Pathophysiology and natural history of atrial septal defect
Introduction
Belonging to one of the most frequent categories of cardiac malformations, atrial septal defects are responsible for a communication between the systemic and the pulmonary circulations. The degree and the direction of the interatrial shunt depend on the size of the defect and the interatrial pressure gradient, related to the relative ventricular compliances. Therefore, when unclosed, a significant atrial septal defect may influence mortality and morbidity.
The objective of this contemporary review is to focus on the pathophysiologic mechanisms underlying the interatrial shunt. The natural history of an isolated atrial septal defect will also be addressed, as it may impact survival, exercise capacity, and favor heart failure, pulmonary hypertension and arrhythmias.
Pathophysiology
Usually, atrial septal defects result in a left-to-right shunt (Figure 1).
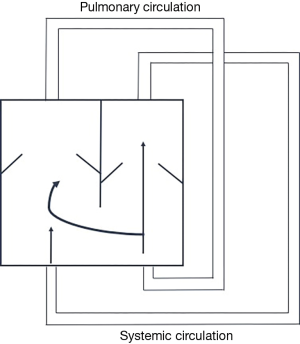
Both direction and magnitude of blood flow through a small atrial communication are determined by the size of the defect and by the relative atrial pressures, which relate to the compliances of the left and right ventricles. In large atrial septal defects, both atrial pressures are equalized and the shunt only depends on the ratio of the ventricular compliances. Brannon and associates first suggested that the relative resistance to filling of the ventricles was the leading factor (1). As demonstrated previously, the defect dimensions, measured by means of transthoracic echocardiography, appear to be well correlated with the pulmonary-to-systemic blood flow ratio evaluated by right heart catheterisation (2).
However, right ventricular compliance is destined to change over time. At birth, pulmonary vascular resistance is high, while right ventricular compliance is low, changing gradually to a high compliance–low resistance circulation. That explains why, left-to-right shunt increases gradually during the first months of life (Figure 2). Therefore, any condition modifying ventricular compliances may impact the degree and direction of the interatrial shunt. Reduction in left ventricular compliance or any condition with elevation of left ventricular filling pressures (e.g., hypertension, ischemic heart disease, cardiomyopathy, aortic and mitral valve disease) will increase the left-to-right shunt. In case of a large defect, systemic venous congestion can occur. Conversely, any condition reducing right ventricular compliance (e.g., pulmonic stenosis, pulmonary hypertension, right ventricular fibrosing process) or tricuspid valve disease may decrease the left-to-right shunt and eventually cause shunt reversal, resulting in cyanosis.
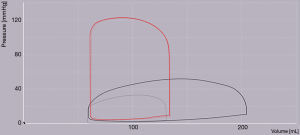
Interestingly, old hemodynamic studies have demonstrated that the left-to-right shunt mostly occurs over an interval encompassing late ventricular systole and early diastole, when both venous systems, both atria, and both ventricles are in free communication (3). The shunt also increases during atrial contraction. Respiration influences the shunt, as during periods with increased intrathoracic pressures, the left-to-right gradient increases and during periods with decreased intrathoracic pressures, there is a fall in the left-to-right gradient. Minute right-to-left shunting has been observed with the onset of ventricular contraction, and during early ventricular diastole (3), without any adverse clinical significance.
In normal conditions, high right ventricular compliance explains that atrial septal defects result more often in a left-to-right shunt through the defect. The left-to-right shunt is responsible for right ventricular volume overload and pulmonary overcirculation. Relevant ventricular volume overload is usually observed when the size of the defect is greater than 10 mm (4). Smaller defects, although responsible for a certain degree of shunting, do not result in significant right ventricular volume overload. For larger shunts, right volume overload gives rise to enlargement of right-sided cavities. This results in altered left ventricular geometry, with the “reverse Bernheim’s effect”, proposed by Dexter et al., in which the septum bulges into and encroaches on the left ventricular cavity (5). In transthoracic echocardiography, a diastolic flattening of the interventricular septum is noticed, with an end-diastolic D-shaped left ventricle (when atrioventricular valves are opened and pressures are equal in both atria and ventricles) and a circular shape in systole (when atrioventricular valves are closed and septal geometry reflects the ratio between left and right systolic ventricular pressures) (6,7). Similar data were reported for all cardiac situations resulting in right ventricular volume overload; e.g., tricuspid regurgitation, pulmonary regurgitation (8). Therefore, the interventricular interdependance gets impaired, and the left ventricle ultimately underfilled and less distensible (compressed by the dilated right ventricle) (9), with a redirection of left atrial flow to the right atrium through the atrial septal defect, and eventually a reduction in cardiac output. Indeed, diminished left ventricular filling capacity has been suggested by the fact that left ventricular end-diastolic pressure is often comparable to or greater than that of normal subjects despite reduced left ventricular end-diastolic volume because of the atrial left-to-right shunt. This was clearly shown in 2 previous studies that demonstrated a leftward and upward shift of diastolic pressure-volume relationship for patients with an atrial septal defect, compared to controls (10,11). Nevertheless, the left ventricular diastolic pressure-volume relationship is influenced by several factors, including intrinsic diastolic stiffness, effects of external constraint, relaxation, and ongoing filling characteristics (12). External constraint and ongoing filling characteristics get reduced or normalised after suppression of the interatrial shunt. Therefore, only the 2 other factors, left ventricular diastolic stiffness and left ventricular abnormal relaxation, may be responsible for acute and most often transient heart failure after the shunt closure (13). In children, study of the left ventricular end-diastolic pressure-volume relationship showed that decrease in filling capacity was not attributed to increased left ventricular passive stiffness (14). Aging is associated with left ventricular stiffness, which increases the risk of left heart failure when closing the defect (15). Prolonged relaxation time has been observed both in children and adults with atrial septal defects, but only in patients with large left-to-right shunts (11), highlighting the ventricular interdependence.
