Re-understanding anti-influenza strategy: attach equal importance to antiviral and anti-inflammatory therapies
Introduction
Influenza is an acute, contagious infectious disease caused by influenza virus; the disease can be harmful to human health, even leading to death. Great progress has been made in the treatment of influenza, especially for patients with severe influenza infection. The most effective approaches to prevention and control measures against influenza disease are vaccination and antiviral therapy. However, owing to antigenic variation of influenza viruses and individual differences in host immunity, the influenza vaccine can be less than effective. Thus, timely and regular antiviral treatment is especially important for confirmed influenza-positive patients, which can greatly reduce the patient’s symptoms and complications and shorten the course of the disease (1,2).
In recent years, together with new understanding of the pathogenesis of influenza, it has been recognized that influenza viruses can directly affect the host and can also cause indirect effects via severe immunological disorders. Influenza virus infection can promote chemokine and cytokine secretion, which help to clear influenza viruses from the host. However, in some cases, excessive or uncontrolled release of inflammatory cytokines occurs after influenza infection, resulting in a so-called “cytokine storm” that can lead to multiple organ damage and even death (3-6). Methods to alleviate the damage caused by a cytokine storm in response to influenza virus infection have become an important part of anti-influenza strategies.
We now have greater understanding of influenza viruses, including the direct harm caused by the virus itself as well as the cytokine storm induced by influenza viruses. These can act together and cause multiple organ dysfunction, such as acute respiratory distress syndrome (ARDS). In this review, we discuss reliable and effective antiviral and anti-inflammatory therapies, which must both be considered to guide the treatment of influenza patients.
Etiology, epidemiology, and impact of influenza viruses
Influenza viruses belong to the family Orthomyxoviridae and are single-stranded, negative-sense, segmented RNA viruses. According to nucleocapsid protein (NP) and matrix protein, influenza viruses are classified into influenza A, B, C, and D. Influenza A and B viruses contain eight different RNA segments, whereas influenza C viruses only have seven different RNA segments, lacking a fragment encoding the neuraminidase (NA) protein. The influenza virus genome is segmented, thus re-assorting among different virus strains readily occurs. In addition, influenza virus RNA often does not function correctly during replication; consequently, influenza has a higher mutation frequency than other viruses. Therefore, new influenza subtypes can easily emerge, leading to influenza epidemics. Influenza viruses have different species specificity. Influenza A virus can infect humans as well as pigs, horses, seals, whales, and ferrets, among other species. Influenza B virus has thus far been isolated in only humans, and influenza C virus has been isolated in only humans and pigs (7). Influenza D virus, recently reported in 2016, has not been implicated in human infection (8).
Influenza A and B viruses contain eight different negative sense (anti-mRNA sense) RNA segments that encode at least 11 viral proteins, including hemagglutinin (HA), NA, NP, matrix proteins (M1, M2), nonstructural proteins (NS1, NS2), and polymerase (PA, PB1, PB2, PB1-F2). Influenza C viruses only have seven different RNA segments, lacking a fragment encoding NA. The current study confirms that some of these proteins have an important role in the infection of the host by influenza virus. HA is responsible for virus attachment to host respiratory epithelial cells and facilitates virus entry into cells. NA can help the virus to pass through mucous membranes, which helps to promote the release and spread of newly synthesized virions from infected cells (9). The PB1, PB2, and PA subunits of influenza virus can form a heterotrimer that associates with NP-complexed viral RNA (vRNA) to form virion ribonucleoproteins (vRNPs) (10). M2 is a multifunctional protein whose transmembrane domain plays a critical role in ion channel activity and whose cytoplasmic domain is required for vRNP incorporation and virion morphogenesis (11). Detailed information about influenza virus replication and the function of each protein have been well summarized in other recent reviews (12). These proteins are also the key targets for anti-influenza therapies. Currently, influenza virus infection can be effectively treated with anti-influenza antiviral agents, which are directed against these influenza virus proteins. These agents either inhibit viral replication by blocking the ion channel at the stage of virus entry into cells or they inhibit the function of virus-coded RNA polymerases or prevent the release of progeny virions from infected cells, among other functions (13,14).
About 20–30% of children and 5–10% of adults in the general population are affected by influenza annually. Approximately 3–5 million people develop severe influenza infection, leading to about 1 million deaths per year (15). Four influenza A pandemics have occurred during the 20th century: the 1918 Spanish influenza pandemic (H1N1), the 1957 Asian influenza pandemic (H2N2), the 1968 Hong Kong influenza pandemic (H3N2), and the 1977 Russian influenza pandemic (H1N1). In the present century, the threat of influenza A virus pandemics remains. In 2009, a new H1N1 influenza virus pandemic swept through 74 countries in 2 months, causing nearly 20,000 deaths (16). Furthermore, research has been focusing more on avian influenza A viruses, which are transmitted among birds and can cause cross-species transmission to infect humans; these viruses include H5N1 (17), H7N9 (18,19), and H5N6 (20). Human infection with avian influenza virus can cause acute lung injury, leading to severe ARDS and multiple organ dysfunction syndrome (MODS); the disease severity and mortality rates are higher than those owing to severe acute respiratory syndrome (SARS) coronavirus. Data published by the China Centers for Disease Control (CDC) show that the mortality rate of highly pathogenic avian influenza A (H5N1) virus is >60%, and influenza A (H7N9) virus has a mortality rate of about 40%. Thus, influenza virus continues to be a major threat to human health and public safety, especially avian influenza.
Pathogenesis of influenza virus infections
Influenza can lead to different degrees of inflammation. Initial symptoms are usually mild, and patients typically develop fever, cough, expectoration, and other symptoms of upper respiratory tract infection. However, some patients quickly develop severe symptoms and pneumonia, ARDS, and other organ dysfunction (21). It is generally believed that the pathogenesis of influenza virus infection includes two main aspects: direct damage caused by virus replication and indirect damage caused by an influenza-induced cytokine storm.
Direct damage caused by influenza virus
The surface hemagglutinin protein (HA) of influenza virus is a glycoprotein that binds to sialic acid receptors on host cells in the respiratory tract; HA mediates viral adsorption and fusion. Influenza viruses then enter host cells via endocytosis, the viral genome is transcribed and replicated in the nucleus, and viral nucleoprotein synthesized in the cytoplasm enters the nucleus and combines with viral RNA to form a nucleocapsid that is exported to the cytoplasm. The nucleocapsid is tightly wrapped in a cell membrane modified by virus-specific membrane proteins, and viral particles are subsequently released through a budding process to infect other normal cells (22). Depending on the viral receptors present, seasonal influenza viruses will generally adhere to and multiply in epithelial cells of the upper respiratory tract. However, avian influenza A viruses, such as H7N9 and H5N1, can invade epithelial cells of the lower respiratory tract and alveolar type II pneumocytes where it can efficiently replicate, which may cause deteriorating lung function (23-25).
