Potential clinical benefits of cell therapy in coronary heart disease: an update
Introduction
Prevention is the only chance to reduce morbidity and mortality from myocardial infarction (MI), and current therapies cannot restore the lost cardiac muscle tissue. In addition, human heart has a very limited regenerating potential, and common strategies aimed at preventing MI events are not always effective (1). Moreover, the only cure for patients with advanced heart failure (HF) is heart transplantation (2). Therefore, it is necessary to develop innovative therapies to repair the damaged cardiac muscle and improve the quality of patients’ life. This goal could be reached by applying cell therapy, the essence of regenerative medicine, to coronary heart disease (CHD). So far, several preclinical and clinical studies have been pursuing this strategy, which implies the transplantation of autologous stem or progenitor cells into the damaged myocardium in order to restore the lost function.
Unfortunately, clinical results are far from being satisfactory, because of the contrasting evidence about the benefits deriving from cell therapy in CHD. Indeed, even though some clinical trials report promising data, others do not show improvement in heart function after cell therapy. The only clear advantage reported in most of the clinical studies, is the reduction of the infarcted area and of non-functional scarring tissue. This review provides a critical update on the clinical studies evaluating the efficacy of cell therapy applied to CHD.
Cell sources
The final outcome of an ischemic damage to the myocardium is the formation of non-functional scar tissue. Therefore, modern approaches for MI are aimed at restoring the contractile function of infarcted myocardium. In particular, transplantation of stem and progenitor cells has been widely studied. Preclinical studies on small and large mammals have used different cell types, such as embryonic stem cells (ESCs), induced pluripotent stem cells (iPSCs), skeletal myoblasts (Sk-Mbs), adult bone marrow-derived mononuclear cells (BM-MNCs), adult bone marrow-derived mesenchymal cells (BM-MSCs), endothelial progenitor cells (EPCs), adipose-derived stem cells (Ad-SCs), adipose- derived MSCs (Ad-MSCs), umbilical cord blood mesenchymal stem cells (UC-MSCs), cardiac stem cells (CSCs), and pericytes (1,3,4). Some of these studies proved that cell therapy can favor the repair of the damaged heart tissue (5-7). However, due to several concerns about the therapeutic use of certain types of stem or precursor cells, recent clinical trials mainly focused on bone marrow-derived cells, and in particular on BM-MNCs and on BM-MSCs (Tables 1,2). Especially, BM-MSCs have been the preferred source for cell therapy in MI.
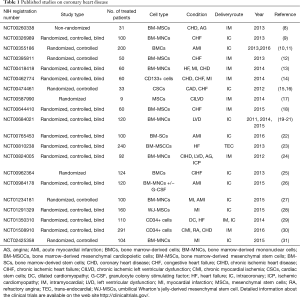
Full table
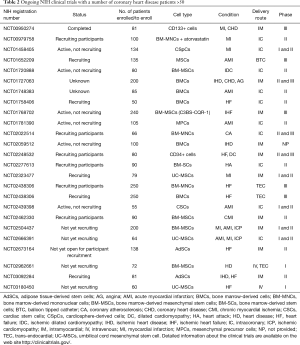
Full table
ESCs are totipotent cells isolated from the inner mass of the blastocyst. These cells can differentiate into all the cell types of an organism, but safety and ethical issues limit their use in clinical practice (32). iPSCs are pluripotent cells derived from genetically reprogrammed adult differentiated cells. These cells can generate cell types from all three germ layers including cardiomyocytes (CMs) (33). The use of iPSC-derived CMs (iPSC-CMs) for clinical practice is encouraged by the good results obtained in preclinical studies. Nonetheless, many issues, such as a standardized protocol for iPSC-CM generation, safety, expensiveness of the procedures remain still unsolved and the time for this type of cells to be introduced into clinical trials has yet to come (33).
Sk-Mbs derive from satellite cells, the quiescent skeletal muscle precursors residing under the basal membrane of muscle fibres (34). Sk-Mbs have been transplanted in ischemic myocardium to repair damaged tissue, but, due to the limited evidence regarding their efficacy and safety, their use in clinical trials is very restricted (35).
BM-MNCs comprise hematopoietic stem and progenitor cells, mesenchymal stromal cells, embryonic-like stem cells, multipotent adult progenitor cells, hemangioblasts, EPCs and tissue- committed stem cells (36). As for the delivery strategies, intramyocardial and intracoronary injections seem to be the preferred routes.
Given the large spectrum of cell types included in the population of BM-MNCs, clinical trials have been performed on specific subpopulations of BM-MNCs, such as CD34+ and CD133+ cells. CD34+ and CD133+ are markers identifying also hematopoietic stem cells and endothelial progenitor cells which can differentiate into different types of adult blood and vascular cells.
