The evaluation of different treatment protocols for trauma-induced lung injury in rats
Introduction
Traumas injury are the leading cause of death for young people (1). Blunt chest trauma (BCT), responsible for 25% of trauma-related deaths, is seen in patients with approximately 10% (1-3). The most common type of injury in BCT, which is often associated with other systemic injuries, is pulmonary contusion; its incidence varies between 17% and 25% (3,4).
BCT-induced lung injury depends on trauma severity and alveolocapillar membrane damage (4,5). A better understanding of the pathophysiological mechanisms and cellular events in lung contusion can help in determining the prognosis and treatment methods for this important clinical condition (4). Therefore, many studies have been performed to clarify the pathophysiological mechanisms, cellular changes, and inflammatory processes occuring in lung consiont (4-8).
Imbalance between defense mechanisms involving antioxidants such as superoxide dismutase (SOD), catalase, and glutathione peroxidase with free radical formation and increased levels of oxygen metabolites during inflammatory processes play an important role in lung damage. In other words, oxidative stress is an important pathophysiological event leading to tissue damage (9,10). The end product of lipid peroxidation, thiobarbituric acid reactive substances (TBARS), is used as a common marker of oxidative stress (11). Several studies have indicated that a significant relationship exists between TBARS levels and the extent of tissue damage (12,13). Moreover, Clara Cell Protein-16 (CC-16) is also a marker of acute lung injury (ALI). This protein which is secreted from the tracheobronchial tree and particularly from the terminal bronchioles plays role as an anti-inflammatory effect on lung tissue after inflammatuar process (10).
In these previous studies, effective anti-inflammatory agents preventing and/or reducing inflammation in lung tissues and medicines with antioxidant and histological healing effects on damaged tissues were used for the treatment of ALI. Steroids are known as the strongest anti-inflammatory agents; they function via intracellular receptors by affecting prostaglandin synthesis (14). In particular, administration of high doses of steroids in the early stage of lung injury was found to be effective (15). Another therapeutic agent with anti-inflammatory and antioxidant properties is quercetin (Q). Several studies have shown that Q prevents tissue damage because of its specific effect on the pathophysiological processes induced by free radical formation (16-18).
The purpose of this study is to determine the effects of the antioxidant and anti-inflammatory properties of Q and methylprednisolone (MP) on damaged lung tissue in a lung contusion model of rats and evaluate whether their combined use improves treatment efficacy.
Materials and methods
Experimental protocol
This study was approved by the Ethics Committee of Ondokuz Mayis University for Experimental Animal Studies. A total of 35 healthy female Sprague Dawley rats weighing 250-300 g were included. Food and tap water were provided ad libitum. All rats were kept in windowless animal quarters with temperature automatically maintained at 24 °C and controlled lighting (12 h light/12 h dark cycle) and humidity (55-60%).
The rats were divided into five groups as follows: Sham (control); BCT; BCT + MP, BCT group treated with i.p. MP (20 mg Prednol-L; Mustafa Nevzat, Turkey); BCT + Q, BCT group that received oral gavage administration of Q (Sigma Chemical Co., St. Louis, MO, USA); and BCT + MP + Q, BCT group treated with MP and Q. Each group had seven animals.
The rats in the BCT groups were anesthetized with ketamine hydrochloride (100 mg/kg, i.p.) and xylazine (10 mg/kg, i.p.) and subjected to chest trauma with 1.96 J of impact energy as described by Raghavendran K. et al. (4). The impact energy (E) of the falling weight was calculated from the following equation: E = m (0.4 kg) × g (9.8 m/s2) × h (50 cm) (19). The rats in the BCT + MP group were injected with MP i.p. once a day (30 mg/kg injected 5 min after the trauma on day 1 and 3 mg/kg from days 2 to 7) (15). The rats in the BCT + Q group were administered Q once a day orally (50 mg/kg administered 5 min after the trauma on day 1 and 50 mg/kg from days 2 to 7) (20). The rats in the BCT + MP + Q group were administered the same doses of MP and Q for the same duration. All rats were kept under observation until they recovered from the experimental procedure.