Adhesion, multiplication, and release of influenza virus in epithelial cells can damage the barrier function of the respiratory tract mucosa, which can cause further damage to the host. First, this process damages the integrity of the normal epithelial cell layer and enhances bacterial colonization, allowing strongly adhesive bacteria [such as Streptococcus pneumoniae (S. pneumoniae)] to colonize (26,27). Second, viral replication exposes the basement membrane, which is rich in fibers that connect proteins, causing respiratory pathogens (such as S. pneumoniae) to have high-affinity binding to proteins. Third, the NA protein of influenza virus can promote viral release from infected cells and help the virus spread in the respiratory tract while simultaneously exposing bacterial receptors in respiratory tissue, which can increase secondary bacterial infection by cleaving sialic acid receptors of epithelial cells (28-30). Finally, influenza virus-infected cells may have reduced expression of antimicrobial peptides, thereby affecting the natural defense function of the host epithelium (31).
Indirect damage caused by influenza-induced excessive inflammatory response
In addition to direct damage, influenza virus also causes indirect damage by inducing an excessive inflammatory response. Influenza virus is highly infectious in human respiratory cells, including endothelial cells, plasma cell-like dendritic cells, epithelial cells, and macrophages. Influenza virus infection causes these cells to release chemokines and cytokines, leading to aggregation of inflammatory cells such as neutrophils, lymphocytes, and monocytes in inflammatory regions (22). For example, airway epithelial cells, macrophages, and dendritic cells of the lung that are infected by influenza virus, viral RNA within an infected cell, and/or virus debris taken up by phagocytic cells lead to NLRP3 signaling activation, interleukin (IL)-1β production, and recruitment of neutrophils and monocytes to the infection site, where they further secrete inflammatory cytokines (32). Dysfunction of the chemokine and cytokine response is associated with severe avian influenza virus infection (17,33), which may contribute to disease severity (34). Additional evidence has showed that patients with H5N1 infection have higher serum levels of macrophage and neutrophil chemoattractant chemokines (CXCL10, CXCL2, IL-8) and pro- and anti-inflammatory cytokines [IL-6, IL-10, interferon (IFN)-γ], particularly those patients who died (17,35). Furthermore, a study on the relationship between viral load and cytokines in the second wave of H7N9 patients in Guangdong Province, China during 2013–2014 showed that there was a positive correlation between viral titers in different clinical specimens and cytokine levels in plasma and bronchoalveolar fluid. Specifically, upregulation of proinflammatory cytokines IP-10, MCP-1, MIG, MIP-1α/β, IL-1β, and IL-8 was found in plasma and bronchoalveolar fluid (19). Increased levels of IP-10, MIG, MIP-1β, MCP-1, IL-6, IL-8, and IFN-α were detected in sera from acute patients with H7N9 infection, which were significantly higher than those in healthy study participants. Some low pathogenic avian influenza viruses, such as avian influenza A (H9N2) virus, can also promote the expression of proinflammatory cytokines and chemokines in human airway epithelial cells. H9N2 virus can stimulate production of proinflammatory cytokines (IL-1β, IL-6) and chemokines (IL-8) (36). Similar findings have been observed in patients with severe influenza A (H1N1)pdm09 and seasonal influenza (37).
As described above, proinflammatory cytokine levels increase after influenza virus infection, which can help clear the virus from host cells. However, this increase may lead to an imbalance in the host regulatory network, leading to a cytokine storm, which can cause more serious conditions such as ARDS and MODS (3-5). There is evidence that cytokine dysregulation contributes to the pathogenesis of H5N1 and H7N9 viruses, which cause severe respiratory disease (17,25). Additionally, cytokine levels have been positively correlated with prolonged hospitalization and C-reactive protein levels and negatively correlated with oxygenation index (38). Another study suggested IP-10 and IL-6 levels as biomarkers for severe cases of H7N9 disease (39).
Treatment strategy for influenza
As described above, damage caused by influenza virus infection can be classified as direct, such as that caused by virus replication, and indirect, such as that caused by a cytokine storm. Therefore, the clinical treatment of influenza should be focused on both antiviral and anti-inflammatory therapies.
Importance of antiviral therapy
More and more evidence has confirmed that antiviral inhibitors are important for influenza treatment. The viral load in influenza patients correlates with disease severity and prolonged viral shedding (40), particularly in infants and young children, who are at high risk of influenza-related hospitalization and adverse disease outcomes. Therefore, antiviral agents are necessary in influenza treatment. One major class of antiviral drug is neuraminidase inhibitors (NAI), which can inhibit NA activities such as glycoside hydrolase activity, which cleaves glycosidic linkages of neuraminic acids to reduce viral spread and second-round infection. Such drugs include older NAIs, such as oseltamivir and zanamivir, and newer NAIs like laninamivir and peramivir. The other major class of antiviral drug is M2 ion channel protein inhibitors (M2 inhibitors), which target the influenza virus M2 protein and can inhibit the replication of influenza virus by interfering with the M2 protein ion channel activity. M2 inhibitors include amantadine and rimantadine. Other antivirals include hemagglutinin inhibitors, such as EB peptide and arbidol; viral RNA polymerase inhibitors, such as ribavirin; and traditional Chinese medicine, which contains anti-influenza activity.
NAI and M2 inhibitors have been approved by the United States Food and Drug Administration for the clinical treatment of influenza. However, because of high resistance (41-43), M2 inhibitors have been withdrawn from the market; currently, NAIs are mainly used. NAIs, such as oseltamivir, remain the mainstay of influenza therapy as they have good tolerance, low toxicity, lower resistance rate, and can effectively inhibit viral replication and transmission of various influenza virus strains (44,45). Thus, NAIs are currently included in national and international guidelines for the use of influenza drugs to guide clinical treatment (45,46).
