Fiducial marker placement for stereotactic body radiation therapy via convex probe endobronchial ultrasound: a case series and review of literature
Introduction
Convex probe endobronchial ultrasound (CP-EBUS) and stereotactic body radiotherapy (SBRT) have revolutionized the diagnosis, staging, and treatment of lung cancer. CP-EBUS facilitates safe, accurate, and efficient sampling of intrathoracic lymph nodes (LNs) and lesions, while simultaneously staging the mediastinum and hilum (1-7). SBRT is an attractive treatment strategy for early-stage lung cancer, especially when patients are inoperable, and in intrathoracic oligo-metastatic disease (8-15). Due to respiratory movement of thoracic structures, SBRT frequently requires intralesional placement of radio-opaque fiducial markers (FMs) as reference points to ensure precise delivery of radiation (16-20). We present a case series of successfully employing a technique of using CP-EBUS to place FMs in centrally located malignant lesions of the thorax, review the literature surrounding similar techniques, and discuss the billing and coding considerations with this approach.
Patients
Eight patients were referred for FM placement to the interventional pulmonology service to guide SBRT for their known or suspected malignancies. In all cases, SBRT was considered the optimal treatment approach once malignancy was proven due to age, comorbidity, previous treatment, or preference (Table 1).
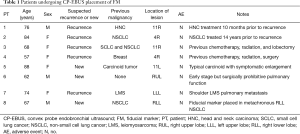
Full table
Procedural technique
Procedures were performed in the bronchoscopy suite with anesthesia by moderate sedation or monitored anesthesia care (MAC).
CP-EBUS was utilized to deploy FM into the lesion of interest under real-time ultrasound visualization by the following method:
- The lesion was located and sampled via CP-EBUS using a 7.5 MHz EBUS bronchoscope and dedicated 21-G needle (Olympus BF-UC180F and ViziShot needle, Olympus America, Center Valley, PA, USA).
- The needle was withdrawn, and rapid on-site evaluation (ROSE) of biopsy material was utilized to diagnose and confirm target.
- A 0.5 mm × 0.75 mm visicoil FM was then opened and modified by straightening the coil into a wire and then dividing it into shorter segments using scissors. The internal stylet was partially pulled back, and pieces were back-loaded into the tip of the needle and the tip sealed with sterile bone wax to prevent dislodgement (Figure 1A,B,C,D).
- The lesion was then relocated via CP-EBUS and punctured with the FM loaded needle. The stylet was advanced into the needle deploying the FM, under real-time imaging, into the lesion. (Figure 2A).
- Steps 3 and 4 were repeated 3–5 times resulting in the placement of 3–5 modified FMs in the target lesion of interest.
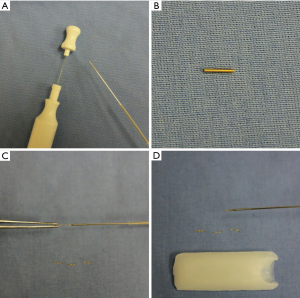
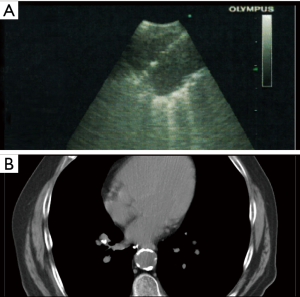
We describe two representative cases with the complete list of patients presented in Table 1.
Case 1
A 76-year-old male with a history of oropharyngeal carcinoma presented post chemotherapy and radiation completed several months prior. A restaging combined positron emission tomography-computed tomography (PET-CT) scan revealed an isolated right infrahilar, station 12R, 1 cm hypermetabolic LN suspicious for metastatic disease. We were asked to biopsy the lesion to confirm recurrent disease and place FMs for SBRT.
The patient was brought to the bronchoscopy suite and underwent MAC anesthesia. Using the dedicated 7.5 MHz EBUS bronchoscope and a 21-G EBUS needle, a 1 cm right infrahilar LN correlating to the radiological finding was identified and sampled by EBUS-guided transbronchial needle aspiration (TBNA). ROSE cytology confirmed the presence of malignant cells in the lesion. Using the above listed steps, modified FMs were then placed, under real-time ultrasound visualization, into the lesion of interest. The patient went on to receive FM-guided SBRT. Subsequent imaging revealed the FMs to be in stable position over several months follow-up (Figure 2B).
Case 2
An 84-year-old female with a history of left upper lobe squamous cell carcinoma of the lung resected 15 years prior was found on surveillance PET-CT to have a hypermetabolic 2 cm × 2 cm right upper lobe nodule approximately in the lower right paratracheal region. Previously, the lesion had been biopsied and proven to be squamous cell lung cancer. A decision was made to treat with SBRT, and we were asked to place a FM. Using the same technique as described in case 1, three modified FM were put in the lesion using real-time CP-EBUS visualization. The patient underwent FM guided SBRT to her malignant lesion.
