minimally invasive lung surgery, a complete history and physical as well as analysis of risk must be performed in the clinic. Prior to making the decision to provide any operative approach, the surgeon should evaluate the patient’s pulmonary function status, underlying comorbidities, frailty, staging, and any other risk factors that may be present including the presence of other lung tumors and the patient’s ability to undergo surgery. Patients presenting with early lung cancer are offered a CT scan of the chest and a PET scan. Those with a single focus of disease, tumors less than 2 cm, and no evidence of mediastinal involvement on imaging studies are then taken directly to the operating room.
This patient is a 45-year-old marathon runner who works as a professor for a local University. His mother died from lung cancer and his brother was undergoing palliative treatment for stage IV lung cancer. Our patient had no symptoms other than diarrhea, which led to a CT scan of the abdomen and diagnosed the low thoracic tumor (Figure 1) along with other ground glass nodules and part-solid nodules within the lung. He had no other pertinent medical history. His lesions were analyzed by the CANARY System (Figure 2), and the right lower lobe lesion was reported to be in the “poor” risk category, indicating a high likelihood of a diagnosis of invasive adenocarcinoma.
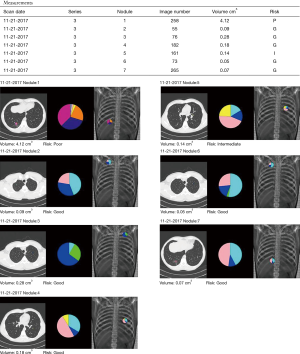
Three-dimensional (3D) printing technology was used to map the tumor and delineate the exact segmental location; identifying the segmental arterial branch, and described the relationship of the pulmonary veins and bronchus in relation to the tumor (Figure 3). With a formal 3D print, the surgeon can manipulate and rotate the model to mimic the operative anatomy and guide the segmentectomy. This technology can also be utilized to inform the patient of the planned procedure and more accurately describe what will happen in a method they better understand.
Once a decision has been made to offer a segmentectomy, the next steps would involve the utilization of shared decision making and obtaining informed consent. Following this, the patient is brought into the operating room whereby an anesthesiologist intubates the patient and places all lines and monitors, including but not limited to arterial line monitoring, large bore peripheral intravenous access, and initially a single lumen endotracheal tube that will later be changed to a double lumen tube for single lung ventilation. The patient typically undergoes a video bronchoscopy to visualize the entire tracheobronchial tree down to the level of the subsegmental bronchi, and, if no abnormalities are found, lung localization of the tumor can be performed with electromagnetic navigational bronchoscopy.
3D imaging and printing
Utilizing current imaging technology and planning software, DICOM images obtained from fine-cut (1.5 mm) CT scans can be utilized to regenerate 3D imaging that may allow localization of the tumor. Contrast enhancement always helps to delineate arterial and vascular structures from one another. 3D reconstruction facilitates identification of the location of the tumor within the segment, any aberrant anatomy, and the location of other pertinent structures. It permits exact mapping of the tumor and resection (Figure 4). At the Mayo Clinic, we have found 3D imaging and printing particularly useful when patients have undergone prior surgery, have aberrant anatomy, or when the location of the tumor is close between two different segments.
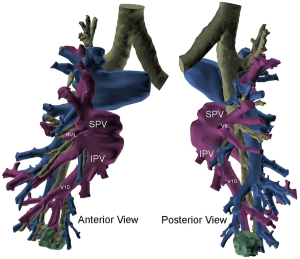
Data should have sufficient spatial resolution to support the desired anatomy to be modeled thus avoiding a stair-step effect in the reconstruction or making. This may also lead to excessive smoothing requirements, which may alter the shape of the anatomy and compromise the integrity of the final model. Images should be acquired with isotropic voxels when available, or efforts to acquire near-isotropic voxels should be taken. When the production of a 3D model is known prior to scan acquisition, slice thickness of the acquired data to be reconstructed should be exported no greater than 2.5 mm, preferably 1.25 mm or less. Images are reconstructed with a thickness compatible with the spatial resolution need for the use of the model generated. In general, what this means is that the smallest anatomy of interest is captured on multiple sequential DICOM images of a particular series. For example, if the anatomy of interest measures 3 mm, it would be desirable for this anatomy to be captured on at least 3 sequential scans, thus the exported slice thickness should be no more than 1 mm. In cases where a 3D model is requested after the volumetric data has been removed from the Radiology System (CT, MRI, etc.), the decision falls on the treatment team to make a judgment if a superior scan is necessary for optimizing outcomes in spite of the additional radiation of another scan.