After seven days of treatment, all rats were killed with i.p. ketamine hydrochloride and xylazine injections. Their lungs were removed from the thorax for histopathological and immunohistochemical analyses.
Histopathological evaluation
p>Lung tissue samples were immersed in neutral-buffered 10% formalin solution for 24 h and then embedded in paraffin. For histopathological analysis, 5-μm-thick tissue sections were stained with the hematoxylin-eosin (HE) stain. Pathologists analyzing the samples were blinded, and the samples from all study groups were examined microscopically to determine the extent of peribronchial inflammatory cell infiltration (PICI), alveolar septal infiltration (ASI), alveolar edema (AED), alveolar exudate (AEX), alveolar histiocytes (AHI), and interstitial fibrosis (IF) formation using a 4-point scale (Table 1) (21).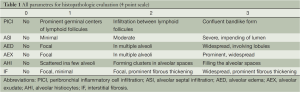
Full table
Biochemical analysis
Sample preparation
After the rats were sacrificed, blood samples were collected in sterile test tubes. Whole blood was allowed to clot at room temperature for 30 min. Then, the samples were centrifuged at 3,000 ×g for ten minutes at 4 °C. Following centrifugation, the serum was removed and transfered into a clean tube. All samples were stored at –80 °C until analysis. A day before the study, all samples were dissolved at 2-8 °C.
Measurement of serum CC-16 levels
CC-16 levels were determined using a sandwich ELISA plate as per the manufacturer’s instructions (Uscn Life Science Inc., Wuhan, China). The plate was pre-coated with an antibody specific to CC-16. Then, samples with a biotin-conjugated antibody specific to CC-16 were added to the wells. Next, avidin-conjugated to horseradish peroxidase was added to the wells and the plate was incubated. After the TMB substrate solution was added, the enzyme-substrate reaction was terminated by adding sulphuric acid solution, and the CC-16 levels were determined colorimetrically at 450 nm. The concentration of CC-16 concentration was then determined from the standard curve, and the results have been presented in picograms per milliliter.
Measurement of serum TBARS levels
TBARS were measured using the TBARS Assay Kit (Catalog No. 10009055, Cayman Chemical Company, Ann Arbor, MI, USA). The principle of the test is the formation of the Malondialdehyde-Thiobarbituric acid (MDA-TBA) adduct by the reaction of MDA and TBA under high temperature (90-100 °C) and acidic conditions. The concentration of the MDA-TBA adduct was measured colorimetrically at 530 and 540 nm. The results have been presented in micromoles per liter.
Measurement of serum SOD levels
SOD levels were measured using the Superoxide Dismutase Assay Kit (Catalog No. 706002, Cayman Chemical Company, Ann Arbor, MI, USA). The principle of the test is based on the utilization of a tetrazolium salt for the detection of superoxide radicals generated by xanthine oxidase and hypoxanthine. One unit of SOD is defined as the amount of enzyme needed to cause 50% dismutation of superoxide radicals. The results have been presented in units per milliliter.
Statistical analysis
Biochemical results and histopathological scores were analyzed using IBM SPSS 21.0 for Windows. The results were presented as median (minimum/maximum) or mean ± standard deviation. All values were evaluated with the non-parametric Mann-Whitney U test. Differences were considered significant at P
Results
Biochemical examination
Increased serum CC-16 and TBARS levels were found in the BCT group compared to the Sham group (P=0.001 and P=0.001, respectively) (Table 2). However, serum SOD levels were decreased in the BCT group compared to the Sham group (P=0.001). With MP and Q treatment, serum CC-16 levels were decreased in the BCT group compared to the Sham group, but only the BCT + MP group showed a significant difference (P0.05). Serum TBARS levels were significantly lower in the BCT + MP and BCT + Q group than the BCT group (P=0.001 and P=0.001, respectively). However, serum SOD levels were not significantly difference between these treatment groups (BCT + MP and BCT + Q) (Table 2). In the BCT + MP + Q group, serum CC-16 and TBARS levels were decreased compared to the BCT group (P=0.001 and P0.01, respectively). On the other hand, serum SOD levels were the highest in the BCT + MP + Q group among the BCT groups (PTable 2). The combined therapy regimen significantly decreased CC-16 levels compared to the individual treatments with MP and Q (P0.01 and P=0.001, respectively). Similarly, combined MP and Q treatment significantly increased the serum SOD levels compared to the other two treatment groups (P0.01 and P0.05, respectively) (Table 2). Serum TBARS levels were not significantly different in the BCT + MP + Q group compared to the other treatment groups (Table 2).