Procedural outcomes
All patients went on to receive FM guided SBRT to their malignant lesions. There were no cases of FM migration or malfunction (Figure 2B) and the modified FMs worked as intended in accurately guiding SBRT to the targeted volume. No adverse events related to the diagnostic biopsy or FM placement procedures were noted. On follow-up, there was no evidence of FM migration or expectoration, hemoptysis, pneumothorax, or pulmonary or mediastinal infection. Further, we are not aware of any serious adverse events related to the SBRT treatment of our patients’ centrally located lesions.
Discussion
In 2016, an estimated 224,390 new US cases of lung cancer were diagnosed with 158,080 deaths from lung cancer (21). Incidence of lung cancer diagnoses, and especially early disease, is likely to increase after the results of the National Lung Screening Trial (NLST) showed a relative reduction in mortality for patients screened with low dose CT (LDCT) of 20.0% (22). This led to the recommendation of LDCT screening in selected groups by the U.S. Preventative Service Task Force (23). The determining factor in lung cancer survival is the distinction between early and late stage disease, as the former can usually be managed with surgical resection of lung tumor. However, as many as 25% of patients with potentially surgical disease may be ineligible to surgery due to prohibitively diminished pulmonary function or medical comorbidities (24). Twenty-five percent of patients with stage 1A lung cancer are, unfortunately, ineligible for surgical remedy due to age, comorbidity, or preference (24). This incidence is likely to continue to increase as the population ages further, and with continued widespread adoption of lung cancer screening.
SBRT in inoperable lung cancer and oligometastatic disease
Historically, patients with inoperable early stage NSCLC were offered conventional radiation therapy (RT) or observation alone. While there is a survival benefit to receiving conventional RT, the 3-year survival of either approach is well below 50% (25). Early on, attempts to improve tumor control by escalating radiation doses were offset by organ toxicity. The unique radiobiology of SBRT has been shown to cause secondary killing of tumor cells through induction of apoptosis, vascular damage, and enhancement of anti-tumor responses (8). These are crucial therapeutic mechanisms of SBRT, but its defining characteristic is accuracy. This feature permits SBRT to deliver highly therapeutic doses of radiation while minimizing collateral damage to adjacent, healthy, tissues. Beginning in the 1990s, technological advances and computer modeling allowed for significant advances by way of stereotactic body frames and improved image guidance (8). This allowed for the development of stereotactic body radiation therapy (SBRT), a form of ionizing RT, which can be delivered with a high degree of accuracy over a single dose, or a small number of fractions, to a peripheral or central thoracic target (9). In 2010, results of a phase 2 North American multicenter trial led to the wide acceptance of SBRT’s potential. In this landmark study, RTOG 0236, Timmerman et al., performed SBRT on patients with biopsy proven peripheral NSCLC stages T1–T2, N0, M0 who were non-operable. These patients received a total of 54 gray (Gy) delivered in 3 fractions over 1–2 weeks. This treatment resulted in a 3-year survival rate of 55.8% (26). To date, no other development in the management of NSCLC has had such a positive impact on patient survival.
SBRT planning
Effective radiotherapy of treatment of tumors in the thorax must balance tumor control with minimizing toxicity to surrounding tissues, including adjacent lung parenchyma, spinal cord, esophagus, heart, and great vessels. Planning starts with a radiation oncologist simulating therapy with CT/PET, MRI, or a combination of all these modalities. Images are then transferred to a planning computer where the radiation oncologist and physicist will outline the gross tumor volume (GTV). GTV can then be extended to account for microscopic spread of tumor creating the clinical target volume (CTV). Finally, consideration of tumor motion due to respiratory and or cardiac motions, along with detailed information regarding the geometry of normal surrounding tissues, will result in the final planning target volume (PTV). Radiation beams will then be contoured to the PTV to maximize accuracy and minimize toxicity (9).
Further accuracy of SBRT is attributed to its ability to deliver radiation to moving targets, especially in the thorax. This is achieved by careful positioning, immobilization of the patient during simulation and treatment and respiratory tracking or gating. 4-dimensional (4DCT) scans, slow CT scans, confocal CT, and or audio-visual biofeedback are some of the ways respiratory motion is accounted for during simulation and treatment (8). Another way of tracking tumor movement is with the use of internal FMs.
