Nontuberculous mycobacterial pulmonary infections
Submitted Jul 12, 2013. Accepted for publication Dec 12, 2013.
doi: 10.3978/j.issn.2072-1439.2013.12.24
Introduction
Historically, human infections due to Mycobacterium were due almost exclusively to Mycobacterium tuberculosis (TB); the extensive societal impact of this infection is legendary. More recently, other species of mycobacterium causing clinical disease have been identified and, in many geographical regions, cause greater disease burden than TB. These organisms are referred to by a variety of collective names—anonymous or atypical mycobacteria, mycobacteria other than tuberculosis (MOTT) and nontuberculous mycobacteria (NTM). In this paper, the acronym NTM is used to collectively describe NTM organisms.
Similar to TB, NTM infections can occur throughout the body. However, pulmonary infections, lymphadenitis, and skin and soft tissue infections are the most commonly described attributable human infections (1). Both host factors and organism characteristics influence the susceptibility and manifestations of infection (1).
Overview of atypical mycobacteria
Mycobacteria are aerobic, non-motile organisms that appear positive with acid-fast alcohol stains (2). They have a lipid rich, hydrophobic cell wall, which is substantially thicker than most other bacteria (2). The thickness and composition of the cell wall renders mycobacteria impermeable to hydrophilic nutrients and resistant to heavy metals, disinfectants, and antibiotics (3).
NTM are ubiquitous in the environment with the heaviest concentrations found in soil and water sources. They are associated with biofilm formation (4), which contributes to disinfectant and antibiotic resistance (3,5). The hydrophobicity of NTM results in preferential aerosolization from water (6), and many of these organisms are resistant to high temperature and are relatively resistant to low pH (7,8).
Given these characteristics of NTM it is not surprising that drinking water, household plumbing, peat rich soils, brackish marshes, and drainage water are reservoirs of NTM (9). Water systems in hospitals, hemodialysis centers, and dental offices have particularly high rates of mycobacterium colonization (10). When sampling a potential source for NTM colonization, biofilms should be included in the sampled specimens given the organisms’ predilection for biofilm adherence (11-13).
Currently, there are more than 150 species of Mycobacterium and it is likely that more will be discovered. The rapid increase in identified species in recent years is due to improved culturing techniques and more precise differentiations of species. Species differentiation improved dramatically with the development of molecular techniques that enabled the detection of differences in the 16S rRNA gene (14). This gene is highly conserved amongst species and slight differences characterize different species. A full listing of recognized NTM can be found at www.bacterio.cict.fr/m/mycobacterium.html.
Although mycobacterium organisms other than TB were identified soon after Koch’s identification of TB in 1882, it was not until the 1950’s when they were recognized to cause human disease (15). Historically, different classification systems have been proposed, but NTM are most commonly classified by growth rate—either slowly growing or rapidly growing (Table 1). By far, the most common organism associated with pulmonary disease is the Mycobacterium avium complex (MAC), a slow growing NTM that encompasses many subspecies including avium, silvaticum, hominissuis, and paratuberculosis, as well as the species intracellulare, arosiense, chimaera, colombiense, marseillense, timonense, bouchedurhonense, and ituriense. Mycobacterium kasassii, also a slow growing organism, is the second most common cause of pulmonary infections in the United States and is responsible for pockets of infection in England (16,17) M. abscessus, is the most commonly isolated rapidly growing NTM and is the third most common cause of lung disease (16). Although most NTM lung infections are caused by these three organisms, it is important to recognize that many other NTM may cause pulmonary disease in both immunocompetent and immunocompromised hosts. Thus, the pathogenic significance of a NTM specimen must be determined in the context of a patient’s clinical presentation (18,19).
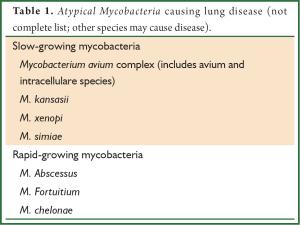
Full table
Epidemiology
Over the last three decades, it has been suggested that the incidence of both NTM laboratory isolation and disease prevalence is increasing. This change has been attributed, in part, to improved culturing techniques, coupled with greater disease awareness and a true increase in disease prevalence. However, it is challenging to accurately characterize the incidence and prevalence of NTM pulmonary infections since isolation of the organism does not universally indicate clinical infection. Additionally, NTM infections, unlike TB, do not require public health reporting, which hinders an accurate understanding of epidemiology. Furthermore, nearly all epidemiologic data is reported from the United States, Japan, and Europe, and thus, may not be reflective of changes in prevalence worldwide.
When similar culture techniques are employed, environmental recovery is similar in various geographical regions (20). In Western societies, most laboratories report a dramatically greater prevalence of NTM than TB (21). However, not all positive NTM cultures represent infection. A recent analysis showed that approximately half of those with positive NTM respiratory cultures fulfilled clinical criteria for active infection (22). In Kendall and Winthrop’s review, the prevalence of NTM pulmonary infections based on laboratory records coupled with clinical characteristics varied between 4.1 and 14.1 per 100,000 patient years (23). In patients greater than 65 years the prevalence was 47 per 100,000 patient years (24). Women are also more likely to have NTM disease than men (24), the disease prevalence increases with age (25), and it is more common in the West and Southeast (24). In the United States, Caucasians account for 90% of cases followed by Asians/Pacific Islanders and Blacks (24).
In a review from Oregon, NTM pulmonary infections were associated with more densely populated areas, suggesting that urban municipal water supply exposure predisposed individuals to disease (26). However, in Japan, NTM was more commonly found in farmers and gardeners than in urban patients with bronchiectasis, suggesting a greater etiologic role for exposure to soil than water sources (27).
Skin testing for MAC antigens suggests that exposure to MAC is not uncommon and varies greatly geographically. Early studies of United States Navy recruits demonstrated reactivity to MAC antigens in 10-20% of recruits from northern and western areas of the United States, but over 70% of recruits from the southeast demonstrated MAC antigen reactivity (28).