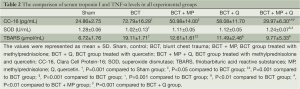
Full table
Histopathological findings
Images of histopathological sections from all study groups are shown in Figure 1. All histopathological scores including PICI, ASI, AED, AEX, AHI, and IF were higher in the BCT group than in the Sham group (P0.01, P=0.001, P=0.001, P0.01, P0.01, and P=0.001, respectively) (Figures 1,2).
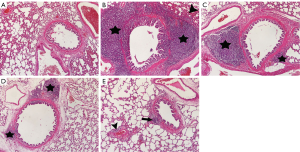
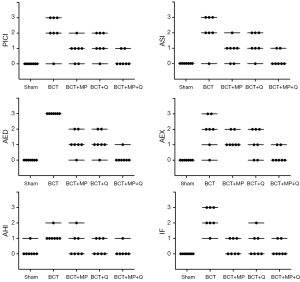
The MP caused a significant decrease in the PICI, ASI, AED, and IF scores compared to the BCT group (P0.05, P0.05, P=0.001, and P=0.001, respectively) (Figures 1,2). However, Q treatment only caused a significant decrease in the AED and IF scores in BCT + Q group compared to the BCT group (P=0.001 and P0.01, respectively) (Figure 2). On the other hand, combined MP and Q combined treatment caused a significant decrease in the scores of all the above histopathological parameters (Figure 2).
Compared to all treatment groups; the combined treatment group (BCT + MP + Q) showed significantly lower AED and AEX scores compared to the BCT+MP group (P0.05 and P0.05, respectively) (Figure 2). In addition, PICI and AED scores in combined therapy group showed significantly lower than the BCT + Q group (P0.05 and P0.05, respectively). There was no significant difference in the histopathological scores between the BCT + MP and BCT + Q groups (Figure 2).
Discussion
In this study, the effects of the antioxidant and anti-inflammatory properties of Q and MP on damaged lung tissue in a lung contusion model of rats were and evaluated. Combined MP and Q treatment significantly reduced AED and AEX formation, which is particularly important in the progression of ALI to acute respiratory distress syndrome (ARDS) according to alone usage of MP. In addition, this combined therapy significantly reduced PICI and AED, which is regarded as an important marker of inflammatory reaction according to alone treatment of Q. Apart from its histopathological effectiveness, the ability of combined therapy to reduce serum CC-16 and to increase SOD levels are considered particularly effective in alleviating lung injury according to the other treatment groups.
ALI developing after BCT is among the most important factors that determine prognosis. This injury occurs in about 50% to 60% of patients with BCT (22). Today, despite the widespread availability of intensive care facilities and advanced respiratory devices, the BCT-related mortality rate is 15.5%, and BCT is the third most common cause of death among young people (23,24).
Lung contusion developing after BCT is a clinical condition accompanied by histopathological findings such as alveolar congestion, alveolar hemorrhage, AED, leukocyte infiltration and ventilation-perfusion defects caused by pathological changes, hypoxemia, hypercapnia caused by intrapulmonary shunt development and reduction in compliance, and increased respiratory workload with variable weight and duration (25,26). ARDS occurs because of the disruption of endothelial and epithelial cell functions as a result of lung injury developing after BCT. The most significant histopathological event in the lung tissues of ARDS patients is noncardiogenic high-permeable pulmonary edema (27). Alveolocapillar damage, occurring with fibrosis following structural disorder of type 2 alveolar pnomosit and increase in myofibroblasts after edema in the lungs, leads to ARDS (28). In our study, similar to the histopathological findings mentioned in the literature, there was a significant increase in the PICI, ASI, AED, AEX, AHI, and IF scores after BCT. We also found that MP and Q combination therapy was more effective than MP or Q alone in reducing AED, which is accepted as a trigger in the process leading to ARDS after lung contusion.