FMs
In FM based tracking, 2–3 fiducials are placed adjacent to or within the target lesion. FMs are generally composed of gold, as these are dense enough to be targeted by SBRT tracking systems. After placement, 3D or 4D (4th dimension = time-tracked) treatment planning will be performed using the FMs as reference points for computer simulation, and can even be tracked in real time by some SBRT platforms (27). For example, the CyberKnife Robotic radiosurgery system (Accuray, Inc., Sunnyvale, CA, USA) is an SBRT platform that delivers highly accurate therapy by way of FM tracking (17,28-33). During CyberKnife treatments, a robotic arm tracks internally placed fiducials in real-time during SBRT sessions. CyberKnife tracking depends on contrast differences between tumor and adjacent tissue. FMs are essential to aid accurate targeting, particularly when the lesion of interest is less than 20 mm in diameter or is adjacent to the heart or spine (34). Traditionally, FMs have been placed using a transthoracic CT-guided approach, a procedure with up to a 30–50% rate of pneumothorax rate when performed for this indication (27,35-39). This presents a uniquely problematic issue in a patient population with high incidence of severely compromised lung function. This approach is also suboptimal for central thoracic lesions given the need to cross more lung and thoracic structures to reach the lesion, and this results in a significantly higher risk of pneumothorax and bleeding.
Bronchoscopy and SBRT
Patients being considered for SBRT should be carefully evaluated for any LN spread of disease using a systematic approach. A potential drawback of SBRT versus surgery is the lack of LN sampling. A study evaluating the additive diagnostic benefit of CP-EBUS when considering SBRT found EBUS-TBNA led to stage shift in 47 out of 266 patients (18%). Patients were upstaged, and therefore considered ineligible for SBRT, but 40 (54%) were also down staged to N0 after EBUS-TBNA, and therefore became SBRT eligible (40). Studies are ongoing (NCT01786590 and NCT02719847) comparing CP-EBUS to PET scan and its additive benefit for use in patients being considered for SBRT.
Combined CP-EBUS, electromagnetic navigation bronchoscopy (ENB), and radial probe endobronchial ultrasound (RP-EBUS) to diagnose, stage patients and place FMs, if appropriate, in a single procedure have been demonstrated by other groups (17,31,33,34,41-52) (see Table 2).
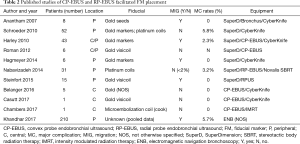
Full table
The above referenced approaches have differences in certain procedural details depending whether the FM was modified or not, whether FM were front-loaded or back-loaded, and the FMs used. However, they all share the characteristics of providing a single, “all-in-one,” bronchoscopic approach to diagnosis, staging, and FM placement which is safe, convenient, and cost-effective.
Another advantage to bronchoscopy as a delivery mechanism for FM placement is the complimentary nature of CP-EBUS delivered FM and RP-EBUS plus ENB delivered FM. CP-EBUS can be used to for systematic hilar and mediastinal staging, and accurate delivery of FMs to central targets including LNs and central parenchymal lesions (24,41,42,44). The RP-EBUS+ENB approach is similarly safe and effective in biopsy and FM marker placement of, and in, peripheral lesions that might be outside the reach of CP-EBUS (24,28,33,47,48,50,53-55). Relative to CT-guided percutaneous FM placement, RP-EBUS + ENB delivery of FMs results in fewer complications; namely iatrogenic pneumothorax. While the two modalities have not been directly compared, the estimated rate of pneumothorax with CT-guided FM delivery versus RP-EBUS + ENB FM delivery is approximately 30% versus 6%, respectively (33,42). Notably, FMs delivered via either approach have not been shown to migrate out of position after placement over time (28,34,41,56).
Taken together, CP-EBUS and RP-EBUS + ENB, allow for complete bronchoscopic access to the lung parenchyma and thoracic LNs. This avoids exposing patients to multiple procedures and anesthesia. Bronchoscopy for diagnosis, staging, and placement of FMs can be safely performed in a single procedure, even in high-risk populations (24). This provides for safe and efficient diagnosis, staging, and FM placement with maximal patient convenience.
We believe our technique has numerous favorable characteristics. As CP-EBUS is becoming ubiquitous, use of available equipment results in significant cost savings. Similarly, training in EBUS bronchoscopy is largely the norm in pulmonary fellowship training programs. For the physician facile with the use of CP-EBUS, FM placement by this technique is a simple procedure to learn and perform, with little overall added procedure time. Combining diagnosis, staging, and FM placement in once procedure may result in further cost savings, convenience, and improved safety for the patient. Although a small series, this technique has the same excellent safety profile as EBUS-TBNA with minimal bleeding or pneumothorax risk, a major advantage over CT-guided transthoracic approach (57-61). Finally, this technique allows for real-time ultrasound guided direct visualization of FM placement, which facilitates precise and effective placement of FM. A reasonable concern is whether our FM modification process compromises their efficacy or predisposes them to migration. In our series, the modified FMs performed exactly as required for targeting, with no evidence of migration as indicated by surveillance imaging (Figure 2B). A limitation of our technique owes to the large size of the CP-EBUS bronchoscope (6.8 mm outer diameter), which confines this approach to mediastinal and hilar LNs and centrally located lesions. Once technology evolves and smaller CP-EBUS bronchoscopes are made, the technique may be expanded to more peripheral lesions. In this respect, FM placement via CT-guided biopsy, ENB-guided, and RP-EBUS placement offer complementary approaches to access peripheral lesions currently beyond the reach of our technique.