An accumulation of data has shown that anti-influenza drugs, such as oseltamivir, can significantly reduce the median duration of symptoms in patients with influenza infection and viral shedding (47). Clinical analysis of antiviral treatment has revealed that the median length of hospitalization of participants who received antiviral therapy was significantly shorter than that of participants who did not (2,48,49). Findings of a double-blind, randomized, controlled trial showed that median duration of symptoms and virus isolation were lower in patients who received oseltamivir treatment within 48 hours of symptom onset compared with those given a placebo (50,51); this finding suggests that early antiviral treatment is efficacious. However, further clinical trials and basic research studies suggest that delayed treatment with NAIs (>48 hours since illness onset) remains effective against influenza infection (52,53).
Although antiviral monotherapy has been shown to be effective, there are some limitations to its use. Primarily, viral resistance has been shown to reduce the activity of antivirals such as amantadine and rimantadine, which have been rendered largely ineffective owing to the high prevalence of resistant viruses in nature (54). Initially, NAI and M2 inhibitors were highly successful in inhibiting and preventing influenza infection, and nearly no resistance to influenza virus was detected (55,56). However, in 2003 and 2004, the frequency of adamantane-resistant A (H3N2) viruses in China increased substantially to 57.5% and 73.8%, respectively (57). Our previous study suggested that clinical isolates of H1N1 and H3N2 developed amantadine resistance at 93.1% and 100%, respectively, in Guangzhou, China, 2009 (43). Dong et al. found that 45.2% of influenza A (H1-H17) viruses circulating globally in 2013 were resistant to adamantanes (58). Up until 2007, influenza viruses resistant to the NA inhibitor oseltamivir had been isolated at a low level (13); however, since the beginning of 2007, the resistance of influenza A (H1N1) viruses to oseltamivir has increased significantly, by 7.1% (59). Moreover, resistance to zanamivir has been reported among immunodeficient patients (60). Recently, according to antiviral susceptibility monitoring of the World Health Organization Global Influenza Surveillance and Response System, virtually all influenza A viruses circulating in humans have been deemed resistant to one category of antiviral drugs, namely, M2 inhibitors (amantadine and rimantadine). However, as of October 2017, the frequency of resistance to the NA inhibitor oseltamivir remains low (1–2%), (http://www.who.int/mediacentre/factsheets/fs194/en/).
Thus, clinicians should consider combination therapy using two or three antiviral drugs to produce synergistic effects, increase anti-influenza virus activity, reduce the emergence of drug-resistant virus strains, and lower influenza mortality rates (61,62). However, combination therapy can reduce the use of individual antivirals and may have dose-related drug toxicity and adverse effects (13). One such example is oseltamivir-ribavirin combination therapy, which significantly inhibits influenza A virus replication in experimental mouse models, controls the spread of influenza viruses in the respiratory tract, and improves parameters of lung function, particularly mean breath volume (63,64). In addition, oseltamivir-zanamivir combination therapy has been found to be less effective than oseltamivir or zanamivir monotherapy for seasonal influenza A (H3N2) virus and H1N1 virus (65). Some combination therapies may exhibit antagonistic effects. One group noted that oseltamivir and peramivir both act on N1 NA enzymes; however, peramivir is more likely to bind to N1 NA enzymes than oseltamivir, which may lead to antagonistic interactions (66). Moreover, a retrospective study of adults infected with H7N9 virus showed that oseltamivir-peramivir combination therapy was not superior to oseltamivir monotherapy (67). Thus, antiviral drugs included in combination therapies should have different antiviral mechanisms (68). If the antivirals are in the same class, then an antagonistic effect between the two drugs is likely to occur because they compete for the same binding site, which ultimately affects the antiviral activity of the drug.
Owing to the rapid and extensive emergence of drug resistance among the available drugs, as well as the increasing need for combination therapy, new antiviral strategies with novel mechanisms of action and reduced drug resistance potential are urgently required. Recent years have seen the development of inhibitors that block virus-cell interactions occurring at different stages of influenza virus replication, such as attachment, entry, viral genome transcription and replication, nuclear export of viral products, and viral particles release. The new antiviral strategies target HA (69,70), RNA polymerase (71-73), NP (74), and NS1 (75); however, to date, no anti-influenza drugs targeting NS1 are under clinical development. These have been well summarized in other recent reviews (12,76). There are new developments in NA inhibitors, such as laninamivir, which holds great promise for its long-acting inhibitory activity (77). The characteristics of these anti-influenza virus drugs have been summarized in Table 1.
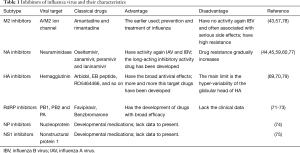
Full table
Here, we only review anti-influenza drugs according to the important functional proteins of influenza virus. However, other anti-influenza therapies should also be adopted, including those that regulate cell metabolism, host signaling pathways, and host antiviral response. Detailed information has been provided in other recent reviews (12,80).
Importance of anti-inflammatory therapy
In some cases, the cytokine storm induced by influenza virus infection is an important cause of ARDS, MODS and even patient death (6). Therefore, in addition to conventional antivirus therapies, the use of inflammation inhibitors in patients, especially those infected by highly pathogenic avian influenza viruses, is another possible therapeutic approach. Currently, corticosteroids are the most widely used and effective adjuvant therapy. Glucocorticoids can reduce alveolar effusion, capillary permeability, and the inflammatory response of the lung parenchyma and interstitium, to shorten the course of severe viral pneumonia. The use of glucocorticoids in the treatment of severe viral pneumonia has come from the experience of SARS treatment in Guangzhou, China. SARS coronavirus induces a IFN-γ-related cytokine storm in patients, which might be involved in the immunopathological damage observed in patients with SARS (81). A retrospective study of Guangzhou SARS cases showed that corticosteroid use reduced mortality and shortened hospitalization (82). In an experimental mouse model of H1N1 infection, corticosteroid treatment ameliorated acute lung injury induced by 2009 H1N1 virus (83). However, other reports have suggested that corticosteroid therapy does not significantly improve the survival of patients with influenza A virus infection (84) and may even be associated with higher mortality in patients with severe influenza H1N1 infection (85). Among H1N1-positive patients with ARDS in particular, the early use of corticosteroids may be harmful (86). Therefore, there is no clear consensus on corticosteroid use in the treatment of severe viral pneumonia or on the timing, dosage, and course of treatment.