Lower left ventricular performance has been reported, in case of right ventricular volume overload, due to reduced chamber preload (demonstrated by reduced left ventricular end-diastolic volume), reduced myofiber stretching and consequently reduced left ventricular stroke volume, according to the Starling’s law (7).
A longstanding haemodynamically significant shunt leads to chronic right-sided cavities volume overload, and then to myocardial injury, as demonstrated by higher highly sensitive cardiac troponin I values in patients with atrial septal defects compared to matched controls (16). Right-sided cavities enlargement increases the myocardial oxygen demand, which results in relative hypoperfusion of the myocardium. Higher levels of angiotensine II and catecholamines, inducing necrosis and apoptosis of myocardial cells, may play a role in myocardial injury. The amino-terminal procollagen type III peptide is a circulating blood marker, reflecting collagen synthesis in the tissues. Its concentration is increased in patients with heart failure. Its level has been demonstrated to correlate with the pulmonary-to-systemic blood flow ratio in patients with hemodynamically significant atrial septal defects (17). As a marker of myocardial remodeling, high levels of procollagen type III N-terminal peptide may be associated with an increase in myocardial stiffness and ultimately, with diastolic heart failure. Moreover, Poiseuille’s law demonstrates that the chronic increase in flow through pulmonary arteries leads to chronic increase in pressure. A systematic histologic study of perioperative lung biopsies in adults with surgically atrial septal defect closure revealed a considerable proportion of hypertensive pulmonary vascular remodeling, with no correlation with pre-operative data, including hemodynamic data (18).
Natural history
The availability of surgical closure of atrial septal defects since 1952 hampers the evaluation of its natural history in the modern era. Nevertheless, historical series have well demonstrated decreased life expectancy and expected long-term complications (Figure 3), depending on the size and location of the defect, the intensity of the shunt and specific individual factors.
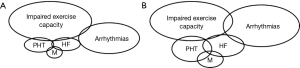
Life expectancy
Significant atrial septal defects are associated with increased morbidity and mortality, as demonstrated by old studies, highlighting the rising mortality with age. Campbell reported a low annualised mortality rate in the first two decades of life (0.6% and 0.7% per year, respectively), increasing to 4.5% per year in the fourth decade and 7.5% per year in the sixth decade, and related to pulmonary hypertension and congestive heart failure (19). These dramatic data seem to exaggerate the poor outcome, as many unrepaired patients remain remarkably well and symptom-free through early adulthood. A subsequent study from Murphy et al., particularly studied long-term outcome of 123 adults with surgical repair of ostium secundum or sinus venosus atrial septal defects. When operated on before 25 years of age, survival was similar to that of a matched population. In contrast, those operated on later in life experienced higher mortality and morbidity. Independent predictors of long-term survival were age at operation and systolic pulmonary arterial pressure before surgery. Twenty seven late deaths were reported, with 18 from cardiovascular cause (13 cardiac and 5 stroke deaths) (20). Doty et al. reported premature death due to progressive right ventricular dilation with diminished coronary reserve (21).
Size of the defect
Previous studies have shown that two-dimensional echocardiographic dimensions of the atrial septal defect are well correlated with the actual anatomic size of the defect measured during surgery (22).