Given the density of critical organs (e.g., heart, trachea, mainstem bronchi, esophagus) and neurovascular structures (e.g., great vessels, recurrent laryngeal and vagus nerves) located within the central thorax, a longstanding concern has been that application of SBRT to centrally located lesions posts an unacceptably high risk of serious or even fatal complications due to collateral damage of adjacent healthy structures. In particular, the 2 cm area extending from the main carina to the lobar bronchi, colloquially known as the “no-fly zone (NFZ)” was considered to be especially high risk to treat with SBRT (62). Potential serious complications included airway necrosis, fatal hemoptysis, severe esophagitis, pneumonitis, neuropathies, bronchial stenosis, and atelectasis. As a result, traditional thinking was that lesions in the NFZ of the central thorax were not well suited to SBRT. However, since this concern was first published in 2006, much progress has been made in the planning and delivery of SBRT to central thoracic lesions, including the NFZ to significantly mitigate this risk. First, improved and refined planning and delivery technology for SBRT has further increased the precision of this treatment approach reducing the affected volume of normal tissue adjacent to central lesions being treated with SBRT (63). Second, as the number and breadth of patients undergoing SBRT increases over time, groups at higher risk for serious central lesion SBRT-related complications have been identified and can now be prospectively identified as requiring an alternative treatment plan (64,65). Along these same lines, numerous risk mitigation SBRT strategies, such as hyperfractionation or dose reduction, have been developed to treat patients with high-risk NFZ central lesions being considered for SBRT (66,67). Advances in technology, improved patient selection, and refinement and customization of SBRT treatment strategies now make treatment of centrally located thoracic lesions with SBRT a highly efficacious option with an acceptable rate of minor and major complications, and more recently published case series are reflective of this (66,68-70).
Coding and billing
SBRT (Thoracic) Planning CPT 32701 is defined as thoracic target(s) delineation for stereotactic body radiation therapy (SRS/SBRT), (photon or particle beam), entire course of treatment (71).
SBRT planning is a collaborative service between the surgeon or operator, and radiation oncologist that is distinct from treatment, dosimetry, or management by the radiation oncologist. Planning occurs prior to treatment and is billed once to capture the planning for the entire course of treatment. During the planning process, thoracic targets, borders, volume, relation to adjacent structures, and if available, FMs are delineated. Note that placement of FMs (CPT 31626 if done via bronchoscopy) is a distinct procedure from SBRT planning, done separately, and does not impact the SBRT coding. Planning may also help validate target in tracking system that does not use fiducials depending on software capabilities (see Figures 3,4). Planning systems have become more comprehensive and offer the ability to generate detailed reports prior to treatment.
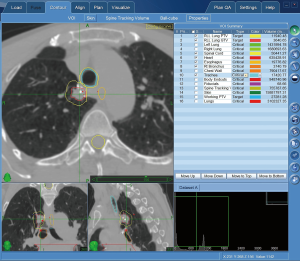
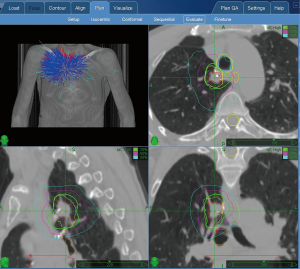
SBRT documentation requirements include:
- Collaboration between physician and radiation oncologist;
- Correlation of tumor and contiguous body structures;
- Determination of borders and volume of tumor;
- Identification of FM;
- of target when FMs are not used.
Reporting requirements include:
- Computer planning with image fusion;
- Using available imaging, collaboration to outline target and help develop dosimetry plans (s) and select the plan with focus on maximal treatment to target lesion and minimal impact on surrounding structures;
- Communication with patient, family, and treating physicians;
- Availability to assist optimal patient positioning or to adjust plan;
- Any supporting notes and orders;
- Signature credentials, date, time.
In our center after patient has undergone successful FM placement SBRT planning is initiated. We perform a thorough review of the imaging, note the volume of the tumor, determine the borders and contiguous body structures, identify FM and document this clearly as described above. We collaborate with radiation oncologist to develop the dosimetry plans and once plans are finalized these are uploaded in the EMR as part of documentation.