Currently, greater than 90% of NTM cultures in the United States are from pulmonary secretions (29). At the height of the human immunodeficiency virus (HIV) epidemic, NTM was more commonly cultured from blood due to disseminated infections, but anti-retroviral therapy and appropriate prophylaxis have substantially decreased the incidence of disseminated disease in this population (30).
For reasons that are not fully understood, some pathogenic NTM organisms tend to cluster in specific geographical distributions. In the United States, M. kansasii is most commonly seen in southern and central regions (16). However, even outside of geographically endemic areas for M. kansasii, disease prevalence is high in areas with a substantial HIV disease (31). M. abscessus is most commonly identified in the southeastern United States from Florida to Texas. However, it has also been identified outside of this region.
NTM culture and Identification
When NTM are sought, care must be taken when processing samples. Specimens from non-sterile sites, such as sputum, require decontamination to avoid overgrowth by bacteria or fungi. NTM are not visible on routine Gram stain, so the fluorochrome technique for staining is recommended (2). Culturing specimens in both broth and solid media is recommended. Broth cultures offer the advantage of greater yield and more rapid growth, but they are more susceptible to bacterial overgrowth. Growth of specimens on solid media allows an opportunity to visualize characteristics of colony growth (32). Cultured NTM should be identified to the species level to guide decisions regarding clinical relevance and appropriate therapy. Speciation of NTM can be achieved with polymerase chain reactions, gene probe assays, and high-performance liquid chromatography (33).
When NTM is cultured from a human sample such as sputum, its clinical significance needs to be determined. In some instances the mycobacterial organisms are pathogenic; but in others they are commensal. Many NTM are less virulent than M tuberculosis. Additionally, since NTM are so commonly identified in water systems (9), it is important to assure that NTM in a clinical specimen, especially when present in low concentration, is not the result of contamination from water sources.
Mechanism of infection
The mode of transmission to humans has not been defined. In many instances the Koch postulates do not prevail. Unlike TB, person-to-person transmission has not been convincingly demonstrated. Although animals may serve as a reservoir for NTM, animal to human transmission is not thought to occur. However, shared drinking water systems with animals may serve as a source of infection (34).
Although the exact route of NTM infection is not established with certainty, based on NTM environmental distribution, it is very likely that the organism is ingested, inhaled, or implanted. Cervical lymphadenitis due to NTM occurs more frequently in children, coincident at the time that they are exploring outdoors and there is trauma to gums due to erupting teeth (35). Thus, it is assumed that NTM enter the tissues via the mouth. Previous infections leading to cervical lymphadenitis were most often due to Mycobacterium scrofulaceum; now most are due to MAC (35).
Aerosolization of droplets small enough to enter the alveoli is the likely route of acquisition of pulmonary disease. Bathroom showers have been implicated as a primary source of exposure to aerosolized NTM (36). Households with water heater temperatures ≤50 °C are more likely to demonstrate colonization of their water supply with NTM than those with water heater temperature ≥55 °C (37). Potting soils, particularly those enriched with peat, have a high concentration of NTM and dust generated from soil may produce particles small enough particles to enter the alveoli (38). However, a case controlled cohort study by Dirac et al. looked at “aerosol-generating activities in the home and found that the only activity predictive of the development of NTM lung disease was the use of a spray bottle for watering plants (39).
Contamination of hospital water supplies, medical equipment, including bronchoscopes and endoscopes, and contaminated dialysis solutions, has led to both NTM colonization and nosocomial outbreaks of disease. The site of disease is dependent upon the exposure, but cutaneous abscesses, pulmonary disease, meningitis, and surgical site infections have all been described (10). It is hypothesized that regulations to limit hospital water system temperatures to prevent scalding hinder the control of NTM (40). Advances in DNA techniques have allowed easier and more rapid identification of the source of NTM in the setting of nosocomial outbreaks. However, recognition of nosocomial outbreaks is highly dependent upon clinical suspicion and investigation.
Susceptibility to NTM infection
Nearly everyone is presumed exposed to NTM, yet most do not develop clinical signs of infection. The factors predisposing to infection are not well understood, but likely are due to an interaction between host defense mechanisms and the load of clinical exposure.
Disseminated disease is most commonly seen in association with profound immunosuppression. In HIV infected patients, dissemination does not typically occur unless the CD-4+ T-lymphocyte count is below 50/uL (41). Structural lung disease, such as chronic obstructive pulmonary disease (COPD), silicosis, pneumoconiosis or prior TB infection, predisposes to pulmonary infection. Nodular bronchiectasis is very strongly associated with NTM infections.
NTM infections are of particular importance in patients who are awaiting or have undergone lung transplantation and those with cystic fibrosis (CF) (42,43). The manifestations of infection are protean and may affect soft tissues, bones, and joints, as well as the lung (44). As in other populations, isolation of NTM organisms is not uncommon among transplant patients, but most have transient colonization and do not require treatment. Knoll et al., reviewed lung transplant recipients from 1990-2005 (45). NTM, especially, M. avium was commonly found in the respiratory secretions of this cohort, but a minority of patients fulfilled criteria for active pulmonary infections (45). However, lung transplant patients with NTM after transplantation have a higher mortality (46). In a multi-centered cohort study of 1,582 patients with CF, 6.6% demonstrated sputum positivity for NTM, while 3.3% fulfilled bacteriologic criteria for disease (47). A prior multicenter study showed a 13% prevalence of NTM sputum positivity (48). Molecular typing of the organisms suggested neither person-to-person transmission nor nosocomial spread was common. In study groups, M. avium and M. abscessus were most commonly identified.