Passive immunotherapy may be a good option for the treatment of influenza A (H5N1 or H1N1) infection. Treatment with convalescent plasma in patients with H1N1 infection can effectively reduce respiratory tract viral load, serum cytokine response, and mortality (87,88). Furthermore, some groups have applied monoclonal antibodies to combat the abnormal secretion of cytokines under pathological conditions or have used specific recombinant protein to regulate the cytokine storm, which can also alleviate immune damage to some extent. For example, some studies have found that IP-10 and IL-17 were significantly elevated in the serum of patients with H1N1-induced ARDS. In an experimental mouse model of acute lung injury caused by influenza A (H1N1) virus infection, anti-IL-17 and anti-IP-10 monoclonal antibodies significantly reduced the expression of related cytokines and reduced lung injury (89,90).
Anti-inflammatory therapy has become an important component of anti-influenza strategies, and equal importance should be placed on antiviral and anti-inflammatory therapies to effectively treat severe influenza virus infection. However, the optimal anti-inflammatory drug, duration of treatment, and timing of treatment remain unclear and require further investigation.
Influenza treatment with traditional Chinese medicine
There are some disadvantages to using antiviral and anti-inflammatory therapies; mainly, most drugs do not contain both antiviral and anti-inflammatory activities. It has been postulated that novel antiviral agents could control influenza, thus attenuating excessive proinflammatory responses and limiting symptoms and tissue damage associated with infection. An increasing number of antiviral drugs influence host immune responses (91), with the most successful drugs possessing both antiviral and anti-inflammatory functions (92). Based on this concept, traditional Chinese herbal medicine (TCM) has an innate advantage in that it can target the virus and the host simultaneously, offering more effective inhibition of host inflammatory responses induced by influenza virus infection. Thus, the development of novel therapeutic agents from TCM is a promising approach for anti-influenza strategies.
Various TCMs have been shown to have anti-influenza effects, such as the herb Radix isatidis (R. isatidis), honeysuckle, forsythia, and compound formulas Lianhua-Qingwen and MaxinShigan Tang. Lianhua-Qingwen capsules are an important TCM that has been confirmed to have anti-influenza A activity in a double-blind, randomized, positive-controlled clinical trial (93,94). Furthermore, we have found that Lianhua-Qingwen capsules have strong efficacy in inhibiting virus-induced excessive inflammation immune responses (95), which has also been observed with R. isatidis (96). Thus, in addition to directly inhibiting viral replication, TCM regulates influenza virus-induced excessive inflammatory immune responses; the latter effect is has a beneficial role in fighting influenza infection.
R. isatidis, also known as Ban-Lan-Gen in Chinese, has traditionally been used for the treatment of influenza, viral pneumonia, mumps, pharyngitis, and hepatitis (97). Clinical studies have also demonstrated high efficacy of R. isatidis in acute pharyngitis (50% caused by a virus) and influenza virus-induced acute upper respiratory infections and pneumonia, including cough, nasal congestion, runny nose, sneezing, and other inflammatory catarrhal symptoms (98,99). Another study showed that R. isatidis also plays a significant role in the treatment of viral myocarditis and other viral-mediated inflammatory diseases (100). These clinical observations suggest that R. isatidis has therapeutic effects for mild to moderate influenza-like symptoms and virus-induced inflammatory catarrhal symptoms.
Pharmacological studies have also demonstrated that R. isatidis components serve two main functions: antiviral and anti-inflammatory. Various types of compounds have been isolated from R. isatidis including polysaccharides, indirubin, clemastanin B, alkaloids, lignans, and flavonoids. Our research and previous studies have shown that R. isatidis polysaccharides inhibit in vitro replication of influenza virus (101); the underlying mechanism may include direct competitive binding to the HA of the virus, thereby inhibiting viral invasion and replication (102). We further elucidated that polysaccharides have a strong anti-inflammatory effect on influenza virus via suppression of host toll-like receptor 3 (TLR3) signaling in vitro (96). Moreover, Kido et al. reported that one of the antiviral components of R. isatidis, namely, isatis root polysaccharide, induces the production of protective IgG antibodies, which may serve as an antiviral vaccine adjuvant (103).
It is unclear whether indirubin, an indole alkaloid, has a direct effect on influenza virus in vitro (104). However, it is clear that indirubin and its derivatives can significantly inhibit influenza virus (H5N1)-induced overproduction of inflammatory cytokines, such as IP-10 and MIG, in airway epithelial cells and macrophages (105). Moreover, indirubin has potent anti-influenza viral activity via inhibition of RANTES (also known as CCL5) expression (106). Concerning lignans, our preliminary study demonstrated that clemastanin B, a lignan, can slightly inhibit replication of influenza virus in vitro by inhibiting the expression and nuclear export of early influenza virus NP (97). In recent studies, we demonstrated that lariciresinol-4-O-β-d-glucopyrano-side, another lignan, exhibited strong anti-influenza virus A activity and decreased proinflammatory cytokine expression via inhibition of the NF-κB pathway in influenza A virus-infected alveolar epithelial (A549) cells (107). Thus, as described above, R. isatidis contains numerous chemical substances with a broad spectrum of anti-viral and anti-inflammatory activities that may serve as potential therapeutic targets.
In-depth study of the clinical and basic pharmacology of R. isatidis has revealed its broad anti-influenza effects: it is not only a direct antiviral but, more significantly, R. isatidis also inhibits virus-induced inflammation by regulating excessive host immune responses. These pharmacodynamic characteristics are not restricted to R. isatidis; other anti-influenza medicines also exhibit these activities. Therefore, a deeper understanding of new anti-influenza strategies using combined antiviral and anti-inflammatory therapies is required. Particular emphasis should be placed on the pharmacodynamic characteristics of TCM in inhibiting influenza virus-induced host inflammatory and immune responses.
In addition, based on the superior anti-inflammatory efficacy of TCM, some researchers have investigated the treatment of influenza with oseltamivir plus TCMs. Using this approach, the median duration of fever after treatment was significantly shorter in patients who received Mao-to (a Japanese traditional herbal medicine) plus oseltamivir compared with those who received oseltamivir only. Clinical trials have indicated that combination therapy that includes Mao-to is effective in controlling fever owing to type A influenza infection in children (108). Another clinical trial also found that maxingshigan-yinqiaosan (a TCM) in combination with oseltamivir obviously reduced the time to fever resolution in patients with H1N1 influenza virus infection, as compared with treatment using oseltamivir alone (94). These clinical trials indicate that TCMs are a good support in oseltamivir treatment that can reduce the duration time of fever. There is also evidence showing that TCM (Lianhua-Qingwen capsules) significantly reduces the severity of illness and duration of symptoms including cough, sore throat, and fatigue (93). However, data are lacking regarding whether treatment with TCMs plus oseltamivir is effective for inhibiting viral shedding or reducing the outbreak rate of viral resistance. These topics warrant further research in the future. Nevertheless, drug interactions must be seriously considered when using TCMs plus oseltamivir.