The natural history of atrial septal defects varies according to the anatomic form. In ostium secundum defects, spontaneous closure is not uncommon. In a large retrospective series including 200 consecutive children, followed-up during 6 months, 34% of the defects closed spontaneously and 28% decreased to a diameter of 3 mm or less. Initial atrial septal defect diameter was the main predictor of spontaneous closure (23). An Iranian study concluded later that atrial septal defects of less than 6 mm typically close spontaneously, and those between 6 and 9 mm may regress in infants and children. When over 1 cm, the probability of spontaneous closure becomes poor (24,25). Based on these similar observations, Radzik et al. suggest that infants born with an atrial septal defect of less than 3 mm do not require cardiac follow-up because spontaneous closure will occur in all, considering it may not constitute a cardiac malformation (26). Conversely, for atrial septal defects larger than 8 mm, families should be informed of the possible need for interventional closure later in life, either percutaneously or surgically, therefore requiring a regular echocardiographic control. Several mechanisms have been advocated to explain spontaneous closure: downward growth of the septum secundum (27), thrombotic plug formation (28), septal aneurysm formation (29,30), fusion of valve like openings (31).
For the ostium secundum defects that do not spontaneously close, the diameter can change overtime. One hundred four patients, mean aged 4.5 years, were examined in the Texas Children’s Hospital, during a mean follow-up period of 3 years. At diagnosis, 33% of the defects were small (between at least 3 and 6 mm), 38% were moderate (between at least 6 and 12 mm), and 29% were large (12 mm and more). Among all, atrial septal defect diameters increased in 68 of 104 patients (65%), including 31 patients (30%) with an increase of at least 50%. Thirteen defects (12%) increased to more than 20 mm. The only independent factor related to growth was the initial size of the defect. Larger defects were more likely to larger growth. Neither the age at diagnosis nor the body surface area was related to the septal defect growth (32).
Considering ostium secundum atrial septal defects, the indication for closure of a hemodynamically significant shunt is based on the pulmonary-to-systemic blood flow ratio (Qp/Qs), when evidence of right-sided cavities overload (i.e., superior to 1.5) (33). There is no cut-off value considering the size of the defect.
Clinical presentation
Patients with an isolated atrial septal defect commonly remain asymptomatic during childhood and adolescence (19). Even those with a large left-to-right atrial shunt may not have overt symptoms until adulthood. Incidental diagnosis by an echocardiogram performed because of a heart murmur or an abnormal finding on a chest radiogram or an electrocardiogram (i.e., rSr’ or rsR’ QRS configuration in right precordial leads) is common (34). Pregnancy may sometimes lead to the diagnosis in case of a well heard heart murmur.
Rarely, they may cause symptoms in infancy, as failure to thrive, tachypnea, recurrent respiratory infections, heart failure, and respiratory insufficiency necessitating artificial ventilation (35). Lammers et al., retrospectively reported the outcome of 24 infants younger than 1 year of age, who underwent a surgical closure of isolated atrial septal defects for symptomatic shunts. Most of them had either pulmonary hypertension from other reason (i.e., diaphragmatic hernia, chronic lung disease subsequent to prematurity), or non-cardiac anomalies (i.e., dysmorphic syndromes), or both. The authors observed a significant improvement of clinical performance, growth, and development in nearly all patients after 46±33 months of follow-up, after surgical atrial shunt closure. All ventilator-dependent children could be weaned shortly after the defect closure (36). Nevertheless, the question should be discussed as to whether surgery is of benefit in these children, and particularly, how pulmonary vascular resistances may evolve.
Formally, adult patients will become symptomatic from the third or fourth decade (19). In the absence of any arrhythmias or pulmonary hypertension, the right-sided cavities overload and the pulmonary overcirculation can lead to subtle symptoms, including shortness of breath with exertion and the physical feeling of palpitations. By contrast, most adults with large shunts will experience fatigue, exercise intolerance, palpitations, syncope, and shortness of breath. Ultimately, they will suffer from right-sided heart failure, with peripheral edema, cyanosis in case of Eisenmenger syndrome, or even manifestations of thromboembolism with paradoxical emboli (37).
Exercise capacity
Exercise capacity is a matter of concern when considering atrial septal defect closure, as one of the objectives of removing the shunt may be to improve functional abilities. A study from Rhodes et al. (38) included 9 consecutive pediatric patients with significantly hemodynamic inter-atrial shunts, without pulmonary hypertension, and referred for transcatheter closure. All underwent bicycle ergometric exercise test 0 to 2 months before the procedure and 1 to 7 months after. No significant difference was observed in parameters before and after the suppression of the shunt. Exercise capacity appeared to be well preserved and did not improve following transcatheter closure. As the individual’s exercise function is determined by a complex interaction between circulatory, respiratory, and musculoskeletal systems (39); an improvement solely in a component of the circulatory system (e.g., by closing an atrial septal defect) may therefore have little effect upon exercise function unless ultimately accompanied by improvements in other systems as well, such as through exercise training or a rehabilitation program.