Image localization
Once the correct segment has been selected and the surgery is planned (which may include utilizing SuperDimension software for mapping the pathway to the tumor), the bronchoscope is guided through a single lumen tube into the patient's tracheobronchial tree and subsequently a catheter is placed through the working port of the bronchoscope. The catheter is then guided into the periphery of the lung (Figure 5). The catheter is centered near the tumor and either a Wang needle is deployed into the tumor or methylene blue dye marker localization is injected directly into the tumor (Figure 6). Fluoroscopy is optional. Air can follow this injection to help disseminate contrast towards the periphery of the lung.
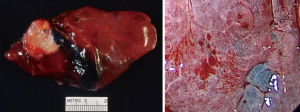
Surgical localization of the tumor and segment
Once the dye marker localization is complete the catheter is withdrawn and the patient is then repositioned. The single-lumen tube is removed and a double-lumen endotracheal tube is placed for single lung ventilation. The patient is then rolled into the left lateral decubitus position when the tumor is on the right side and the arms are tucked and all pressure points are padded. The table is flexed such that the interspaces between the ribs are opened. A sterile prep is applied to the right hemi thorax. The ports are placed as shown in Figures 7A,B. Loco-regional liposomal bupivacaine (Exparel) nerve blocks are infused. The pleural space is then inspected for metastatic disease and lung nodule location (Figure 7B). Once the target lesion is seen, one may begin to dissect into the fissure for an S10 segmentectomy, exposing the basilar segmental branches of the pulmonary artery (Figure 8). The superior segmental and right middle lobar branches are also exposed, and the A10 artery is encircled and occluded while indocyanine green (ICG) is injected into a peripheral or central vein by anesthesia (Figure 9).
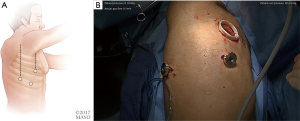
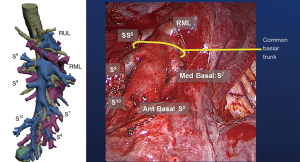
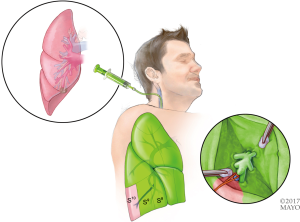
Indocyanine “fox” green was developed by Eastman Kodak specifically for Mayo Clinic in 1957, created by Dr. Irwin J. Fox, who was a research fellow at the time. There are multiple different endoscopic near-infrared (NIR) fluorescence imaging systems available on the market, for example: Novadaq Pinpoint System (Bonita Springs, Florida, USA), Karl Storz IMAGE1 S™ (Tuttlingen, Germany), and Intuitive Firefly systems (Sunnyvale, California, USA). They each essentially require the same volume of ICG injection, and re-dosing is an option. The endoscopic camera systems allow surgeons to visualize perfusion in real-time. By combining a NIR laser with an imaging agent, ICG is injected intravenously and rapidly binds to plasma proteins in the blood. When the NIR laser (scope) is placed to visualize the area of interest, it excites the ICG molecules and they fluoresce. Thus, the systems allow visualized perfusion in real-time imaging. When ICG is injected, it appears on the screen within 10–20 seconds and has a 3-minute half-life, starting to significantly fade from view after 3 minutes. The dye takes 15–20 minutes to fully process out of circulation and reach “baseline” again. The dye is metabolized in the liver and excreted in bile. One should exercise caution/avoid use in patients with an iodide allergy, as ICG has up to 5% sodium iodide.
If the incorrect artery is occluded, the segment surrounding the methylene-blue dye marked tumor will turn green (Figure 10). When the correct artery is occluded, the segments that do not include the blue dye marker will turn green (Figure 11). The tumor marked within the S10 segment along with the blue dye and the parenchyma of that segment will not turn green (Figure 11).
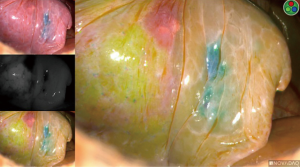
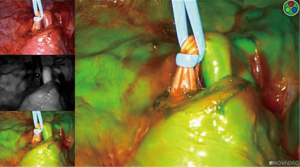
Identification of the pulmonary veins for a segment
Identification of the pulmonary veins draining a segment can be challenging, particularly for V10. When the anatomy is not as clear, we recommend injecting another round of 3 cc of ICG (Figure 12). When utilizing ICG to visualize which veins are without perfusion, the images should be inspected quickly, as once the pulmonary artery has been divided, ICG will no longer travel through the lung and empty into veins, but the left atrium and the primary draining vein may allow some of the ICG to wash back into those veins. Once correctly identified, the veins are either stapled, clipped, or divided. For oncologic purposes, some surgeons advocate taking the pulmonary veins first and then approaching the pulmonary arteries.