Conclusions
Influenza viruses, especially avian influenza viruses, still cause significant human disease and can lead to direct or indirect harm via viral replication or hyper-inflammatory immune responses, respectively. Antiviral therapy is particularly beneficial for the treatment of mild influenza or influenza-like pneumonia in adults and children. Although early antiviral use is critical for controlling influenza, delayed use of some NAIs (oseltamivir or peramivir) may also be effective. However, anti-inflammatory treatment of influenza must not be omitted, especially for avian influenza. Therefore, we stress the equal importance of antiviral and anti-inflammatory therapies when developing anti-influenza strategies. Additionally, the use of TCMs for influenza treatment has gained recent attention as TCMs have both antiviral and anti-inflammatory activities and may represent potent new anti-influenza agents.
Acknowledgements
Funding: This work was supported by the National Natural Science Foundation of China (grant numbers 81703788, U1201227, 81761128014 and 81490534); the Ministry of Science and Technology of China (grant number 2015DFM30010); the Administration of Traditional Chinese Medicine of Guangdong Province, China (grant number 20174005); the Science Research Project of the Guangdong Province (grant number 2016A050503047); the Municipal Science and Technology Bureau Foundation of Guangzhou (grant number 2014Y2-00031); and the Academician Zhong Nanshan workstation (grant number 2015IC001).
Footnote
Conflicts of Interest: The authors have no conflicts of interest to declare.
References
- Yang P, Deng Y, Pang X, et al. Severe, critical and fatal cases of 2009 H1N1 influenza in China. J Infect 2010;61:277-83. [Crossref] [PubMed]
- Qu JX, Gu L, Pu ZH, et al. Viral etiology of community-acquired pneumonia among adolescents and adults with mild or moderate severity and its relation to age and severity. BMC Infect Dis 2015;15:89. [Crossref] [PubMed]
- Peiris JS, Hui KP, Yen HL. Host response to influenza virus: protection versus immunopathology. Curr Opin Immunol 2010;22:475-81. [Crossref] [PubMed]
- Peiris JS, Cheung CY, Leung CY, et al. Innate immune responses to influenza A H5N1: friend or foe? Trends Immunol 2009;30:574-84. [Crossref] [PubMed]
- Oakley OR, Garvy BA, Humphreys S, et al. Increased weight loss with reduced viral replication in interleukin-10 knock-out mice infected with murine cytomegalovirus. Clin Exp Immunol 2008;151:155-64. [Crossref] [PubMed]
- Zheng BJ, Chan KW, Lin YP, et al. Delayed antiviral plus immunomodulator treatment still reduces mortality in mice infected by high inoculum of influenza A/H5N1 virus. Proc Natl Acad Sci U S A 2008;105:8091-6. [Crossref] [PubMed]
- Zhong NS, Li YM, Yang ZF, et al. Chinese guidelines for diagnosis and treatment of influenza (2011). J Thorac Dis 2011;3:274-89. [PubMed]
- Song H, Qi J, Khedri Z, et al. Correction: An Open Receptor-Binding Cavity of Hemagglutinin-Esterase-Fusion Glycoprotein from Newly-Identified Influenza D Virus: Basis for Its Broad Cell Tropism. PLoS Pathog 2016;12. [Crossref] [PubMed]
- Prober CG. Antiviral therapy for influenza virus infections. Semin Pediatr Infect Dis 2002;13:31-9. [Crossref] [PubMed]
- Perales B, Sanz-Ezquerro JJ, Gastaminza P, et al. The replication activity of influenza virus polymerase is linked to the capacity of the PA subunit to induce proteolysis. J Virol 2000;74:1307-12. [Crossref] [PubMed]
- Iwatsuki-Horimoto K, Horimoto T, Noda T, et al. The cytoplasmic tail of the influenza A virus M2 protein plays a role in viral assembly. J Virol 2006;80:5233-40. [Crossref] [PubMed]
- Loregian A, Mercorelli B, Nannetti G, et al. Antiviral strategies against influenza virus: towards new therapeutic approaches. Cell Mol Life Sci 2014;71:3659-83. [Crossref] [PubMed]
- Govorkova EA, Webster RG. Combination chemotherapy for influenza. Viruses 2010;2:1510-29. [Crossref] [PubMed]
- Barik S. New treatments for influenza. BMC Med 2012;10:104. [Crossref] [PubMed]
- Vaccines against influenza WHO position paper - November 2012. Wkly Epidemiol Rec 2012;87:461-76. [PubMed]
- Michaelis M, Doerr HW, Cinatl J Jr. An influenza A H1N1 virus revival - pandemic H1N1/09 virus. Infection 2009;37:381-9. [Crossref] [PubMed]
- de Jong MD, Simmons CP, Thanh TT, et al. Fatal outcome of human influenza A (H5N1) is associated with high viral load and hypercytokinemia. Nat Med 2006;12:1203-7. [Crossref] [PubMed]
- Gao R, Cao B, Hu Y, et al. Human infection with a novel avian-origin influenza A (H7N9) virus. N Engl J Med 2013;368:1888-97. [Crossref] [PubMed]
- Yang ZF, Mok CK, Liu XQ, et al. Clinical, virological and immunological features from patients infected with re-emergent avian-origin human H7N9 influenza disease of varying severity in Guangdong province. PloS one 2015;10. [Crossref] [PubMed]
- Yang ZF, Mok CK, Peiris JS, et al. Human Infection with a Novel Avian Influenza A(H5N6) Virus. N Engl J Med 2015;373:487-9. [Crossref] [PubMed]
- Feng Y, Guan W, Yuan B, et al. Emergence of triple-subtype reassortants of fatal human H5N6 avian influenza virus in Yunnan, China. J Infect 2016;72:753-6. [Crossref] [PubMed]
- Jingxian Chen RZ, Tao Peng, Dapeng Xiang, Mingli Wang. Clinical Virology. China Science Publishing & Media Ltd 2012:950-83.