Interleukin-12 (IL-12) and interferon-gamma (INF-γ) are crucial elements of the host defense response to NTM. Defects in these pathways increase susceptibility to NTM infections (49). Abnormalities in IFN-γ receptors have been described in association with NTM infections in both individuals and familial clusterings of disease (15). However, therapy with aerosolized IFN-γ has been clinically unrewarding.
Increasing therapeutic use of tumor necrosis factor-alpha (TNF-α) receptor antagonist drugs, especially in rheumatoid arthritis and other connective tissue diseases, has been associated with a concomitant increase in NTM infections. In a review of cases reported to the FDA, Winthrop et al., founds that most cases of NTM infection were due to lung infections, but there were significant extra-pulmonary sites of involvement as well (50). M. avium was responsible for half of the cases. In a review of 8,000 users of anti-TNF-α agents, the rate of NTM infections was 74/100,000 person years (51).
Measures taken may reduce exposure to NTM, and may be warranted in patients with recognized risk factors such as immunosuppression or structural lung disease. Bathroom showers with large diameter water streams have been suggested as preferable to one producing a fine mist. However, a prospective study examining risk factors for NTM lung infection was unable to correlate infection with shower exposure (52). It is presumed beneficial to use a bathroom vent that can exhaust the aerosol rapidly. Higher water temperatures with water heater temperatures greater than 55 °C are associated with lower NTM recovery. Thus, increasing the water heater temperature may be advantageous. Additionally, access to well water rather than use of a piped supply might reduce NTM levels.
Filtration of water has theoretical advantages in reducing the passage of NTM, but these filters are expensive and require replacement regularly (53). Chemical disinfection of water also has potentially negative ramifications by changing the microbial flora. Avoidance of hot tubs, especially in enclosed areas, may be beneficial.
Diagnosis of NTM pulmonary infections
Unlike TB, the isolation of NTM in pulmonary specimens does not equate with disease.
In an effort to standardize the definition of NTM infection, the American Thoracic Society (ATS) and the Infectious Disease Society of America (IDSA) jointly published guidelines in 2007 (16). The diagnosis of NTM pulmonary infection requires the presence of symptoms, radiologic abnormalities, and microbiologic cultures in conjunction with the exclusion of other potential etiologies. Clinical symptoms vary in scope and intensity but chronic cough, often with purulent sputum, is common. Hemoptysis may also be present. Systemic symptoms including malaise, fatigue, and weight loss may occur often in association with advancing disease. As demonstrated in Figures 1-4, various radiographic patterns may be seen in patients with NTM pulmonary infections. Fibrocavitary disease is commonly identified on chest roentgenograms. Characteristic findings include thin walled cavities with an upper lobe distribution and surrounding pleural abnormalities. There is no radiographic finding to reliably differentiate fibrocavitary NTM from TB. NTM in conjunction with nodular bronchiectasis may be visible on chest radiograph, but is best appreciated on high resolution chest computed tomography (HRCT). Characteristic findings include clusters of small nodules usually less than 0.5 mm—the so-called tree-in-bud sign. Larger nodules, with or without cavitation, may occur, which are suspicious for malignancy. Uptake of 18F fluorodeoxyglucose (FDG) on PET scan has been described in nodules due to NTM (54). Infected areas of lung parenchyma may demonstrate atelectasis or cystic or saccular bronchiectasis.
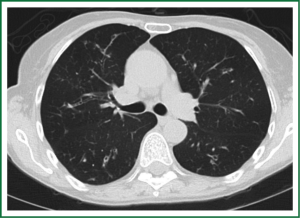
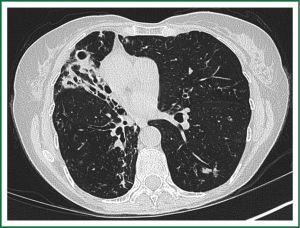
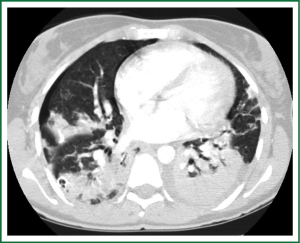
Because NTM are ubiquitous in the environment, especially in water sources, a single positive pulmonary specimen does not fulfill microbiologic criteria for infection. Confirmatory microbiologic findings requires culture growth of NTM from either one bronchoalveolar lavage, two sputum samples, or culture from respiratory tissue demonstrating granulomatous histopathology.
Common clinical scenarios of NTM pulmonary infections
There are two commonly encountered patterns of NTM pulmonary infections. Upper lobe fibrocavitary disease historically had been most well described. It commonly occurs in patients with COPD or other structural lung diseases including silicosis, pneumoconiosis, or prior TB infections. Similar to the historical demographics of COPD, infected patients were often older males with a history of underlying lung disease.
In 1989, Prince et al., described a cohort of 21 patients, 19 of whom were women, with NTM pulmonary infections without known underlying lung disease. They presented with slowly progressive nodular opacities on chest radiograph (55). This report led to the subsequent recognition of NTM infections in association with nodular bronchiectasis. The prototypical patient is an older thin female, often with pectus excavatum, scoliosis, and mitral valve prolapse (56). In these patients, it is uncertain if the NTM infection lead to the development of bronchiectasis, or is a consequence of it.
A presentation suggestive of hypersensitivity pneumonitis (HP) has been reported to occur after exposure to aerosolized MAC, most often in association with indoor hot tub use (57,58). HP has also been suggested to occur in workers exposed to metalworking fluid (59). However, it is often challenging to demonstrate growth of NTM in the workplace (60). Patients with HP often present acutely ill with fevers and pulmonary symptoms in conjunction with various chest radiograph abnormalities. A favorable response to strict avoidance of exposure to the antigen, with or without adjunctive corticosteroid therapy suggests that this is a hypersensitivity reaction rather than true infection (57,58).
Co-infection with different strains of NTM or different organisms is reported. MAC and M. abscessus infections may co-exist, making eradication even more challenging.