Conclusions
Our experience demonstrates the feasibility, efficacy, and safety of combining CP-EBUS and FM placement, and can be widely performed by any pulmonologist trained in the use of EBUS-bronchoscopy with little added training, cost, or risk. Our case series demonstrates the repeated successful clinical application of this approach in a diverse group of patients, as well as highlighting its broader applicability to peribronchial recurrent or oligo-metastatic thoracic neoplasms of any origin, not simply early stage bronchogenic neoplasms. We also highlight the ever-expanding applications of CP-EBUS. This trend is likely to continue as the size of CP-EBUS bronchoscopes shrink, increasing the reach of CP-EBUS, and applications for delivering therapeutics directly to lesions via bronchoscopy are developed. In conjunction with RP-EBUS + ENB, almost complete endobronchial access to the thoracic LNs and lungs is achievable, and represents a new safe, effective, and convenient option for patients in the diagnosis, staging, and treatment of intrathoracic malignancies.
Acknowledgements
None.
Footnote
Conflicts of Interest: The authors have no conflicts of interest to declare.
References
- Ernst A, Anantham D, Eberhardt R, et al. Diagnosis of mediastinal adenopathy-real-time endobronchial ultrasound guided needle aspiration versus mediastinoscopy. J Thorac Oncol 2008;3:577-82. [Crossref] [PubMed]
- Ernst A, Feller-Kopman D, Herth FJ. Endobronchial ultrasound in the diagnosis and staging of lung cancer and other thoracic tumors. Semin Thorac Cardiovasc Surg 2007;19:201-5. [Crossref] [PubMed]
- Herth FJ, Eberhardt R, Vilmann P, et al. Real-time endobronchial ultrasound guided transbronchial needle aspiration for sampling mediastinal lymph nodes. Thorax 2006;61:795-8. [Crossref] [PubMed]
- Herth FJ, Ernst A, Eberhardt R, et al. Endobronchial ultrasound-guided transbronchial needle aspiration of lymph nodes in the radiologically normal mediastinum. Eur Respir J 2006;28:910-4. [Crossref] [PubMed]
- Herth F, Becker HD, Ernst A. Conventional vs endobronchial ultrasound-guided transbronchial needle aspiration: a randomized trial. Chest 2004;125:322-5. [Crossref] [PubMed]
- Vilmann P, Clementsen PF, Colella S, et al. Combined endobronchial and esophageal endosonography for the diagnosis and staging of lung cancer: European Society of Gastrointestinal Endoscopy (ESGE) Guideline, in cooperation with the European Respiratory Society (ERS) and the European Society of Thoracic Surgeons (ESTS). Endoscopy 2015;47:545-59. [Crossref] [PubMed]
- Yasufuku K, Nakajima T, Motoori K, et al. Comparison of endobronchial ultrasound, positron emission tomography, and CT for lymph node staging of lung cancer. Chest 2006;130:710-8. [Crossref] [PubMed]
- Kelsey CR, Salama JK. Stereotactic body radiation therapy for treatment of primary and metastatic pulmonary malignancies. Surg Oncol Clin N Am 2013;22:463-81. [Crossref] [PubMed]
- Inoue T, Katoh N, Onimaru R, et al. Clinical outcomes of stereotactic body radiotherapy for patients with lung tumors in the state of oligo-recurrence. Pulm Med 2012;2012:369820. [PubMed]
- Bradley JD, El Naqa I, Drzymala RE, et al. Stereotactic body radiation therapy for early-stage non-small-cell lung cancer: the pattern of failure is distant. Int J Radiat Oncol Biol Phys 2010;77:1146-50. [Crossref] [PubMed]
- Chang JY, Balter PA, Dong L, et al. Stereotactic body radiation therapy in centrally and superiorly located stage I or isolated recurrent non-small-cell lung cancer. Int J Radiat Oncol Biol Phys 2008;72:967-71. [Crossref] [PubMed]
- Navarria P, Ascolese AM, Tomatis S, et al. Stereotactic body radiotherapy (sbrt) in lung oligometastatic patients: role of local treatments. Radiat Oncol 2014;9:91. [Crossref] [PubMed]
- Oh D, Ahn YC, Seo JM, et al. Potentially curative stereotactic body radiation therapy (SBRT) for single or oligometastasis to the lung. Acta Oncol 2012;51:596-602. [Crossref] [PubMed]