The prevalence of exercise intolerance increases insidiously with age. In a cohort of 17 adults with atrial septal defects compared to age- and gender-matched controls, a lower peak oxygen uptake was noticed, as well as a lower ventilatory efficiency (40). Simultaneously, perceived health-status evaluated by the SF-36 questionnaire, was lower in patients than in controls. The best independent predictors of functional limitations appeared to be pulmonary hypertension and right ventricular volume overload in a multivariable model of adult patients from the Euro Heart Survey database (41).
Pulmonary function was studied among 55 children undergoing atrial septal defect percutaneous closure: pulmonary function tests were impaired before the shunt closure, particularly for peak expiratory flow and in the peripheral airway. Improved pulmonary outcome was noticed after closure, and all the more so when no pulmonary hypertension preexisted (42).
These observations would encourage closing atrial septal defects, to prevent alterations in exercise capacity and pulmonary function. Nonetheless, a study from Santos et al. revealed the persistence of an impaired exercise capacity in a subset of asymptomatic adult patients, with normal resting hemodynamic parameters, having previously undergone shunt closure (43). Despite similar resting hemodynamic variables, patients with impaired peak oxygen uptake had a lower peak cardiac index due to abnormal stroke volume augmentation and an abnormal total pulmonary vascular resistance change during exercise, raising the hypothesis of a combination of abnormal pulmonary vascular response to exercise and impaired right ventricular function (43).
Pulmonary hypertension
Pulmonary arterial hypertension may develop in response to a chronic volume overload of the pulmonary circulation, caused by a left-to-right shunt. According to the Poiseuille’s law, volume overload will lead to an increase in right-sided cardiac output, and therefore, to an increase in pulmonary pressures. Chronic volume overload of the pulmonary circulation is also thought to cause irreversible changes of the medium-sized and small arteries (18,44), leading to pulmonary vascular remodeling. It is an important complication in patients with hemodynamically relevant atrial septal defects as it negatively affects outcome. Its prevalence in this population has been estimated around 10% (45).
Different clinical pulmonary arterial hypertension scenarios can be encountered, despite similar volume overload patterns. At one end of the spectrum, there are adults with no or only mild pulmonary vascular disease and a large shunt. These are patients who can safely undergo shunt closure. At the other end of the spectrum, there are adults with severe, irreversible pulmonary vascular disease, shunt reversal and chronic cyanosis, that is, Eisenmenger syndrome (46). In some patients, the progression rate is much faster (47). This suggests a multifactorial cause of pulmonary vascular disease, with a likely role for genetic factors, as in idiopathic pulmonary arterial hypertension, still largely underknown so far. Contributing factors such as Down syndrome, upper airway obstruction, pulmonary emboli or in-situ pulmonary artery thrombosis, and drug use such as anorexigenic agents should be carefully explored, especially in patients with fairly small defects.
Subsequently, pulmonary hypertension is uncommon in children with an isolated atrial septal defect, except for those living in high altitude (48). In adults, the prevalence of patients with pulmonary hypertension has been estimated to 34%, among all patients with open atrial septal defects, including those with Eisenmenger syndrome, from the database of the Euro Heart Survey (49). Median age was 15 years higher in patients with pulmonary hypertension compared to those without, and in multivariate analysis, the probability of pulmonary hypertension significantly increased with a factor of 1.041 for each extra year of age. Along with the longstanding volume overload, it cannot be excluded that the increase in prevalence with age is related to the process of aging in itself. A larger size of the defect has also been shown to be related to higher levels of pulmonary pressure (50).
The presence of pulmonary hypertension was strongly associated with clinical symptoms, with reduction in exercise capacity: a strong correlation was demonstrated between the level of pulmonary hypertension and NYHA functional class. As a consequence, the probability of right ventricular dysfunction increased with the level of pulmonary pressures (49), although clinical signs of right heart failure are rather uncommon (51). A retrospective echocardiographic study of 92 adults with atrial septal defects revealed that, in multivariable logistic regression, systolic pulmonary arterial pressure was the variable that most often predicted right ventricular systolic dysfunction as evidenced by TAPSE of less than 17 mm and right ventricular fractional shortening area of less than 35%. The high Qp/Qs (reflecting fluid overload) and right-sided tissue Doppler S <9.5 cm/s (strongly dependent on age and therefore, on the time course of the disease) most accurately predicted a Tei index >0.55 (50). Due to the correlation between pulmonary vascular changes and pulmonary hemodynamics, cardiac catheterization is useful for diagnosis, adding further information to echocardiography in the decision-making process (46), before its therapeutic role. A specific chapter is dedicated to the hemodynamic assessment of unoperated atrial septal defects.