Treatment of NTM pulmonary infections: medical therapy
There are numerous clinical challenges regarding the treatment of NTM pulmonary infections. The duration and toxicity of antimycobacterial therapy must be balanced against the often indolent clinical course and the patients other co-morbidities. It is often appropriate to observe a patient’s clinical course before embarking on therapy. There is currently a paucity of data on the natural history of untreated NTM infections adding to the difficulties of clinical decision-making. Kikuchi et al, have demonstrated that specific genotypic patterns of MAC may predict clinical behavior (61). If confirmed by subsequent investigations, these data may augment clinical decision making on directed drug therapy.
Once a decision is made to begin targeted NTM therapy, recommendations for medical therapy are limited by a paucity of adequately powered randomized controlled trials. Often consensus opinion, in conjunction with in vitro susceptibility testing results, guides therapeutic recommendations. The choice of agents and duration of therapy is based upon the specific organism and extent or disease.
Patients with advanced lung disease in need of transplantation present particular challenges when they develop NTM pulmonary infections. It is debated if listing for transplant should be deferred until completion of therapy. Because of the long duration of therapy for NTM, it is the authors’ opinion that listing these patients for bilateral lung transplants should not be delayed, as the involved lungs will be resected at the time of transplantation. Directed NTM therapy should be initiated and continued in the post-transplant period, although the duration of post-transplant therapy is not well defined.
Recommended antibiotic therapy for the most common causes of pulmonary infections is outlined below. For details regarding therapy of other NTM infections, the reader is referred to the ATS consensus statement (16). Monitoring patients for signs of drug toxicity is required during therapy (Table 2). Attention to adequate nutritional intake and weight stability is important as gastrointestinal side-effects, such as nausea and vomiting, are commonly seen in conjunction with the macrolide agents, rifampin, and rifabutin. It is especially important that weight is monitored in patients with nodular bronchiectasis given the propensity of these patients to be quite thin.
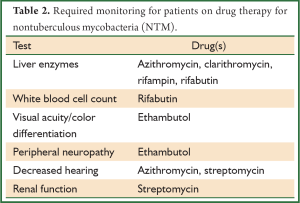
Full table
M. avium
Newer macrolide drugs such as azithromycin and clarithromycin are central to drug therapy for MAC lung infections. These agents demonstrate in vitro and clinical activity (62) against MAC, and are able to achieve penetration into phagocytes and tissue (63). It is imperative that these agents not be used in isolation due to the substantial possibility of the development of resistance. Combination drug therapy with a macrolide (azithromycin or clarithromycin), rifampin or rifabutin, and ethambutol with or without an intravenous aminoglycoside are recommended. Therapy should be continued for at least one year after conversion of sputum cultures from positive to negative (16). The duration of antimicrobial therapy commonly exceeds 18 months or more. In patients with nodular bronchiectasis, thrice weekly “triple therapy” with a macrolide, ethambutol, and rifamycin is typically recommended over daily therapy to improve drug tolerability and decrease costs. Aminoglycoside therapy is typically not required. Sequential introduction of the drugs over a few weeks may improve patient tolerability. For patients with fibrocavitary disease, previously treated disease, or severe disease daily therapy with the inclusion of either streptomycin or amikacin to the aforementioned agents is recommended. Success, defined as sustained eradication of the organism without relapse for several years after the discontinuation of therapy, is only achieved in 55% of patients treated with a macrolide based regimen (15). The duration of therapy, the suboptimal efficacy, and morbidity of drug toxicity often leads to reluctance to pursue treatment or discontinuation of therapy. In a meta-analysis of studies examining the efficacy of a macrolide containing antibiotic regimen, the patient dropout rate ranged from 11-33% (15). Furthermore, even after completion of recommended therapy, many patients will grow MAC organisms again and require repeated prolonged courses of therapy. In a study of 34 patients treated for 18 months with combination drug therapy, 11/34 (32%) grew MAC in sputum one year following discontinuation of therapy (64). Early reoccurrence of positive cultures suggests relapsed disease, whereas as later reoccurrence suggests a new infection.
Recent evidence suggests that macrolide antibiotics may decrease exacerbations and sputum density in patients with bronchiectasis (65,66). It is known that MAC infection following successful MAC therapy treatment with a macrolide containing regimen is associated with subsequent macrolide resistant MAC (67). Thus, there is great concern that the use of macrolides in patients with bronchiectasis may render subsequent NTM infections resistant to therapy.
M. Kansasii
Prolonged triple drug therapy including isoniazid (INH), rifampin, and ethanbutol is recommended for treatment of M. kansasii infections. Therapy should be continued for 12 months after sputum conversion to negative. Macrolides such as clarithromycin and the fourth generation fluoroquinolone moxifloxacin demonstrate very good in vitro activity against M. kansasii and may be an alternative to INH (68).
M. Abscessus
Lung infections due to M. abscessus are notoriously difficult to treat successfully with drug therapy alone. Chemotherapy in conjunction with surgical resection is often needed in those who can tolerate it. It is important that laboratories specifically differentiate infections with M. abscessus, as opposed to reporting a recovered organism as M. chelonae/abscessus, as the potential for success with drug therapy differs.
The role of surgery in NTM infections
The role of surgical intervention in patients with NTM infections has been infrequently studied. Thus, the historical roles of surgery in the management of TB, including diagnostic assistance, an adjuvant treatment option, and the management of complications of the disease have been extrapolated to patients with NTM infections. However, the public health implications for TB clearly differ from NTM. Surgical control of the disease is important in TB on both an individual and societal level because of the substantial risk of disease transmission to other patients and the development of multidrug resistance. These issues do not pertain to NTM.
The optimal procedure, the timing of surgical intervention, the value of debulking the most diseased areas, and expected morbidity and mortality of surgery for NTM infections remain as incompletely answered questions.