- Pastva AM, Wright JR, Williams KL. Immunomodulatory roles of surfactant proteins A and D: implications in lung disease. Proc Am Thorac Soc 2007;4:252-7. [Crossref] [PubMed]
- Gu J, Xie Z, Gao Z, et al. H5N1 infection of the respiratory tract and beyond: a molecular pathology study. Lancet 2007;370:1137-45. [Crossref] [PubMed]
- Zhou J, Wang D, Gao R, et al. Biological features of novel avian influenza A (H7N9) virus. Nature 2013;499:500-3. [Crossref] [PubMed]
- Bogaert D, De Groot R, Hermans PW. Streptococcus pneumoniae colonisation: the key to pneumococcal disease. Lancet Infect Dis 2004;4:144-54. [Crossref] [PubMed]
- Håkansson A, Kidd A, Wadell G, et al. Adenovirus infection enhances in vitro adherence of Streptococcus pneumoniae. Infect Immun 1994;62:2707-14. [PubMed]
- Peltola VT, Murti KG, McCullers JA. Influenza virus neuraminidase contributes to secondary bacterial pneumonia. J Infect Dis 2005;192:249-57. [Crossref] [PubMed]
- McCullers JA, Bartmess KC. Role of neuraminidase in lethal synergism between influenza virus and Streptococcus pneumoniae. J Infect Dis 2003;187:1000-9. [Crossref] [PubMed]
- Hedlund M, Aschenbrenner LM, Jensen K, et al. Sialidase-based anti-influenza virus therapy protects against secondary pneumococcal infection. J Infect Dis 2010;201:1007-15. [Crossref] [PubMed]
- Bosch AA, Biesbroek G, Trzcinski K, et al. Viral and bacterial interactions in the upper respiratory tract. PLoS Pathog 2013;9. [Crossref] [PubMed]
- Owen DM, Gale M Jr. Fighting the flu with inflammasome signaling. Immunity 2009;30:476-8. [Crossref] [PubMed]
- Abdel-Ghafar AN, Chotpitayasunondh T, Gao Z, et al. Update on avian influenza A (H5N1) virus infection in humans. N Engl J Med 2008;358:261-73. [Crossref] [PubMed]
- Tisoncik JR, Korth MJ, Simmons CP, et al. Into the eye of the cytokine storm. Microbiol Mol Biol Rev 2012;76:16-32. [Crossref] [PubMed]
- Peiris JS, Yu WC, Leung CW, et al. Re-emergence of fatal human influenza A subtype H5N1 disease. Lancet 2004;363:617-9. [Crossref] [PubMed]
- Farzin H, Toroghi R, Haghparast A. Up-Regulation of Pro-Inflammatory Cytokines and Chemokine Production in Avian Influenza H9N2 Virus-Infected Human Lung Epithelial Cell Line (A549). Immunol Invest 2016;45:116-29. [Crossref] [PubMed]
- Lee N, Wong CK, Chan PK, et al. Cytokine response patterns in severe pandemic 2009 H1N1 and seasonal influenza among hospitalized adults. PLoS One 2011;6. [Crossref] [PubMed]
- Hagau N, Slavcovici A, Gonganau DN, et al. Clinical aspects and cytokine response in severe H1N1 influenza A virus infection. Crit Care 2010;14:R203. [Crossref] [PubMed]
- Chi Y, Zhu Y, Wen T, et al. Cytokine and chemokine levels in patients infected with the novel avian influenza A (H7N9) virus in China. J Infect Dis 2013;208:1962-7. [Crossref] [PubMed]
- Giannella M, Alonso M, Garcia de Viedma D, et al. Prolonged viral shedding in pandemic influenza A(H1N1): clinical significance and viral load analysis in hospitalized patients. Clin Microbiol Infect 2011;17:1160-5. [Crossref] [PubMed]
- Deyde VM, Sheu TG, Trujillo AA, et al. Detection of molecular markers of drug resistance in 2009 pandemic influenza A (H1N1) viruses by pyrosequencing. Antimicrob Agents Chemother 2010;54:1102-10. [Crossref] [PubMed]
- Centers for Disease C. Prevention. Update: influenza activity - United States, August 30-October 31, 2009. MMWR Morb Mortal Wkly Rep 2009;58:1236-41. [PubMed]
- Yang ZF, Guan WD, Xu GR, et al. Adamantane resistance among seasonal influenza A viruses between January to October in Guangzhou, 2009. Zhonghua Jie He He Hu Xi Za Zhi 2011;34:95-9. [PubMed]
- Jefferson T, Demicheli V, Rivetti D, et al. Antivirals for influenza in healthy adults: systematic review. Lancet 2006;367:303-13. [Crossref] [PubMed]
- Committee On Infectious Diseases. American Academy Pediatrics. Recommendations for prevention and control of influenza in children, 2014-2015. Pediatrics 2014;134:e1503-19. [Crossref] [PubMed]
- Harper SA, Bradley JS, Englund JA, et al. Seasonal influenza in adults and children--diagnosis, treatment, chemoprophylaxis, and institutional outbreak management: clinical practice guidelines of the Infectious Diseases Society of America. Clin Infect Dis 2009;48:1003-32. [Crossref] [PubMed]
- Nicholson KG, Aoki FY, Osterhaus AD, et al. Efficacy and safety of oseltamivir in treatment of acute influenza: a randomised controlled trial. Neuraminidase Inhibitor Flu Treatment Investigator Group. Lancet 2000;355:1845-50. [Crossref] [PubMed]
- Lee N, Chan PK, Hui DS, et al. Viral loads and duration of viral shedding in adult patients hospitalized with influenza. J Infect Dis 2009;200:492-500. [Crossref] [PubMed]
- Rath B, von Kleist M, Tief F, et al. Virus load kinetics and resistance development during oseltamivir treatment in infants and children infected with Influenza A(H1N1) 2009 and Influenza B viruses. Pediatr Infect Dis J 2012;31:899-905. [Crossref] [PubMed]
- Kumar D, Michaels MG, Morris MI, et al. Outcomes from pandemic influenza A H1N1 infection in recipients of solid-organ transplants: a multicentre cohort study. Lancet Infect Dis 2010;10:521-6. [Crossref] [PubMed]
- Cao B, Li XW, Mao Y, et al. Clinical features of the initial cases of 2009 pandemic influenza A (H1N1) virus infection in China. N Engl J Med 2009;361:2507-17. [Crossref] [PubMed]