Ultimately, Eisenmenger syndrome occurs, with right-to-left atrial-level shunting, accounting for 5% to 10% of all causes of pulmonary arterial hypertension. Total pulmonary vascular resistance and arterial oxygen saturation therefore influence the outcome (52). Eisenmenger syndrome is a contra-indication for shunt closure, as it would contribute to a high risk of acute right ventricular failure and the prognosis of patients with a persistent shunt is better than that of patients without any shunt (33). As the interatrial right-to-left shunt only relieves diastolic right ventricular pressure, patients with pre-tricuspid shunts have a worst prognosis than patients with Eisenmenger syndrome and post-tricuspid shunt (e.g., ventricular septal defect or patent ductus arteriosus). Few case reports have reported favorable outcome of adults with ostium secundum or sinus venosus atrial septal defects and severe pulmonary hypertension, with a beneficial role of vasodilator therapy until stabilization or even reversal of the shunt (53,54).
Right-sided heart failure
The occurrence of right heart failure in patients with unclosed atrial septal defects appears to be independent from the presence of pulmonary hypertension, due to right-sided cavities chronic volume overload. Nevertheless, pulmonary hypertension appears to be an aggravating factor.
Pulmonary overcirculation may also increase the risk of pneumonia. There is no data about its incidence. Usually, this complication occurs from the fourth or fifth decade.
Palpitations/arrhythmias
Up to 33.8% of adults with unrepaired atrial septal defects complain of palpitations (55), which can often be attributed to sinus tachycardia, reversible with the suppression of the shunt. Overall, the rhythm is most often sinus among patients with open atrial septal defects. New-onset electrocardiographic anomalies can help diagnosing atrial septal defects. An rSr’ or rsR’ QRS configuration is typically seen on the ECG in right precordial leads, likely reflecting right ventricular overload (56). In a retrospective pediatric study including 141 children with atrial septal defects, the development of an rSR’ pattern had an incremental value in predicting the need for surgical or device intervention for closure in both moderate and large shunt groups (57). A complete right bundle-branch block pattern is not infrequent with increasing age (34). In inferior leads, a notch near the apex of the R wave, coined “crochetage”, has been correlated with atrial septal defect. Its incidence was higher in patients with larger defects and greater left-to-right shunting (58). Some authors suggest that the co-existence of right bundle branch block and isolated negative T wave in the precordial leads is well correlated with hemodynamically significant atrial shunts (59).
Major arrhythmias are uncommon in children with atrial septal defects. In a study from Berger et al., 211 adults were examined from an arrhythmic point of view, before and after atrial shunt closure: before surgery, only 1% of patients younger than 40 years old experienced atrial flutter, against 15% between 40 and 60 years old. No difference was noticed according to the anatomic location of the defect (60). Atrial tachyarrhythmia or bradyarrhythmia in older patients is the byproduct of longstanding right atrial dilation and stretch, which may be sustained by tricuspid regurgitation, pulmonary arterial hypertension, right ventricular failure. A recent study from Labombarda et al. confirmed the hypothesis, among adults with various congenital heart defects, that intra-atrial reentrant tachycardia remains the most common presenting atrial arrhythmia, whatever the complexity of the congenital heart disease, with a predominantly paroxysmal pattern. Atrial fibrillation increases in prevalence and atrial arrhythmias progressively become permanent as the population ages (61).
Atrial arrhythmias may also be associated by a higher incidence of stroke and paradoxical emboli (62).
Several mutations in NKX2.5 gene have been reported, linked to atrial septal defect and atrioventricular block, and are not uncommon (63).
Conclusions
Atrial septal defects allow communication between the systemic and the pulmonary circulations. The nature of the shunt, including direction and magnitude of blood flow, depends on both the size of the defect and the relative atrial pressures, which relate to the compliances of the left and right ventricles. Any hemodynamic condition modifying the ventricular compliances or intracardiac pressures will impact the interatrial shunt. A longstanding significant interatrial shunt will lead to a chronic right-sided cavities overload, and therefore will impact survival and morbidity. The natural history of atrial septal defects, although less a matter of interest since the development of surgical and percutaneous closure, remains relevant. Significant interatrial shunting may decrease exercise capacity, favor atrial arrhythmias, contribute to the development of heart failure and pulmonary hypertension, and ultimately impact survival.
Acknowledgements
None.
Footnote
Conflicts of Interest: The authors have no conflicts of interest to declare.