Assistance in making the diagnosis
NTM can present with various radiographic patterns including a diffuse ground-glass infiltrate, nodules, and mosaic attenuation consistent with patchy air trapping. Given the lack of specificity of these radiographic findings, surgical biopsies or resections to identify their etiology may fortuitously establish a diagnosis of NTM. An appreciation of the potential for an underlying infectious etiology of radiographic abnormalities highlights the importance of culturing explanted tissue removed at an operative procedure, if a benign diagnosis is provided (69).
The need for medical therapy after a surgical resection identifying NTM is dependent upon the extent of remaining disease, the immune status of the patient, and the pathogenicity of the organism isolated. Patients with remaining areas of infection nodules will presumably benefit from medical treatment post-operatively. The role of post-operative medical therapy and the duration of therapy in patients who have had resection of an isolated solitary nodule are uncertain.
The role of surgery in the management of NTM pulmonary infections
Given the suboptimal outcomes of medical therapy for NTM lung disease (15,70). Surgical resection is considered in selected individuals. However, the role of surgery is not definitively established. The severity and geographical distribution of the disease, responsiveness to antimicrobial therapy, and pulmonary reserve all influence the decision to pursue surgical management. Ideally, positive sputum needs to be converted and remain negative for at least three months if the infecting organism is susceptible to medical therapy. Optimization of bronchial hygiene with chest physical therapy or mechanical devices is recommended pre-operatively and post-operatively. Some authors recommend resected specimens be “double cultured’ with samples sent to two different microbiology laboratories to minimize sampling error (71). Pre-operative or intraoperative bronchoscopy is performed to exclude endobronchial pathology when suspected. Surgical resection is typically indicated in the setting of focal persistent disease amenable to complete anatomical resection. In more diffuse disease, surgery may be indicated to reduce disease burden and systemic symptoms of chronic infection in selected patients. There are not clearly established criteria upon which to recommend surgery in these cases.
Surgical technique
The largest experience with resection for atypical mycobacteria is reported from Denver, USA. These authors have reported both open thoracotomy and video-assisted thoracoscopic surgery (VATS) approaches to pulmonary resection with good results (71). However, in many instances the disease incites an inflammatory response, which obliterates the pleura, making a VATS approach difficult.
Mitchell and colleagues from Denver appear to have a low tolerance for use of muscle flaps (Latissimus dorsi) or omentum to fill large spaces or buttress the bronchial stump. One of the present author’s (JAO) approaches is to perform a muscle-sparing thoracotomy for any disease process where the risk of bronchopleural fistula and/or empyema is increased. In many instances, a muscle flap is not needed and is preserved and available for use, but only if necessary. The author’s experience advocates that dissection should proceed in the intrapleural plane rather than the sometimes easier extrapleural plane, because of excessive bleeding from myriads of small vessels. Lymph node and other tissue surrounding the bronchus should be retained and utilized to cover the bronchial stump. There exists a belief that resection for TB is associated with a high incidence of bronchopleural fistula, but in truth, this complication is infrequent (72).
If a pneumonectomy is performed, drainage is avoided if possible, recognizing that the risk of empyema increases with the duration of the presence of a chest tube. In those with persistent oozing or bleeding, a chest tube is necessary, but should be removed as soon as drainage becomes serous.
In patients with TB, the ipsilateral lung remaining after resection may be abnormal and fibrotic and may not completely fill the thoracic cavity. Options in these circumstances are to do nothing, with the anticipation and hope that in the post-operative period the space will become obliterated. Pleural tents are often not possible if there have been extensive adhesions that have been freed. In these instances where pleural space problems are anticipated, a multihole catheter alongside the phrenic nerve, with local anesthetic infused so that the hemidiaphragm is temporarily paralyzed is indicated.
Results of surgery
In the Denver experience, the overall operative mortality was low at 2.6%. Eleven patients developed a bronchopleural fistula (4.2%), of whom 10 had positive sputums at the time of surgery (71). In contradistinction to the experience with TB, where left pneumonectomy is undertaken twice as commonly as right pneumonectomy, right pneumonectomy is more commonly performed for atypical mycobacteria (71). Bronchopleural fistulae occurred after right pneumonectomy (71). A similarly high incidence of bronchopleural fistula after right pneumonectomy was also reported by Shiraishi and coworkers (73). These reports suggest that prophylactic muscle flaps are likely necessary in this patient sub group.
In a recent series of 110 patients who underwent surgical resection for right middle lobe or lingual bronchiectasis from NTM, 84% had negative post-operative cultures and smears (74). Eight of the negative patients subsequently became positive again, representing either relapse or reinfection. Sixteen percent did not convert, suggesting failure of surgical therapy. Therefore, 22% in total (24/110) remained positive (74). Although some may suggest these data argue unfavorably against surgical resection, it must be highlighted that symptoms of chronic cough and a feeling of being unwell were relieved in most. The impact of removal of the bulk of disease in those with persistent or subsequent positive sputum cultures on future health is not established, although, excision of a large focus of infection may facilitate medical management of remaining sites of disease (75).
The role of surgery in control of complications of NTM infections
In addition to surgery for control of the disease, surgery may be necessary to deal with complications of disease such as hemoptysis, cavitation with or without fungal ball formation, and empyema. These situations often require urgent treatment precluding the luxury of pre-operative testing, antibacterial therapy, and optimization of the patient’s cardiopulmonary and nutritional status.
NTM skin and soft tissue infection
NTM may infect surgical wounds post-operatively. The etiology of these infections is unknown, but it is most likely due to direct implantation or contamination of surgical instruments. Both host and organism characteristics are contributory, as these infections occur more commonly in those who are immunosuppressed, and the causative organisms are usually rapidly growing NTM.