- Takahashi W, Yamashita H, Niibe Y, et al. Stereotactic body radiotherapy for metastatic lung cancer as oligo-recurrence: an analysis of 42 cases. Pulm Med 2012;2012:454107. [PubMed]
- Taremi M, Hope A, Dahele M, et al. Stereotactic body radiotherapy for medically inoperable lung cancer: prospective, single-center study of 108 consecutive patients. Int J Radiat Oncol Biol Phys 2012;82:967-73. [Crossref] [PubMed]
- Smith RL, Yang D, Lee A, et al. The correlation of tissue motion within the lung: implications on fiducial based treatments. Med Phys 2011;38:5992-7. [Crossref] [PubMed]
- Harada T, Shirato H, Ogura S, et al. Real-time tumor-tracking radiation therapy for lung carcinoma by the aid of insertion of a gold marker using bronchofiberscopy. Cancer 2002;95:1720-7. [Crossref] [PubMed]
- Guckenberger M, Richter A, Boda-Heggemann J, et al. Motion compensation in radiotherapy. Crit Rev Biomed Eng 2012;40:187-97. [Crossref] [PubMed]
- Shirato H, Seppenwoolde Y, Kitamura K, et al. Intrafractional tumor motion: lung and liver. Semin Radiat Oncol 2004;14:10-8. [Crossref] [PubMed]
- Murphy MJ. Fiducial-based targeting accuracy for external-beam radiotherapy. Med Phys 2002;29:334-44. [Crossref] [PubMed]
- Siegel RL, Miller KD, Jemal A. Cancer statistics, 2016. CA Cancer J Clin 2016;66:7-30. [Crossref] [PubMed]
- National Lung Screening Trial Research T, Aberle DR, Adams AM, et al. Reduced lung-cancer mortality with low-dose computed tomographic screening. N Engl J Med 2011;365:395-409. [Crossref] [PubMed]
- Moyer VA, Force USPST. Screening for lung cancer: U.S. Preventive Services Task Force recommendation statement. Ann Intern Med 2014;160:330-8. [PubMed]
- Kular H, Mudambi L, Lazarus DR, et al. Safety and feasibility of prolonged bronchoscopy involving diagnosis of lung cancer, systematic nodal staging, and fiducial marker placement in a high-risk population. J Thorac Dis 2016;8:1132-8. [Crossref] [PubMed]
- Potters L, Gaspar LE, Kavanagh B, et al. American Society for Therapeutic Radiology and Oncology (ASTRO) and American College of Radiology (ACR) practice guidelines for image-guided radiation therapy (IGRT). Int J Radiat Oncol Biol Phys 2010;76:319-25. [Crossref] [PubMed]
- Timmerman R, Paulus R, Galvin J, et al. Stereotactic body radiation therapy for inoperable early stage lung cancer. JAMA 2010;303:1070-6. [Crossref] [PubMed]
- Yousefi S, Collins BT, Reichner CA, et al. Complications of thoracic computed tomography-guided fiducial placement for the purpose of stereotactic body radiation therapy. Clin Lung Cancer 2007;8:252-6. [Crossref] [PubMed]
- Nabavizadeh N, Zhang J, Elliott DA, et al. Electromagnetic navigational bronchoscopy-guided fiducial markers for lung stereotactic body radiation therapy: analysis of safety, feasibility, and interfraction stability. J Bronchology Interv Pulmonol 2014;21:123-30. [Crossref] [PubMed]
- Collins BT, Vahdat S, Erickson K, et al. Radical cyberknife radiosurgery with tumor tracking: an effective treatment for inoperable small peripheral stage I non-small cell lung cancer. J Hematol Oncol 2009;2:1. [Crossref] [PubMed]
- Pop D, Venissac N, Bondiau PY, et al. Peroperative fiducial placement for postoperative stereotactic Cyberknife radiosurgery. Interact Cardiovasc Thorac Surg 2010;10:1034-6. [Crossref] [PubMed]
- Unger K, Ju A, Oermann E, et al. CyberKnife for hilar lung tumors: report of clinical response and toxicity. J Hematol Oncol 2010;3:39. [Crossref] [PubMed]
- Patel A, Khalsa B, Lord B, et al. Planting the seeds of success: CT-guided gold seed fiducial marker placement to guide robotic radiosurgery. J Med Imaging Radiat Oncol 2013;57:207-11. [Crossref] [PubMed]
- Schroeder C, Hejal R, Linden PA. Coil spring fiducial markers placed safely using navigation bronchoscopy in inoperable patients allows accurate delivery of CyberKnife stereotactic radiosurgery. J Thorac Cardiovasc Surg 2010;140:1137-42. [Crossref] [PubMed]