The underlying molecular and physiological changes in lung injury developing after BCT have still not been clearly elucidated. However, bacterial toxins often increase free oxygen radical release by activating inflammatory cells, proteases, and microembolies, thus leading to expansion of lung pores and increased permeability. Further, apoptosis causes pulmonary edema, which is accepted as the basic mechanism underlying ALI (27,29). Under normal physiological conditions, there is a balance between free oxygen radicals, which are effective in pathophysiology, and the antioxidant enzyme system (SOD, catalase, GSH-Px, glutathione, etc.). An imbalance between oxidants and antioxidants causes DNA and RNA damage due to oxidation of structural lipids, proteins, and polysaccharides at the cellular level (9). Increased TBARS (end product of lipid peroxidation) levels in the damaged tissue is recognized as a marker of oxidative damage (12,13). An experimental study performed by Mokra et al. showed that serum TBARS levels correlated with the severity of lung injury and increased significantly in lung injury occurring after meconium aspiration (30). Further, Goraca et al. demostrated that TBARS levels in the BAL fluid increased after oxidative stress and decreased after treatment with alpha-lipoic acid in one of their studies (31). In our study, we measured serum TBARS and SOD levels for determining the oxidant-antioxidant balance. Significant decrease was found in the level of serum SOD after BCT, while there was an increase in serum TBARS levels. We found that while MP and Q therapies when used alone did not cause significant changes in serum SOD level, they only caused significant increase in TBARS levels. We also found that the combined use of MP and Q was the most effective treatment for maintaining the balance of oxidants and antioxidants. This result demonstrated that the combined therapy was significantly effective in reducing oxidative damage and particularly effective in maintaining the oxidant-antioxidant balance.
CC-16 is a protein secreted from tracheobronchial tree and particularly from the terminal bronchioles where the Clara cells reside and plays a protective role against oxidative stress and inflammatory response in the respiratory tract (10,32). It is considered as a biomarker to determine the severity of lung injury (10,33). In a study performed by Wutzler et al., it has been reported that there was a significant correlation between the level of serum CC-16 and increased respiratory complications after chest trauma (33). In our study, in accordance with histopathological findings, significant increase in serum CC-16 levels were detected after contusion. Moreover, it was determined that the combined therapy of MP and Q decreased serum CC-16 level significantly compared to the other treatment groups.
The pathophysiological mechanism of lung contusion has not been clearly elucidated. Symptomatic and supportive approach is usually considered in the treatment of BCT. These treatment strategies are respiratory failure treatment, post-traumatic pain management, chest physiotherapy, and fluid support (34). In addition to these treatment strategies, medications such as antioxidants, steroids diuretics, and surfactants are used in reducing lung injury (3,5,34-36). The beneficial effect of steroid administration in lung injury has been found to reduce proinflammatory cytokine production, expression of leukocyte adhesion proteins, and prevention of excessive collagen accumulation in alveolar tissues because of antifibrotic activity (27). Some studies have reported that early administration of high-dose steroids after BCT significantly reduced lung injury and in-hospital mortality; the incidence of post-traumatic complications was also reduced in patients treated with steroids (37,38). Glucocorticoids and antioxidants were used together for treating lung and heart lesions in a variety of experimental studies (39,40).
In conclusion, high-dose MP therapy should be administered in the early stage of lung injury developed after BCT. The initial high-dose MP therapy should be maintained on lower doses. In addition, we suggest that the use of combined quercetin and this MP treatment regimen is more effective for treating lung injury after BCT.
Acknowledgements
Disclosure: The authors declare no conflict of interest.