The author (JAO) has noticed some characteristics of these wounds. They tend to appear very clean with minimal slough, and pus does not exude. If there is a liquid component to the wound, it is of low volume, clear, and watery. Granulation tissue is not abundant. The patient may have pain out of proportion to the extent of the wound and, in these patients, magnetic resonance imaging (MRI) or CT scans may identify deep tissue involvement. Curiously, some patients have minimal pain. Under normal circumstances, healing would be expected, as these wounds appear quite clean, but this does not readily occur despite adjunctive therapies such as the use of a wound vac or other similar device. Once the diagnosis is established with appropriate culturing, healing occurs with the institution of appropriate antimicrobial therapy.
Summary
The incidence of NTM infections surpasses that of TB infections in developed countries. Although infection may occur in virtually any organ, pulmonary infections are most common. M. avium, M. kansasii, and M. abscessus are the most frequently identified organisms causing lung disease. The isolation of an NTM organism does not necessarily equate with active infection; clinical, radiologic, and microbiologic parameters are all needed to establish the diagnosis of infection. Eradication of disease with drug therapy requires prolonged combination therapy. Surgical resection is often indicated in localized disease, in the presence of drug resistant organisms, or in some cases, of failure of medical therapy.
There remain significant gaps in our knowledge of the acquisition and management of NTM pulmonary infections. Susceptibility to disease is incompletely understood and, thus, it is unclear what preventative measures may be effective. Additionally, given the difficulty of eradicating NTM and its substantial re-occurrence, identifying appropriate candidates for treatment and the timing of initiation of therapy are clinically difficult decisions. A better understanding of the natural history of untreated MAC infection in association with nodular bronchiectasis, the identification of markers for disease progression, and improved understanding of the risk factors for re-infection would be of substantial help to clinicians and patients. Additionally, there exists a substantial need for improved pharmacotherapy for M. abscessus.
Acknowledgements
Disclosure: The authors declare no conflict of interest.
References
- Chan ED, Iseman MD. Underlying host risk factors for nontuberculous mycobacterial lung disease. Semin Respir Crit Care Med 2013;34:110-23. [PubMed]
- Ray CG, Ryan KJ. eds. Sherris Medical Microbiology, 4th Edition. McGraw-Hill Medical, 2004.
- Jarlier V, Nikaido H. Mycobacterial cell wall: structure and role in natural resistance to antibiotics. FEMS Microbiol Lett 1994;123:11-8. [PubMed]
- Schulze-Röbbecke R, Janning B, Fischeder R. Occurrence of mycobacteria in biofilm samples. Tuber Lung Dis 1992;73:141-4. [PubMed]
- Falkinham JO 3rd. Growth in catheter biofilms and antibiotic resistance of Mycobacterium avium. J Med Microbiol 2007;56:250-4. [PubMed]
- Parker BC, Ford MA, Gruft H, et al. Epidemiology of infection by nontuberculous mycobacteria. IV. Preferential aerosolization of Mycobacterium intracellulare from natural waters. Am Rev Respir Dis 1983;128:652-6. [PubMed]
- Bodmer T, Miltner E, Bermudez LE. Mycobacterium avium resists exposure to the acidic conditions of the stomach. FEMS Microbiol Lett 2000;182:45-9. [PubMed]
- Kirschner RA Jr, Parker BC, Falkinham JO 3rd. Epidemiology of infection by nontuberculous mycobacteria. Mycobacterium avium, Mycobacterium intracellulare, and Mycobacterium scrofulaceum in acid, brown-water swamps of the southeastern United States and their association with environmental variables. Am Rev Respir Dis 1992;145:271-5. [PubMed]
- Falkinham JO 3rd. Ecology of nontuberculous mycobacteria--where do human infections come from? Semin Respir Crit Care Med 2013;34:95-102. [PubMed]
- Phillips MS, von Reyn CF. Nosocomial infections due to nontuberculous mycobacteria. Clin Infect Dis 2001;33:1363-74. [PubMed]
- Falkinham JO 3rd. Nontuberculous mycobacteria from household plumbing of patients with nontuberculous mycobacteria disease. Emerg Infect Dis 2011;17:419-24. [PubMed]
- Falkinham JO 3rd. Hospital water filters as a source of Mycobacterium avium complex. J Med Microbiol 2010;59:1198-202. [PubMed]
- Feazel LM, Baumgartner LK, Peterson KL, et al. Opportunistic pathogens enriched in showerhead biofilms. Proc Natl Acad Sci U S A 2009;106:16393-9. [PubMed]
- Tortoli E. Impact of genotypic studies on mycobacterial taxonomy: the new mycobacteria of the 1990s. Clin Microbiol Rev 2003;16:319-54. [PubMed]
- Field SK, Fisher D, Cowie RL. Mycobacterium avium complex pulmonary disease in patients without HIV infection. Chest 2004;126:566-81. [PubMed]
- Griffith DE, Aksamit T, Brown-Elliott BA, et al. An official ATS/IDSA statement: diagnosis, treatment, and prevention of nontuberculous mycobacterial diseases. Am J Respir Crit Care Med 2007;175:367-416. [PubMed]
- Yates MD, Grange JM, Collins CH. The nature of mycobacterial disease in south east England, 1977-84. J Epidemiol Community Health 1986;40:295-300. [PubMed]