- Fry AM, Goswami D, Nahar K, et al. Efficacy of oseltamivir treatment started within 5 days of symptom onset to reduce influenza illness duration and virus shedding in an urban setting in Bangladesh: a randomised placebo-controlled trial. Lancet Infect Dis 2014;14:109-18. [Crossref] [PubMed]
- Li Z, Li R, Li J, et al. Efficacy of delayed treatment of China-made Peramivir with repeated intravenous injections in a mouse influenza model: from clinical experience to basal experiment. BMC Infect Dis 2016;16:325. [Crossref] [PubMed]
- Deyde VM, Xu X, Bright RA, et al. Surveillance of resistance to adamantanes among influenza A(H3N2) and A(H1N1) viruses isolated worldwide. J Infect Dis 2007;196:249-57. [Crossref] [PubMed]
- Dolin R, Reichman RC, Madore HP, et al. A controlled trial of amantadine and rimantadine in the prophylaxis of influenza A infection. N Engl J Med 1982;307:580-4. [Crossref] [PubMed]
- Hurt AC, Barr IG, Hartel G, et al. Susceptibility of human influenza viruses from Australasia and South East Asia to the neuraminidase inhibitors zanamivir and oseltamivir. Antiviral Res 2004;62:37-45. [Crossref] [PubMed]
- Bright RA, Medina MJ, Xu X, et al. Incidence of adamantane resistance among influenza A (H3N2) viruses isolated worldwide from 1994 to 2005: a cause for concern. Lancet 2005;366:1175-81. [Crossref] [PubMed]
- Dong G, Peng C, Luo J, et al. Adamantane-resistant influenza a viruses in the world (1902-2013): frequency and distribution of M2 gene mutations. PLoS One 2015;10. [Crossref] [PubMed]
- Sheu TG, Deyde VM, Okomo-Adhiambo M, et al. Surveillance for neuraminidase inhibitor resistance among human influenza A and B viruses circulating worldwide from 2004 to 2008. Antimicrob Agents Chemother 2008;52:3284-92. [Crossref] [PubMed]
- Lackenby A, Thompson CI, Democratis J. The potential impact of neuraminidase inhibitor resistant influenza. Curr Opin Infect Dis 2008;21:626-38. [Crossref] [PubMed]
- Ilyushina NA, Hoffmann E, Salomon R, et al. Amantadine-oseltamivir combination therapy for H5N1 influenza virus infection in mice. Antivir Ther 2007;12:363-70. [PubMed]
- Smee DF, Hurst BL, Wong MH, et al. Effects of double combinations of amantadine, oseltamivir, and ribavirin on influenza A (H5N1) virus infections in cell culture and in mice. Antimicrob Agents Chemother 2009;53:2120-8. [Crossref] [PubMed]
- Ilyushina NA, Hay A, Yilmaz N, et al. Oseltamivir-ribavirin combination therapy for highly pathogenic H5N1 influenza virus infection in mice. Antimicrob Agents Chemother 2008;52:3889-97. [Crossref] [PubMed]
- Julander JG, Hagloch J, Latimer S, et al. Use of plethysmography in assessing the efficacy of antivirals in a mouse model of pandemic influenza A virus. Antiviral Res 2011;92:228-36. [Crossref] [PubMed]
- Escuret V, Cornu C, Boutitie F, et al. Oseltamivir-zanamivir bitherapy compared to oseltamivir monotherapy in the treatment of pandemic 2009 influenza A(H1N1) virus infections. Antiviral Res 2012;96:130-7. [Crossref] [PubMed]
- Bantia S, Arnold CS, Parker CD, et al. Anti-influenza virus activity of peramivir in mice with single intramuscular injection. Antiviral Res 2006;69:39-45. [Crossref] [PubMed]
- Zhang Y, Gao H, Liang W, et al. Efficacy of oseltamivir-peramivir combination therapy compared to oseltamivir monotherapy for Influenza A (H7N9) infection: a retrospective study. BMC Infect Dis 2016;16:76. [Crossref] [PubMed]
- Smee DF, Hurst BL, Wong MH, et al. Combinations of oseltamivir and peramivir for the treatment of influenza A (H1N1) virus infections in cell culture and in mice. Antiviral Res 2010;88:38-44. [Crossref] [PubMed]
- Zhu L, Li Y, Li S, et al. Inhibition of influenza A virus (H1N1) fusion by benzenesulfonamide derivatives targeting viral hemagglutinin. PLoS One 2011;6. [Crossref] [PubMed]
- Nicol MQ, Ligertwood Y, Bacon MN, et al. A novel family of peptides with potent activity against influenza A viruses. J Gen Virol 2012;93:980-6. [Crossref] [PubMed]
- Furuta Y, Gowen BB, Takahashi K, et al. Favipiravir (T-705), a novel viral RNA polymerase inhibitor. Antiviral Res 2013;100:446-54. [Crossref] [PubMed]
- Perez DR, Donis RO. Functional analysis of PA binding by influenza a virus PB1: effects on polymerase activity and viral infectivity. J Virol 2001;75:8127-36. [Crossref] [PubMed]
- Loregian A, Palu G. Disruption of protein-protein interactions: towards new targets for chemotherapy. J Cell Physiol 2005;204:750-62. [Crossref] [PubMed]
- Lejal N, Tarus B, Bouguyon E, et al. Structure-based discovery of the novel antiviral properties of naproxen against the nucleoprotein of influenza A virus. Antimicrob Agents Chemother 2013;57:2231-42. [Crossref] [PubMed]
- Basu D, Walkiewicz MP, Frieman M, et al. Novel influenza virus NS1 antagonists block replication and restore innate immune function. J Virol 2009;83:1881-91. [Crossref] [PubMed]
- Amarelle L, Lecuona E, Sznajder JI. Anti-Influenza Treatment: Drugs Currently Used and Under Development. Arch Bronconeumol 2017;53:19-26. [PubMed]
- Koyama K, Takahashi M, Oitate M, et al. CS-8958, a prodrug of the novel neuraminidase inhibitor R-125489, demonstrates a favorable long-retention profile in the mouse respiratory tract. Antimicrob Agents Chemother 2009;53:4845-51. [Crossref] [PubMed]
- Coulombe F, Divangahi M. Targeting eicosanoid pathways in the development of novel anti-influenza drugs. Expert Rev Anti Infect Ther 2014;12:1337-43. [Crossref] [PubMed]
- Huang KJ, Su IJ, Theron M, et al. An interferon-gamma-related cytokine storm in SARS patients. J Med Virol 2005;75:185-94. [Crossref] [PubMed]