References
- Brannon ES, Warren JV. The use of right heart catheterization in the diagnosis of atrial septal defect. Proc Am Fed Clin Res 1945;2:13. [PubMed]
- Fuse S, Tomita H, Hatakeyama K, et al. Effect of size of a secundum atrial septal defect on shunt volume. Am J Cardiol 2001;88:1447-50, A9.
- Levin AR, Spach MS, Boineau JP, et al. Atrial pressure-flow dynamics in atrial septal defects (secundum type). Circulation 1968;37:476-88. [Crossref] [PubMed]
- Geva T, Martins JD, Wald RM. Atrial septal defects. Lancet 2014;383:1921-32. [Crossref] [PubMed]
- Dexter L. Atrial septal defect. Br Heart J 1956;18:209-25. [Crossref] [PubMed]
- Weyman AE, Wann S, Feigenbaum H, et al. Mechanism of abnormal septal motion in patients with right ventricular volume overload: a cross-sectional echocardiographic study. Circulation 1976;54:179-86. [Crossref] [PubMed]
- Walker RE, Moran AM, Gauvreau K, et al. Evidence of adverse ventricular interdependence in patients with atrial septal defects. Am J Cardiol 2004;93:1374-7, A6.
- Louie EK, Bieniarz T, Moore AM, et al. Reduced atrial contribution to left ventricular filling in patients with severe tricuspid regurgitation after tricuspid valvulectomy: a Doppler echocardiographic study. J Am Coll Cardiol 1990;16:1617-24. [Crossref] [PubMed]
- Popio KA, Gorlin R, Teichholz LE, et al. Abnormalities of left ventricular function and geometry in adults with an atrial septal defect. Ventriculographic, hemodynamic and echocardiographic studies. Am J Cardiol 1975;36:302-8. [Crossref] [PubMed]
- Booth DC, Wisenbaugh T, Smith M, et al. Left ventricular distensibility and passive elastic stiffness in atrial septal defect. J Am Coll Cardiol 1988;12:1231-6. [Crossref] [PubMed]
- Satoh A, Katayama K, Hiro T, et al. Effect of right ventricular volume overload on left ventricular diastolic function in patients with atrial septal defect. Jpn Circ J 1996;60:758-66. [Crossref] [PubMed]
- Burkhoff D, Mirsky I, Suga H. Assessment of systolic and diastolic ventricular properties via pressure-volume analysis: a guide for clinical, translational, and basic researchers. Am J Physiol Heart Circ Physiol 2005;289:H501-12. [Crossref] [PubMed]
- Masutani S, Senzaki H. Left ventricular function in adult patients with atrial septal defect: implication for development of heart failure after transcatheter closure. J Card Fail 2011;17:957-63. [Crossref] [PubMed]
- Senzaki H, Chen CH, Masutani S, et al. Assessment of cardiovascular dynamics by pressure-area relations in pediatric patients with congenital heart disease. J Thorac Cardiovasc Surg 2001;122:535-47. [Crossref] [PubMed]
- Redfield MM, Jacobsen SJ, Borlaug BA, et al. Age- and gender-related ventricular-vascular stiffening: a community-based study. Circulation 2005;112:2254-62. [Crossref] [PubMed]
- Sugimoto M, Ota K, Kajihama A, et al. Volume overload and pressure overload due to left-to-right shunt-induced myocardial injury. - Evaluation using a highly sensitive cardiac Troponin-I assay in children with congenital heart disease. Circ J 2011;75:2213-9. [Crossref] [PubMed]
- Sugimoto M, Masutani S, Seki M, et al. High serum levels of procollagen type III N-terminal amino peptide in patients with congenital heart disease. Heart 2009;95:2023-8. [Crossref] [PubMed]
- Sachweh JS, Daebritz SH, Hermanns B, et al. Hypertensive pulmonary vascular disease in adults with secundum or sinus venosus atrial septal defect. Ann Thorac Surg 2006;81:207-13. [Crossref] [PubMed]
- Campbell M. Natural history of atrial septal defect. Br Heart J 1970;32:820-6. [Crossref] [PubMed]
- Murphy JG, Gersh BJ, McGoon MD, et al. Long-term outcome after surgical repair of isolated atrial septal defect. Follow-up at 27 to 32 years. N Engl J Med 1990;323:1645-50. [Crossref] [PubMed]
- Doty DB, Wright CB, Hiratzka LF, et al. Coronary reserve in volume-induced right ventricular hypertrophy from atrial septal defect. Am J Cardiol 1984;54:1059-63. [Crossref] [PubMed]