- Pulmonary disease caused by M. malmoense in HIV negative patients: 5-yr follow-up of patients receiving standardised treatment. Eur Respir J 2003;21:478-82. [PubMed]
- Jenkins PA, Campbell IA. Pulmonary disease caused by Mycobacterium xenopi in HIV-negative patients: five year follow-up of patients receiving standardised treatment. Respir Med 2003;97:439-44. [PubMed]
- von Reyn CF, Waddell RD, Eaton T, et al. Isolation of mycobacterium avium complex from water in the United States, Finland, Zaire, and Kenya. J Clin Microbiol 1993;31:3227-30. [PubMed]
- Cassidy PM, Hedberg K, Saulson A, et al. Nontuberculous mycobacterial disease prevalence and risk factors: a changing epidemiology. Clin Infect Dis 2009;49:e124-9. [PubMed]
- Winthrop KL, Mcnelley E, Kendall B, et al. Pulmonary nontuberculous mycobacterial disease prevalence and clinical features: an emerging public health disease. Am J Respir Crit Care Med 2010;182:977-82. [PubMed]
- Kendall BA, Winthrop KL. Update on the epidemiology of pulmonary nontuberculous mycobacterial infections. Semin Respir Crit Care Med 2013;34:87-94. [PubMed]
- Adjemian J, Olivier KN, Seitz AE, et al. Prevalence of nontuberculous mycobacterial lung disease in U.S. Medicare beneficiaries. Am J Respir Crit Care Med 2012;185:881-6. [PubMed]
- Prevots DR, Shaw PA, Strickland D, et al. Nontuberculous mycobacterial lung disease prevalence at four integrated health care delivery systems. Am J Respir Crit Care Med 2010;182:970-6. [PubMed]
- Winthrop KL, Varley CD, Ory J, et al. Pulmonary disease associated with nontuberculous mycobacteria, Oregon, USA. Emerg Infect Dis 2011;17:1760-1. [PubMed]
- Maekawa K, Ito Y, Hirai T, et al. Environmental risk factors for pulmonary Mycobacterium avium-intracellulare complex disease. Chest 2011;140:723-9. [PubMed]
- Edwards LB, Acquaviva FA, Livesay VT, et al. An atlas of sensitivity to tuberculin, PPD-B, and histoplasmin in the United States. Am Rev Respir Dis 1969;99:Suppl:1-132. [PubMed]
- O’Brien RJ, Geiter LJ, Snider DE Jr. The epidemiology of nontuberculous mycobacterial diseases in the United States. Results from a national survey. Am Rev Respir Dis 1987;135:1007-14. [PubMed]
- Horsburgh CR Jr, Selik RM. The epidemiology of disseminated nontuberculous mycobacterial infection in the acquired immunodeficiency syndrome (AIDS). Am Rev Respir Dis 1989;139:4-7. [PubMed]
- Bloch KC, Zwerling L, Pletcher MJ, et al. Incidence and clinical implications of isolation of Mycobacterium kansasii: results of a 5-year, population-based study. Ann Intern Med 1998;129:698-704. [PubMed]
- CLSI: Susceptibility Testing of Mycobacteria, Nocardiae, and Other Aerobic Actinomycetes; Approved Standard. CLSI document M24-A (ISBN 1-56238-550-3). CLSI, Wayne, PA, 2003.
- Herold CD, Fitzgerald RL, Herold DA. Current techniques in mycobacterial detection and speciation. Crit Rev Clin Lab Sci 1996;33:83-138. [PubMed]
- Kankya C, Muwonge A, Djønne B, et al. Isolation of non-tuberculous mycobacteria from pastoral ecosystems of Uganda: public health significance. BMC Public Health 2011;11:320. [PubMed]
- Wolinsky E. Mycobacterial lymphadenitis in children: a prospective study of 105 nontuberculous cases with long-term follow-up. Clin Infect Dis 1995;20:954-63. [PubMed]
- Falkinham JO 3rd, Iseman MD, de Haas P, et al. Mycobacterium avium in a shower linked to pulmonary disease. J Water Health 2008;6:209-13. [PubMed]
- Falkinham JO 3rd. Factors influencing the Chlorine susceptibility of Mycobacterium avium, Mycobacterium intracellulare, and Mycobacterium scrofulaceum. Appl Environ Microbiol 2003;69:5685-9. [PubMed]
- De Groote MA, Pace NR, Fulton K, et al. Relationships between Mycobacterium isolates from patients with pulmonary mycobacterial infection and potting soils. Appl Environ Microbiol 2006;72:7602-6. [PubMed]
- Dirac MA, Horan KL, Doody DR, et al. Environment or host?: A case-control study of risk factors for Mycobacterium avium complex lung disease. Am J Respir Crit Care Med 2012;186:684-91. [PubMed]
- Mandel AS, Sprauer MA, Sniadack DH, et al. State regulation of hospital water temperature. Infect Control Hosp Epidemiol 1993;14:642-5. [PubMed]
- Horsburgh CR Jr. Epidemiology of disease caused by nontuberculous mycobacteria. Semin Respir Infect 1996;11:244-51. [PubMed]
- Olivier KN, Weber DJ, Wallace RJ Jr, et al. Nontuberculous mycobacteria. I: multicenter prevalence study in cystic fibrosis. Am J Respir Crit Care Med 2003;167:828-34. [PubMed]
- Baldi S, Rapellino M, Ruffini E, et al. Atypical mycobacteriosis in a lung transplant recipient. Eur Respir J 1997;10:952-4. [PubMed]
- Piersimoni C. Nontuberculous mycobacteria infection in solid organ transplant recipients. Eur J Clin Microbiol Infect Dis 2012;31:397-403. [PubMed]
- Knoll BM, Kappagoda S, Gill RR, et al. Non-tuberculous mycobacterial infection among lung transplant recipients: a 15-year cohort study. Transpl Infect Dis 2012;14:452-60. [PubMed]
- Huang HC, Weigt SS, Derhovanessian A, et al. Non-tuberculous mycobacterium infection after lung transplantation is associated with increased mortality. J Heart Lung Transplant 2011;30:790-8. [PubMed]