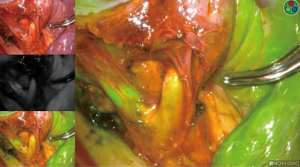
Intersegmental plane
Once ICG has been injected and the pulmonary artery occluded, the intersegmental plane can also be confirmed and marked at this time (Figure 13). Marking can be done with cautery or a felt-tipped marker point placed through one of the ports. Once this confirmatory step has taken place, the pulmonary artery branch that leads to the segment of concern is divided with either a stapling device or silk suture. Bronchoscopy can then be utilized to locate the segmental bronchus based on 3D mapping, and the bronchus leading to the segment housing the tumor is then trans-illuminated (Figure 14). The lung can then be inflated after the stapler is placed around the bronchus and then the bronchus is divided. Once divided, the air will no longer be able to escape from that segment, and the intersegmental plane should be visible both from the ICG marking that was made as well as from the air remaining within the segment while the other segments collapse. These two techniques combined will enhance visualization, sparing unnecessary resection of the segments that do not house the tumor. If the blue dye marker is adjacent to one of the intersegmental planes, then we advise to take a more generous margin, as the goal will be to take a margin that is at least the size of the tumor. The segmental parenchymal division can be performed with energy or a stapler. The advantage of cautery division is the ability to preserve the adjacent veins that lie between the intersegmental plane to preserve the function of the remaining segmental. The advantage of utilizing a stapler is less blood loss and potential quicker discharge as a result of earlier chest tube removal due to shorter air leak. The specimen is then removed in a bag or through a protected port and examined frozen in the pathology lab (Figure 6) to determine the margins, confirmation of pathology, and determine the extent if any of nodal spread. If there is metastasis to regional N1 lymph nodes, a lobectomy should be offered if the patient has adequate pulmonary function. The port sites should be closed to prevent lung herniation through the utility port. A subxiphoid port may be utilized to extract the tumor if only small ports were used during the operation (8). Uniportal incisions are typically generous to always extract a segment. Each segment should be placed into a glove, bag, or delivered through a protected port to prevent port site recurrence (9).
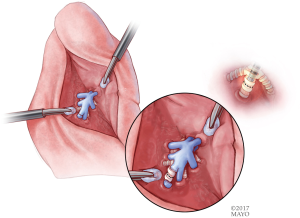
Discussion
The VATS approach can be used for any anatomic segmental resection (10). The most commonly performed segmental resections are lingula sparing left upper lobectomy, lingulectomy, superior segmentectomy, and basilar segmentectomy. On the other hand, a single basilar segmentectomy, such as the S10 segmentectomy described in this paper, is relatively more challenging. With the utilization of several novel technological methods we describe a step by step approach to ICG guided VATS posterior basilar (S10) segmentectomy (Table 1).
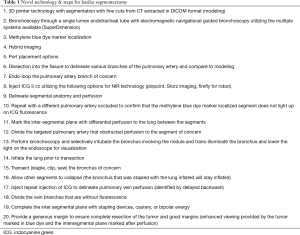
Full table
In general, segmentectomies are technically more challenging than lobectomy, and require deep 3-dimensional knowledge of the relevant bronchoarterial relationships and possible anomalies. The use of 3D imaging and printing, demonstrated in this report, can help the thoracic surgeon in understanding the individual patient’s anatomy in real-time.
After the surgeon becomes familiar with the patient’s anatomy, several localization techniques can be used to accurately guide the surgeon to the location of the targeted lesion intraoperatively. This is especially helpful in patients with ground glass opacities or lesions smaller than 1 cm. In this report, we guide the resection with the use of methylene blue dye injected via SuperDimension navigational bronchoscopy. The combination of methylene blue and ICG provides real time confirmation of the segment to be resected thoracoscopically. Other techniques such as radiotracer injection and wire hook and coil radiopaque markers using intraoperative fluoroscopy can also be used (11-13).
There are multiple ways to identify the intersegmental plane. The traditional method is by occluding the segmental bronchus and inflating the remainder of the lung. Collateral ventilation through Kohn’s pores often fills the isolated segment owing to the positive pressure ventilation, which then fails to correctly outline the inflation/deflation line. Further, in patients with emphysema, expansion of the remaining lung can obscure surgical visualization. Alternatively, Okada and colleagues (14), describe selective jet ventilation of the isolated segment, which allows visualization of the intersegmental plane without losing surgical domain due to expansion of the remaining lung. Misaki and colleagues (15) report using ICG to delineate the plane without ventilation. In this report, we use ICG fluorescence after ligating the segmental pulmonary artery in addition to traditional ventilation to delineate the dissection plane. The use of ICG provides rapid identification, and ventilation confirms the plane which is then marked and dissected with a stapler or energy device.