- Chen C, Kremer P, Schroeder E, et al. Usefulness of anatomic parameters derived from two-dimensional echocardiography for estimating magnitude of left to right shunt in patients with atrial septal defect. Clin Cardiol 1987;10:316-21. [Crossref] [PubMed]
- Hanslik A, Pospisil U, Salzer-Muhar U, et al. Predictors of spontaneous closure of isolated secundum atrial septal defect in children: a longitudinal study. Pediatrics 2006;118:1560-5. [Crossref] [PubMed]
- Behjati-Ardakani M, Golshan M, Akhavan-Karbasi S, et al. The Clinical Course of Patients With Atrial Septal Defects. Iran J Pediatr 2016;26. [Crossref] [PubMed]
- Saxena A, Divekar A, Soni NR. Natural history of secundum atrial septal defect revisited in the era of transcatheter closure. Indian Heart J 2005;57:35-8. [PubMed]
- Radzik D, Davignon A, van Doesburg N, et al. Predictive factors for spontaneous closure of atrial septal defects diagnosed in the first 3 months of life. J Am Coll Cardiol 1993;22:851-3. [Crossref] [PubMed]
- Cayler GG. Spontaneous functional closure of symptomatic atrial septal defects. N Engl J Med 1967;276:65-73. [Crossref] [PubMed]
- Sherman FS, Sahn DJ, Valdes-Cruz LM, et al. Two-dimensional Doppler color flow mapping for detecting atrial and ventricular septal defects. Studies in an animal model and in the clinical setting. Herz 1987;12:212-6. [PubMed]
- Timmis GC, Gordon S, Reed JO. Spontaneous closure of an atrial septal defect. JAMA 1966;196:17-9. [Crossref] [PubMed]
- Awan IH, Rice R, Moodie DS. Spontaneous closure of atrial septal defect with interatrial aneurysm formation. Documentation by noninvasive studies, including digital subtraction angiography. Pediatr Cardiol 1982;3:143-5. [Crossref] [PubMed]
- Fukazawa M, Fukushige J, Ueda K. Atrial septal defects in neonates with reference to spontaneous closure. Am Heart J 1988;116:123-7. [Crossref] [PubMed]
- McMahon CJ, Feltes TF, Fraley JK, et al. Natural history of growth of secundum atrial septal defects and implications for transcatheter closure. Heart 2002;87:256-9. [Crossref] [PubMed]
- Baumgartner H, Bonhoeffer P, De Groot NM, et al. ESC Guidelines for the management of grown-up congenital heart disease (new version 2010). Eur Heart J 2010;31:2915-57. [Crossref] [PubMed]
- Khairy P, Marelli AJ. Clinical use of electrocardiography in adults with congenital heart disease. Circulation 2007;116:2734-46. [Crossref] [PubMed]
- Andrews R, Tulloh R, Magee A, et al. Atrial septal defect with failure to thrive in infancy: hidden pulmonary vascular disease? Pediatr Cardiol 2002;23:528-30. [Crossref] [PubMed]
- Lammers A, Hager A, Eicken A, et al. Need for closure of secundum atrial septal defect in infancy. J Thorac Cardiovasc Surg 2005;129:1353-7. [Crossref] [PubMed]
- Lok SI, Winkens B, Dimopoulos K, et al. Recurrence of cerebrovascular events in young adults with a secundum atrial septal defect. Int J Cardiol 2010;142:44-9. [Crossref] [PubMed]
- Rhodes J, Patel H, Hijazi ZM. Effect of transcatheter closure of atrial septal defect on the cardiopulmonary response to exercise. Am J Cardiol 2002;90:803-6. [Crossref] [PubMed]
- Wasserman K. The Dickinson W. Richards lecture. New concepts in assessing cardiovascular function. Circulation 1988;78:1060-71. [Crossref] [PubMed]
- Van De Bruaene A, Buys R, Vanhees L, et al. Cardiopulmonary exercise testing and SF-36 in patients with atrial septal defect type secundum. J Cardiopulm Rehabil Prev 2011;31:308-15. [Crossref] [PubMed]
- Engelfriet P, Meijboom F, Boersma E, et al. Repaired and open atrial septal defects type II in adulthood: an epidemiological study of a large European cohort. Int J Cardiol 2008;126:379-85. [Crossref] [PubMed]
- Lee YS, Jeng MJ, Tsao PC, et al. Pulmonary function changes in children after transcatheter closure of atrial septal defect. Pediatr Pulmonol 2009;44:1025-32. [Crossref] [PubMed]
- Santos M, Systrom D, Epstein SE, et al. Impaired exercise capacity following atrial septal defect closure: an invasive study of the right heart and pulmonary circulation. Pulm Circ 2014;4:630-7. [Crossref] [PubMed]