- Lachkar S, Guisier F, Roger M, et al. Assessment by Endoscopically Guided Placement of Fiducial Gold Markers for Small Peripheral Lung Nodules < 20 mm Before Stereotactic Radiation Therapy. Chest 2018;153:387-94. [Crossref] [PubMed]
- Bhagat N, Fidelman N, Durack JC, et al. Complications associated with the percutaneous insertion of fiducial markers in the thorax. Cardiovasc Intervent Radiol 2010;33:1186-91. [Crossref] [PubMed]
- Brook OR, Gourtsoyianni S, Mendiratta-Lala M, et al. Safety profile and technical success of imaging-guided percutaneous fiducial seed placement with and without core biopsy in the abdomen and pelvis. AJR Am J Roentgenol 2012;198:466-70. [Crossref] [PubMed]
- Mendiratta-Lala M, Sheiman R, Brook OR, et al. CT-guided core biopsy and percutaneous fiducial seed placement in the lung: can these procedures be combined without an increase in complication rate or decrease in technical success? Eur J Radiol 2014;83:720-5. [Crossref] [PubMed]
- Kothary N, Heit JJ, Louie JD, et al. Safety and efficacy of percutaneous fiducial marker implantation for image-guided radiation therapy. J Vasc Interv Radiol 2009;20:235-9. [Crossref] [PubMed]
- Trumm CG, Haussler SM, Muacevic A, et al. CT fluoroscopy-guided percutaneous fiducial marker placement for CyberKnife stereotactic radiosurgery: technical results and complications in 222 consecutive procedures. J Vasc Interv Radiol 2014;25:760-8. [Crossref] [PubMed]
- Vial MR, Khan KA, O'Connell O, et al. Endobronchial Ultrasound-Guided Transbronchial Needle Aspiration in the Nodal Staging of Stereotactic Ablative Body Radiotherapy Patients. Ann Thorac Surg 2017;103:1600-5. [Crossref] [PubMed]
- Belanger AR, Zagar T, Akulian JA. Convex Endobronchial Ultrasound-Guided Fiducial Placement for Malignant Central Lung Lesions: A Case Series. J Bronchology Interv Pulmonol 2016;23:46-50. [Crossref] [PubMed]
- Argento AC, Decker R, Puchalski J. Fiducial Marker Placement Via Convex Probe EBUS. J Bronchology Interv Pulmonol 2016;23:181-5. [Crossref] [PubMed]
- Casutt A, Koutsokera A, Peguret N, et al. Linear-Endobronchial Ultrasound-Guided Insertion of a Fiducial Marker: A New Tool for Tracking Central Lesions. J Bronchology Interv Pulmonol 2017;24:166-9. [Crossref] [PubMed]
- Chambers DM, Pfister GJ, Gauhar UA. Linear EBUS-guided fiducial marker placement to guide radiotherapy for endobronchial, radiographically occult synchronous primary squamous cell carcinoma of the lung. Respir Med Case Rep 2017;22:60-3. [Crossref] [PubMed]
- Harley DP, Krimsky WS, Sarkar S, et al. Fiducial marker placement using endobronchial ultrasound and navigational bronchoscopy for stereotactic radiosurgery: an alternative strategy. Ann Thorac Surg 2010;89:368-73; discussion 373-4. [Crossref] [PubMed]
- Harris K, Gomez J, Dhillon SS, et al. Convex probe endobronchial ultrasound placement of fiducial markers for central lung nodule (with video). Endosc Ultrasound 2015;4:156-7. [Crossref] [PubMed]
- Linden PA. Use of navigation bronchoscopy for biopsy and endobronchial fiducial placement. Innovations (Phila) 2011;6:271-5. [Crossref] [PubMed]
- Steinfort DP, Siva S, Kron T, et al. Multimodality guidance for accurate bronchoscopic insertion of fiducial markers. J Thorac Oncol 2015;10:324-30. [Crossref] [PubMed]
- Temming S, Kocher M, Stoelben E, et al. Risk-adapted robotic stereotactic body radiation therapy for inoperable early-stage non-small-cell lung cancer. Strahlenther Onkol 2018;194:91-7. [Crossref] [PubMed]
- Anantham D, Feller-Kopman D, Shanmugham LN, et al. Electromagnetic navigation bronchoscopy-guided fiducial placement for robotic stereotactic radiosurgery of lung tumors: a feasibility study. Chest 2007;132:930-5. [Crossref] [PubMed]
- Harris CL, Toloza EM, Klapman JB, et al. Minimally invasive mediastinal staging of non-small-cell lung cancer: emphasis on ultrasonography-guided fine-needle aspiration. Cancer Control 2014;21:15-20. [Crossref] [PubMed]
- McGuire FR, Liming J, Ochran T, et al. Real-time endobronchial ultrasound-guided implantation of radiotherapy monitoring devices. J Bronchology Interv Pulmonol 2007;14:59-62.