Although most authors advocate taking the pulmonary veins first, it can be very challenging, particularly for V10. In this report, we injected another round of 3 cc of ICG. When utilizing ICG to visualize which veins are without perfusion, the images should be inspected quickly, as once the pulmonary artery has been divided, ICG will no longer travel through the lung and empty into veins, but the left atrium and the primary draining vein may allow some of the ICG to wash back into those veins. Once correctly identified, the veins are either stapled, clipped, or divided.
In conclusion, the novel techniques described for an individual basilar segmental resection can be utilized to guide a surgeon through a safe and parenchymal-sparing minimally invasive resection.
Acknowledgements
The authors would like to acknowledge Dr. Brian Bartholmai for his work on the CANARY system analysis.
Footnote
Conflicts of Interest: Stapling Technology from Medtronic—royalty paid for stapling technology modifications.
Informed Consent: Written informed consent was obtained from the patient for publication of this manuscript and any accompanying images.
References
- The Surveillance, Epidemiology, and End Results (SEER) Program of the National Cancer Institute. Available online: https://seer.cancer.gov/
- CALGB 140503. A phase III Randomized trial of lobectomy versus sublobar resection for small (≤2 cm) peripheral non-small cell lung cancer. (Last accessed on November 22, 2013). Available online: http://www.calgb.org/Public/meetings/presentations/2007/cra_ws/03-140501-Altorki062007.pdf
- Nakamura K, Saji H, Nakajima R, et al. A phase III randomized trial of lobectomy versus limited resection for small-sized peripheral non-small cell lung cancer (JCOG0802/WJOG4607L). Jpn J Clin Oncol 2010;40:271-4. [Crossref] [PubMed]
- Yang CF, D'Amico TA. Thoracoscopic segmentectomy for lung cancer. Ann Thorac Surg 2012;94:668-81. [Crossref] [PubMed]
- Gossot D, Ramos R, Brian E, et al. A totally thoracoscopic approach for pulmonary anatomic segmentectomies. Interact Cardiovasc Thorac Surg 2011;12:529-32. [Crossref] [PubMed]
- Kent MS, Mandrekar SJ, Landreneau R, et al. A Nomogram to Predict Recurrence and Survival of High-Risk Patients Undergoing Sublobar Resection for Lung Cancer: An Analysis of a Multicenter Prospective Study (ACOSOG Z4032). Ann Thorac Surg 2016;102:239-46. [Crossref] [PubMed]
- Mattioli S, Ruffato A, Puma F, et al. Does anatomical segmentectomy allow an adequate lymph node staging for cT1a non-small cell lung cancer? J Thorac Oncol 2011;6:1537-41. [Crossref] [PubMed]
- Dunning J, Elsaegh M, Nardini M, et al. Microlobectomy: A Novel Form of Endoscopic Lobectomy. Innovations (Phila) 2017;12:247-53. [Crossref] [PubMed]
- McKenna RJ Jr, Houck W, Fuller CB. Video-assisted thoracic surgery lobectomy: experience with 1,100 cases. Ann Thorac Surg 2006;81:421-5; discussion 425-6. [Crossref] [PubMed]
- Yang CJ, Fitch ZW, Balderson SS, et al. Anatomic thoracoscopic segmentectomy for early-stage lung cancer. J Vis Surg 2017;3:123. [Crossref] [PubMed]
- Grogan EL, Stukenborg GJ, Nagji AS, et al. Radiotracer-guided thoracoscopic resection is a cost-effective technique for the evaluation of subcentimeter pulmonary nodules. Ann Thorac Surg 2008;86:934-40; discussion 934-40. [Crossref] [PubMed]
- Finley RJ, Mayo JR, Grant K, et al. Preoperative computed tomography-guided microcoil localization of small peripheral pulmonary nodules: a prospective randomized controlled trial. J Thorac Cardiovasc Surg 2015;149:26-31. [Crossref] [PubMed]
- Nomori H, Horio H, Naruke T, et al. Fluoroscopy-assisted thoracoscopic resection of lung nodules marked with lipiodol. Ann Thorac Surg 2002;74:170-3. [Crossref] [PubMed]
- Okada M, Mimura T, Ikegaki J, et al. A novel video-assisted anatomic segmentectomy technique: selective segmental inflation via bronchofiberoptic jet followed by cautery cutting. J Thorac Cardiovasc Surg 2007;133:753-8. [Crossref] [PubMed]
- Misaki N, Chang SS, Igai H, et al. New clinically applicable method for visualizing adjacent lung segments using an infrared thoracoscopy system. J Thorac Cardiovasc Surg 2010;140:752-6. [Crossref] [PubMed]