- Yamaki S, Horiuchi T, Miura M, et al. Pulmonary vascular disease in secundum atrial septal defect with pulmonary hypertension. Chest 1986;89:694-8. [Crossref] [PubMed]
- Duffels MG, Engelfriet PM, Berger RM, et al. Pulmonary arterial hypertension in congenital heart disease: an epidemiologic perspective from a Dutch registry. Int J Cardiol 2007;120:198-204. [Crossref] [PubMed]
- Schwerzmann M, Pfammatter JP. Approaching atrial septal defects in pulmonary hypertension. Expert Rev Cardiovasc Ther 2015;13:693-701. [Crossref] [PubMed]
- Balint OH, Samman A, Haberer K, et al. Outcomes in patients with pulmonary hypertension undergoing percutaneous atrial septal defect closure. Heart 2008;94:1189-93. [Crossref] [PubMed]
- Khoury GH, Hawes CR. Atrial septal defect associated with pulmonary hypertension in children living at high altitude. J Pediatr 1967;70:432-5. [Crossref] [PubMed]
- Engelfriet PM, Duffels MG, Moller T, et al. Pulmonary arterial hypertension in adults born with a heart septal defect: the Euro Heart Survey on adult congenital heart disease. Heart 2007;93:682-7. [Crossref] [PubMed]
- Cossio-Aranda J, Zamora KD, Nanda NC, et al. Echocardiographic correlates of severe pulmonary hypertension in adult patients with ostium secundum atrial septal defect. Echocardiography 2016;33:1891-6. [Crossref] [PubMed]
- Craig RJ, Selzer A. Natural history and prognosis of atrial septal defect. Circulation 1968;37:805-15. [Crossref] [PubMed]
- Steele PM, Fuster V, Cohen M, et al. Isolated atrial septal defect with pulmonary vascular obstructive disease--long-term follow-up and prediction of outcome after surgical correction. Circulation 1987;76:1037-42. [Crossref] [PubMed]
- Anuwatworn A, Gedela M, Bendaly E, et al. Sinus Venosus Atrial Septal Defect Complicated by Eisenmenger Syndrome and the Role of Vasodilator Therapy. Case Rep Cardiol 2016;2016. [Crossref] [PubMed]
- Dudzinski DM, Bhatt DL, Aragam JR, et al. Explaining unexplained dyspnea: the ever "holesome" heart. Circulation 2014;130:2057-66. [Crossref] [PubMed]
- Motiwala A, Fatimi SH, Akhtar N, et al. Patients with congenital atrial septal defects: effect of age at repair and defect size on pulmonary artery pressures prior to repair. Thorac Cardiovasc Surg 2011;59:281-6. [Crossref] [PubMed]
- Sung RJ, Tamer DM, Agha AS, et al. Etiology of the electrocardiographic pattern of "incomplete right bundle branch block" in atrial septal defect: an electrophysiologic study. J Pediatr 1975;87:1182-6. [Crossref] [PubMed]
- Refaei M, Islam S, Mackie AS, et al. Correlation of electrocardiogram parameters and hemodynamic outcomes in patients with isolated secundum atrial septal defects. Ann Pediatr Cardiol 2017;10:152-7. [Crossref] [PubMed]
- Rodriguez-Alvarez A, Martinez De Rodriguez G, Goggans AM, et al. The vectorcardiographic equivalent of the "crochetage" of the QRS of the electrocardiogram in atrial septal defect of the ostium secundum type. Preliminary report. Am Heart J 1959;58:388-94. [Crossref] [PubMed]
- Somura J, Nakagawa M, Ukiami M, et al. Relationship between electrocardiographic signs and shunt volume in atrial septal defect. Pediatr Int 2015;57:535-40. [Crossref] [PubMed]
- Berger F, Vogel M, Kramer A, et al. Incidence of atrial flutter/fibrillation in adults with atrial septal defect before and after surgery. Ann Thorac Surg 1999;68:75-8. [Crossref] [PubMed]
- Labombarda F, Hamilton R, Shohoudi A, et al. Increasing Prevalence of Atrial Fibrillation and Permanent Atrial Arrhythmias in Congenital Heart Disease. J Am Coll Cardiol 2017;70:857-65. [Crossref] [PubMed]
- Attie F, Rosas M, Granados N, et al. Surgical treatment for secundum atrial septal defects in patients >40 years old. A randomized clinical trial. J Am Coll Cardiol 2001;38:2035-42. [Crossref] [PubMed]
- Xu YJ, Qiu XB, Yuan F, et al. Prevalence and spectrum of NKX2.5 mutations in patients with congenital atrial septal defect and atrioventricular block. Mol Med Rep 2017;15:2247-54. [Crossref] [PubMed]