- Chen RC, Tang XP, Tan SY, et al. Treatment of severe acute respiratory syndrome with glucosteroids: the Guangzhou experience. Chest 2006;129:1441-52. [Crossref] [PubMed]
- Li C, Yang P, Zhang Y, et al. Corticosteroid treatment ameliorates acute lung injury induced by 2009 swine origin influenza A (H1N1) virus in mice. PLoS One 2012;7. [Crossref] [PubMed]
- Diaz E, Martin-Loeches I, Canadell L, et al. Corticosteroid therapy in patients with primary viral pneumonia due to pandemic (H1N1) 2009 influenza. J Infect 2012;64:311-8. [Crossref] [PubMed]
- Kim SH, Hong SB, Yun SC, et al. Corticosteroid treatment in critically ill patients with pandemic influenza A/H1N1 2009 infection: analytic strategy using propensity scores. Am J Respir Crit Care Med 2011;183:1207-14. [Crossref] [PubMed]
- Brun-Buisson C, Richard JC, Mercat A, et al. Early corticosteroids in severe influenza A/H1N1 pneumonia and acute respiratory distress syndrome. Am J Respir Crit Care Med 2011;183:1200-6. [Crossref] [PubMed]
- Zhou B, Zhong N, Guan Y. Treatment with convalescent plasma for influenza A (H5N1) infection. N Engl J Med 2007;357:1450-1. [Crossref] [PubMed]
- Hung IF, To KK, Lee CK, et al. Convalescent plasma treatment reduced mortality in patients with severe pandemic influenza A (H1N1) 2009 virus infection. Clin Infect Dis 2011;52:447-56. [Crossref] [PubMed]
- Wang W, Yang P, Zhong Y, et al. Monoclonal antibody against CXCL-10/IP-10 ameliorates influenza A (H1N1) virus induced acute lung injury. Cell Res 2013;23:577-80. [Crossref] [PubMed]
- Li C, Yang P, Sun Y, et al. IL-17 response mediates acute lung injury induced by the 2009 pandemic influenza A (H1N1) virus. Cell Res 2012;22:528-38. [Crossref] [PubMed]
- Baillie JK, Digard P. Influenza--time to target the host? N Engl J Med 2013;369:191-3. [Crossref] [PubMed]
- Ramos I, Fernandez-Sesma A. Modulating the Innate Immune Response to Influenza A Virus: Potential Therapeutic Use of Anti-Inflammatory Drugs. Front Immunol 2015;6:361. [Crossref] [PubMed]
- Duan ZP, Jia ZH, Zhang J, et al. Natural herbal medicine Lianhuaqingwen capsule anti-influenza A (H1N1) trial: a randomized, double blind, positive controlled clinical trial. Chin Med J (Engl) 2011;124:2925-33. [PubMed]
- Wang C, Cao B, Liu QQ, et al. Oseltamivir compared with the Chinese traditional therapy maxingshigan-yinqiaosan in the treatment of H1N1 influenza: a randomized trial. Ann Intern Med 2011;155:217-25. [Crossref] [PubMed]
- Ding Y, Zeng L, Li R, et al. The Chinese prescription lianhuaqingwen capsule exerts anti-influenza activity through the inhibition of viral propagation and impacts immune function. BMC Complement Altern Med 2017;17:130. [Crossref] [PubMed]
- Li Z, Li L, Zhou H, et al. Radix isatidis Polysaccharides Inhibit Influenza a Virus and Influenza A Virus-Induced Inflammation via Suppression of Host TLR3 Signaling In Vitro. Molecules 2017.22. [PubMed]
- Yang Z, Wang Y, Zheng Z, et al. Antiviral activity of Isatis indigotica root-derived clemastanin B against human and avian influenza A and B viruses in vitro. Int J Mol Med 2013;31:867-73. [Crossref] [PubMed]
- Tu B, Nie WM, Ding PP, et al. Efficacy of treatment of influenza A (H1N1) with oseltamivir phosphate and isatis root granules. Med J Chin PAPF 2013;24:6.
- Ganlin D. The cfinic observation of 100 cases with acute pharyngitis cured by composite Banlangen tablet. J Precl1n Med Coll Siiandong Med Univ 2002;16:93-4.
- Ye WY, Li X, Cheng JW. Screening of 11 Antiviral Effect Constituents from Radix Isatidi. JETCM 2011;20:1772-4.
- Yang ZF, Wang YT, Qin S, et al. The effects of a hot water soluble extract (S-03) isolated from Isatis indigotica root on influenza A and B viruses in vitro. Bing Du Xue Bao 2011;27:218-23. [PubMed]
- Yang Z, Wang Y, Zhong S, et al. In vitro inhibition of influenza virus infection by a crude extract from Isatis indigotica root resulting in the prevention of viral attachment. Mol Med Rep 2012;5:793-9. [PubMed]
- Kido H, Okumura Y, Yamada H, et al. Proteases essential for human influenza virus entry into cells and their inhibitors as potential therapeutic agents. Curr Pharm Des 2007;13:405-14. [Crossref] [PubMed]
- XU LH, Huang F, Cheng T, et al. Antivirus constituents of radix of isatis indigotica. Chin J Nat Med 2005;3:359-60.
- Mok CK, Kang SS, Chan RW, et al. Anti-inflammatory and antiviral effects of indirubin derivatives in influenza A (H5N1) virus infected primary human peripheral blood-derived macrophages and alveolar epithelial cells. Antiviral Res 2014;106:95-104. [Crossref] [PubMed]
- Mak NK, Leung CY, Wei XY, et al. Inhibition of RANTES expression by indirubin in influenza virus-infected human bronchial epithelial cells. Biochem Pharmacol 2004;67:167-74. [Crossref] [PubMed]
- Li J, Zhou B, Li C, et al. Lariciresinol-4-O-beta-D-glucopyranoside from the root of Isatis indigotica inhibits influenza A virus-induced pro-inflammatory response. J Ethnopharmacol 2015;174:379-86. [Crossref] [PubMed]
- Kubo T, Nishimura H. Antipyretic effect of Mao-to, a Japanese herbal medicine, for treatment of type A influenza infection in children. Phytomedicine 2007;14:96-101. [Crossref] [PubMed]
- Pinto LH, Lamb RA. The M2 proton channels of influenza A and B viruses. J Biol Chem 2006;281:8997-9000. [Crossref] [PubMed]
- Boriskin YS, Leneva IA, Pecheur EI, et al. Arbidol: a broad-spectrum antiviral compound that blocks viral fusion. Curr Med Chem 2008;15:997-1005. [Crossref] [PubMed]