- Hagmeyer L, Priegnitz C, Kocher M, et al. Fiducial marker placement via conventional or electromagnetic navigation bronchoscopy (ENB): an interdisciplinary approach to the curative management of lung cancer. Clin Respir J 2016;10:291-7. [Crossref] [PubMed]
- Khandhar SJ, Bowling MR, Flandes J, et al. Electromagnetic navigation bronchoscopy to access lung lesions in 1,000 subjects: first results of the prospective, multicenter NAVIGATE study. BMC Pulm Med 2017;17:59. [Crossref] [PubMed]
- Tofts RP, Lee PM, Sung AW. Interventional pulmonology approaches in the diagnosis and treatment of early stage non small cell lung cancer. Transl Lung Cancer Res 2013;2:316-31. [PubMed]
- Hong JC, Yu Y, Rao AK, et al. High retention and safety of percutaneously implanted endovascular embolization coils as fiducial markers for image-guided stereotactic ablative radiotherapy of pulmonary tumors. Int J Radiat Oncol Biol Phys 2011;81:85-90. [Crossref] [PubMed]
- Evison M, Crosbie PA, Martin J, et al. EBUS-TBNA in elderly patients with lung cancer: safety and performance outcomes. J Thorac Oncol 2014;9:370-6. [Crossref] [PubMed]
- Fernández-Villar A, Mouronte-Roibas C, Botana-Rial M, et al. Ten Years of Linear Endobronchial Ultrasound: Evidence of Efficacy, Safety and Cost-effectiveness. Arch Bronconeumol 2016;52:96-102. [Crossref] [PubMed]
- Choi YR, An JY, Kim MK, et al. The diagnostic efficacy and safety of endobronchial ultrasound-guided transbronchial needle aspiration as an initial diagnostic tool. Korean J Intern Med 2013;28:660-7. [Crossref] [PubMed]
- Grosu HB, Morice RC, Sarkiss M, et al. Safety of flexible bronchoscopy, rigid bronchoscopy, and endobronchial ultrasound-guided transbronchial needle aspiration in patients with malignant space-occupying brain lesions. Chest 2015;147:1621-8. [Crossref] [PubMed]
- Varela-Lema L, Fernandez-Villar A, Ruano-Ravina A. Effectiveness and safety of endobronchial ultrasound-transbronchial needle aspiration: a systematic review. Eur Respir J 2009;33:1156-64. [Crossref] [PubMed]
- Timmerman R, McGarry R, Yiannoutsos C, et al. Excessive toxicity when treating central tumors in a phase II study of stereotactic body radiation therapy for medically inoperable early-stage lung cancer. J Clin Oncol 2006;24:4833-9. [Crossref] [PubMed]
- Henke L, Kashani R, Yang D, et al. Simulated Online Adaptive Magnetic Resonance-Guided Stereotactic Body Radiation Therapy for the Treatment of Oligometastatic Disease of the Abdomen and Central Thorax: Characterization of Potential Advantages. Int J Radiat Oncol Biol Phys 2016;96:1078-86. [Crossref] [PubMed]
- Peulen H, Karlsson K, Lindberg K, et al. Toxicity after reirradiation of pulmonary tumours with stereotactic body radiotherapy. Radiother Oncol 2011;101:260-6. [Crossref] [PubMed]
- Haseltine JM, Rimner A, Gelblum DY, et al. Fatal complications after stereotactic body radiation therapy for central lung tumors abutting the proximal bronchial tree. Pract Radiat Oncol 2016;6:e27-33. [Crossref] [PubMed]
- Modh A, Rimner A, Williams E, et al. Local control and toxicity in a large cohort of central lung tumors treated with stereotactic body radiation therapy. Int J Radiat Oncol Biol Phys 2014;90:1168-76. [Crossref] [PubMed]
- Kannarunimit D, Descovich M, Garcia A, et al. Analysis of dose distribution and risk of pneumonitis in stereotactic body radiation therapy for centrally located lung tumors: a comparison of robotic radiosurgery, helical tomotherapy and volumetric modulated arc therapy. Technol Cancer Res Treat 2015;14:49-60. [Crossref] [PubMed]
- Davis JN, Medbery C, Sharma S, et al. Stereotactic body radiotherapy for centrally located early-stage non-small cell lung cancer or lung metastases from the RSSearch((R)) patient registry. Radiat Oncol 2015;10:113. [Crossref] [PubMed]
- Rowe BP, Boffa DJ, Wilson LD, et al. Stereotactic body radiotherapy for central lung tumors. J Thorac Oncol 2012;7:1394-9. [Crossref] [PubMed]
- Lischalk JW, Malik RM, Collins SP, et al. Stereotactic body radiotherapy (SBRT) for high-risk central pulmonary metastases. Radiat Oncol 2016;11:28. [Crossref] [PubMed]
- American Medical Association. CPT 2016 Professional Edition (Current Procedural Terminology, Professional Ed. (Spiral)) (Current Procedural Terminology (CPT) Professional) 2016th Edition. American Medical Association, 2016.