- Roux AL, Catherinot E, Ripoll F, et al. Multicenter study of prevalence of nontuberculous mycobacteria in patients with cystic fibrosis in France. J Clin Microbiol 2009;47:4124-8. [PubMed]
- Hill UG, Floto RA, Haworth CS. Non-tuberculous mycobacteria in cystic fibrosis. J R Soc Med 2012;105 Suppl 2:S14-8. [PubMed]
- Dorman SE, Holland SM. Interferon-gamma and interleukin-12 pathway defects and human disease. Cytokine Growth Factor Rev 2000;11:321-33. [PubMed]
- Winthrop KL, Chang E, Yamashita S, et al. Nontuberculous mycobacteria infections and anti-tumor necrosis factor-alpha therapy. Emerg Infect Dis 2009;15:1556-61. [PubMed]
- Winthrop KL, Baxter R, Liu L, et al. Mycobacterial diseases and antitumour necrosis factor therapy in USA. Ann Rheum Dis 2013;72:37-42. [PubMed]
- Kim RD, Greenberg DE, Ehrmantraut ME, et al. Pulmonary nontuberculous mycobacterial disease: prospective study of a distinct preexisting syndrome. Am J Respir Crit Care Med 2008;178:1066-74. [PubMed]
- Pryor M, Springthorpe S, Riffard S, et al. Investigation of opportunistic pathogens in municipal drinking water under different supply and treatment regimes. Water Sci Technol 2004;50:83-90. [PubMed]
- Bandoh S, Fujita J, Ueda Y, et al. Uptake of fluorine-18-fluorodeoxyglucose in pulmonary Mycobacterium avium complex infection. Intern Med 2003;42:726-9. [PubMed]
- Prince DS, Peterson DD, Steiner RM, et al. Infection with Mycobacterium avium complex in patients without predisposing conditions. N Engl J Med 1989;321:863-8. [PubMed]
- Iseman MD, Buschman DL, Ackerson LM. Pectus excavatum and scoliosis. Thoracic anomalies associated with pulmonary disease caused by Mycobacterium avium complex. Am Rev Respir Dis 1991;144:914-6. [PubMed]
- Cappelluti E, Fraire AE, Schaefer OP. A case of “hot tub lung” due to Mycobacterium avium complex in an immunocompetent host. Arch Intern Med 2003;163:845-8. [PubMed]
- Rickman OB, Ryu JH, Fidler ME, et al. Hypersensitivity pneumonitis associated with mycobacterium avium complex and hot tub use. Mayo Clin Proc 2002;77:1233-7. [PubMed]
- Lacasse Y, Girard M, Cormier Y. Recent advances in hypersensitivity pneumonitis. Chest 2012;142:208-17. [PubMed]
- Barber CM, Burton CM, Robinson E, et al. Hypersensitivity pneumonitis due to metalworking fluid exposures. Chest 2013;143:1189. [PubMed]
- Kikuchi T, Watanabe A, Gomi K, et al. Association between mycobacterial genotypes and disease progression in Mycobacterium avium pulmonary infection. Thorax 2009;64:901-7. [PubMed]
- Wallace RJ Jr, Brown BA, Griffith DE, et al. Clarithromycin regimens for pulmonary Mycobacterium avium complex. The first 50 patients. Am J Respir Crit Care Med 1996;153:1766-72. [PubMed]
- Eisenberg E, Barza M. Azithromycin and clarithromycin. Curr Clin Top Infect Dis 1994;14:52-79. [PubMed]
- Hasegawa N, Nishimura T, Ohtani S, et al. Therapeutic effects of various initial combinations of chemotherapy including clarithromycin against Mycobacterium avium complex pulmonary disease. Chest 2009;136:1569-75. [PubMed]
- Altenburg J, de Graaff CS, Stienstra Y, et al. Effect of azithromycin maintenance treatment on infectious exacerbations among patients with non-cystic fibrosis bronchiectasis: the BAT randomized controlled trial. JAMA 2013;309:1251-9. [PubMed]
- Serisier DJ, Martin ML, Mcguckin MA, et al. Effect of long-term, low-dose erythromycin on pulmonary exacerbations among patients with non-cystic fibrosis bronchiectasis: the BLESS randomized controlled trial. JAMA 2013;309:1260-7. [PubMed]
- Heifets LB, Iseman MD. Individualized therapy versus standard regimens in the treatment of Mycobacterium avium infections. Am Rev Respir Dis 1991;144:1-2. [PubMed]
- Alcaide F, Calatayud L, Santín M, et al. Comparative in vitro activities of linezolid, telithromycin, clarithromycin, levofloxacin, moxifloxacin, and four conventional antimycobacterial drugs against Mycobacterium kansasii. Antimicrob Agents Chemother 2004;48:4562-5. [PubMed]
- Khoor A, Leslie KO, Tazelaar HD, et al. Diffuse pulmonary disease caused by nontuberculous mycobacteria in immunocompetent people (hot tub lung). Am J Clin Pathol 2001;115:755-62. [PubMed]
- Philley JV, Griffith DE. Management of nontuberculous mycobacterial (NTM) lung disease. Semin Respir Crit Care Med 2013;34:135-42. [PubMed]
- Mitchell JD, Bishop A, Cafaro A, et al. Anatomic lung resection for nontuberculous mycobacterial disease. Ann Thorac Surg 2008;85:1887-92; discussion 1892-3.
- Pomerantz M, Madsen L, Goble M, et al. Surgical management of resistant Mycobacterial tuberculosis and other mycobacterial pulmonary infections. Ann Thorac Surg 1991;52:1108-11; discussion 1112. [PubMed]
- Shiraishi Y, Nakajima Y, Katsuragi N, et al. Pneumonectomy for nontuberculous mycobacterial infections. Ann Thorac Surg 2004;78:399-403. [PubMed]
- Yu JA, Pomerantz M, Bishop A, et al. Lady Windermere revisited: treatment with thoracoscopic lobectomy/segmentectomy for right middle lobe and lingular bronchiectasis associated with non-tuberculous mycobacterial disease. Eur J Cardiothorac Surg 2011;40:671-5. [PubMed]
- Watanabe M, Hasegawa N, Ishizaka A, et al. Early pulmonary resection for mycobacterium avium complex lung disease treated with macrolides and quinolones. Ann Thorac Surg 2006;81:2026-30. [PubMed]