References
- Kidher E, Krasopoulos G, Coats T, et al. The effect of prehospital time related variables on mortality following severe thoracic trauma. Injury 2012;43:1386-92. [PubMed]
- Markogiannakis H, Sanidas E, Messaris E, et al. Predictors of in-hospital mortality of trauma patients injured in vehicle accidents. Ulus Travma Acil Cerrahi Derg 2008;14:125-31. [PubMed]
- Hasbahçeci M, Ozpek A, Başak F, et al. Factors affecting mortality in blunt thoracic trauma. Ulus Travma Acil Cerrahi Derg 2013;19:127-32. [PubMed]
- Raghavendran K, Davidson BA, Helinski JD, et al. A rat model for isolated bilateral lung contusion from blunt chest trauma. Anesth Analg 2005;101:1482-9. [PubMed]
- Cohn SM. Pulmonary contusion: review of the clinical entity. J Trauma 1997;42:973-9. [PubMed]
- Obertacke U, Neudeck F, Majetschak M, et al. Local and systemic reactions after lung contusion: an experimental study in the pig. Shock 1998;10:7-12. [PubMed]
- Wang ND, Stevens MH, Doty DB, et al. Blunt chest trauma: an experimental model for heart and lung contusion. J Trauma 2003;54:744-8. [PubMed]
- Celik B, Yardan T, Kefeli M, et al. Diagnostic value of ischaemia-modified albumin in pulmonary contusion in rats. Injury 2012;43:357-61. [PubMed]
- Shim SY, Kim HS. Oxidative stress and the antioxidant enzyme system in the developing brain. Korean J Pediatr 2013;56:107-11. [PubMed]
- Alaçam H, Karli R, Alici O, et al. The effects of α-tocopherol on oxidative damage and serum levels of Clara cell protein 16 in aspiration pneumonitis induced by bile acids. Hum Exp Toxicol 2013;32:53-61. [PubMed]
- Davies KJ. Oxidative stress: the paradox of aerobic life. Biochem Soc Symp 1995;61:1-31. [PubMed]
- Gupta A, Singh RL, Raghubir R. Antioxidant status during cutaneous wound healing in immunocompromised rats. Mol Cell Biochem 2002;241:1-7. [PubMed]
- Rasik AM, Shukla A. Antioxidant status in delayed healing type of wounds. Int J Exp Pathol 2000;81:257-63. [PubMed]
- Chimalakonda AP, Mehvar R. Effects of methylprednisolone and its liver-targeted dextran prodrug on ischemia-reperfusion injury in a rat liver transplantation model. Pharm Res 2007;24:2231-8. [PubMed]
- Teng D, Pang QF, Yan WJ, et al. The harmful effect of prolonged high-dose methylprednisolone in acute lung injury. Int Immunopharmacol 2013;15:223-6. [PubMed]
- Casagrande R, Georgetti SR, Verri WA Jr, et al. Evaluation of functional stability of quercetin as a raw material and in different topical formulations by its antilipoperoxidative activity. AAPS PharmSciTech 2006;7:E10. [PubMed]
- Moskaug JØ, Carlsen H, Myhrstad M, et al. Molecular imaging of the biological effects of quercetin and quercetin-rich foods. Mech Ageing Dev 2004;125:315-24. [PubMed]
- Nijveldt RJ, van Nood E, van Hoorn DE, et al. Flavonoids: a review of probable mechanisms of action and potential applications. Am J Clin Nutr 2001;74:418-25. [PubMed]
- Miller PR, Croce MA, Bee TK, et al. ARDS after pulmonary contusion: accurate measurement of contusion volume identifies high-risk patients. J Trauma 2001;51:223-8; discussion 229-30. [PubMed]
- El-Sayed NS, Rizk SM. The protective effect of quercetin, green tea or malt extracts against experimentally-induced lung fibrosis in rats. Afr J Pharm Pharmacol 2009;3:191-201.
- Takil A, Umuroğlu T, Göğüş YF, et al. Histopathologic effects of lipid content of enteral solutions after pulmonary aspiration in rats. Nutrition 2003;19:666-9. [PubMed]
- Voeller GR, Reisser JR, Fabian TC, et al. Blunt diaphragm injuries. A five-year experience. Am Surg 1990;56:28-31. [PubMed]
- Wang ND, Stevens MH, Doty DB, et al. Blunt chest trauma: an experimental model for heart and lung contusion. J Trauma 2003;54:744-8. [PubMed]
- Voelckel WG, Raedler C, Wenzel V, et al. Arginine vasopressin, but not epinephrine, improves survival in uncontrolled hemorrhagic shock after liver trauma in pigs. Crit Care Med 2003;31:1160-5. [PubMed]
- Raghavendran K, Davidson BA, Woytash JA, et al. The evolution of isolated bilateral lung contusion from blunt chest trauma in rats: cellular and cytokine responses. Shock 2005;24:132-8. [PubMed]
- Oppenheimer L, Craven KD, Forkert L, et al. Pathophysiology of pulmonary contusion in dogs. J Appl Physiol 1979;47:718-28. [PubMed]
- Deal EN, Hollands JM, Schramm GE, et al. Role of corticosteroids in the management of acute respiratory distress syndrome. Clin Ther 2008;30:787-99. [PubMed]
- Kollef MH, Schuster DP. The acute respiratory distress syndrome. N Engl J Med 1995;332:27-37. [PubMed]
- Round table conference. Acute lung injury. Am J Respir Crit Care Med 1998;158:675-9. [PubMed]
- Mokra D, Drgova A, Pullmann R Sr, et al. Selective phosphodiesterase 3 inhibitor olprinone attenuates meconium-induced oxidative lung injury. Pulm Pharmacol Ther 2012;25:216-22. [PubMed]
- Goraca A, Skibska B. Beneficial effect of alpha-lipoic acid on lipopolysaccharide-induced oxidative stress in bronchoalveolar lavage fluid. J Physiol Pharmacol 2008;59:379-86. [PubMed]
- Broeckaert F, Clippe A, Knoops B, et al. Clara cell secretory protein (CC16): features as a peripheral lung biomarker. Ann N Y Acad Sci 2000;923:68-77. [PubMed]
- Wutzler S, Backhaus L, Henrich D, et al. Clara cell protein 16: a biomarker for detecting secondary respiratory complications in patients with multiple injuries. J Trauma Acute Care Surg 2012;73:838-42. [PubMed]
- Simon B, Ebert J, Bokhari F, et al. Management of pulmonary contusion and flail chest: an Eastern Association for the Surgery of Trauma practice management guideline. J Trauma Acute Care Surg 2012;73:S351-61. [PubMed]
- Tsangaris I, Galiatsou E, Kostanti E, et al. The effect of exogenous surfactant in patients with lung contusions and acute lung injury. Intensive Care Med 2007;33:851-5. [PubMed]
- Svennevig JL, Pillgram-Larsen J, Fjeld NB, et al. Early use of corticosteroids in severe closed chest injuries: a 10-year experience. Injury 1987;18:309-12. [PubMed]
- Svennevig JL, Bugge-Asperheim B, Bjørgo S, et al. Methylprednisolone in the treatment of lung contusion following blunt chest trauma. Scand J Thorac Cardiovasc Surg 1980;14:301-5. [PubMed]
- Just-Viera JO, Gonzalez A, Santiago-Delpin E. Glycerol and methylprednisolone in lung contusion. Am Surg 1980;46:33-7. [PubMed]
- Crisafulli C, Mazzon E, Muià C, et al. Effects of combination of melatonin and dexamethasone on acute lung injury in a mice model of carrageenan-induced pleurisy. J Pineal Res 2006;41:228-37. [PubMed]
- Cayli SR, Kocak A, Yilmaz U, et al. Effect of combined treatment with melatonin and methylprednisolone on neurological recovery after experimental spinal cord injury. Eur Spine J 2004;13:724-32. [PubMed]