BM-MSCs are multipotent cells that, under the appropriate stimuli, can differentiate into many tissues, such as bone, cartilage, fat, muscle, tendon.
EPCs are circulating cells derived from hematopoietic stem cells, that are capable of neovasculogenesis (37). These cells belong to the population of BM-derived cells and are characterized by specific surface markers such as CD31, CD34, and CD133 (38). Several studies demonstrated the potential benefits of EPCs in the treatment of MI, however very few are the clinical trials using selected EPCs for the treatment of patients with ischemic heart disease (39).
Adipose tissue contains mesenchymal stem cells (MSCs) and stem cells that can be used for cardiac repair strategies, as demonstrated in rodent models. In these studies, intramyocardial injection of adipose tissue-derived cells improved the recovery from MI (33). In addition, recent studies in humans have shown the beneficial effects of Ad-SCs transplantation in patients with ischemic cardiomyopathy (40).
As far as umbilical cord-blood cells, a heterogeneous population of cells including hematopoietic stem cells, MSCs, and somatic stem cells, their use in CVD is very limited, although these cells have high proliferative capacity and can give rise to different cell types, among which cardiomyocytes (41).
CSCs are multipotent stem cells residing in the heart with self-renewal and cardiac repair properties (42,43). These cells can be isolated also by spheroid clusters, named cardiospheres (CSpCs), which derive from primary culture of myocardial biopsies (44). Recently, the effectiveness of these cardiosphere-derived cells in post-ischemic heart failure has been challenged in studies on rats (45).
Pericytes are cells of the microvascular wall, localised below the basal lamina underlying the endothelial cell layer of the microvessels. It has been demonstrated that these cells, in injured heart, can secrete different factors promoting angiogenesis, reducing fibrosis and apoptotic cell death in the damaged area (46-49). Moreover, intramyocardial injection of pericytes, together with cardiac stem cells, enhanced the repair of infarcted mouse hearts (50). Nevertheless, data on the beneficial effects of these cells on cardiac repair is limited, thus preventing their use in clinical trials.
Although there are many factors that limit the direct contribution of transplanted cells to myocardial repair process, such as cell engraftment, survival, proliferation, differentiation, and integration into the host tissue, benefits of this approach can last very long after the intervention, possibly because of the activation of endogenous repair process (1,51).
Delivery routes
In the clinical setting, intravenous, intracoronary, and intramyocardial injections are the three delivery strategies that have been used for cell transplantation. Each of them has advantages and drawbacks, thus an ultimate preferred procedure is not available yet. Nevertheless, in recent clinical trials, intracoronary and intramyocardial injections are the two most frequently used delivery routes for cell therapy (Table 1) (52).
Nowadays, in order to improve the benefits obtained with cell therapy, novel solutions are being investigated, such as those involving cell transplants supported by the simultaneous delivery of pro-repair drugs, synthetic scaffolds and growth factors (4).
Recent published clinical trials
Over the last 5 years, 20 clinical studies have been published on the use of cell therapy for CHD-derived conditions, such as acute myocardial infarction (AMI), CHD, left ventricular dysfunction (LVD), MI, angina (AG), HF, congestive heart failure (CHF) etc. (https://clinicaltrials.gov/). Among these studies, 13 (65%) gave positive results, whereas 7 (35%) gave negative results regarding functional recovery of the heart. Nevertheless, cell therapy led to a reduction of scar size, even in cases where there was no clear recovery of cardiac function in terms of ejection fraction. As previously stated, there is not a defined preference for a single delivery route, but almost all the studies used intracoronary or intramyocardial injections. Regarding the source of cells, there is a wide consensus on bone marrow cells (BMCs), due to the ease of BMC isolation and culture, as well as the presence of different multipotent stem cells in bone marrow (BM) (36). In particular, clinical studies used either the heterogeneous population of BMCs, or different subpopulations of BMCs, such as BM-MNCs, BM-MSCs, BM-CD34+, BM-CD133+. The advantage of using the whole population of BMCs derives from its heterogeneity, given that it is still unknown what type of cell from the BM could be effective for cell therapy in CHD. On the other hand, the use of a very defined population of cells could be preferred to reach a standardized protocol.
The 13 clinical trials that yielded positive results are summarized below and reported in Table 1. In the NCT00962364, BMCs have been delivered by intracoronary injection in patients affected by chronic ischemic HF, resulting in an improvement of the cardiopulmonary exercise capacity. NCT00395811 trial showed that, at 12-month follow-up, the intramyocardial injection of BM-MNCs in patients with chronic MI during coronary artery bypass graft, led to a 5.5% improvement of the left ventricular ejection fraction (LVEF) and a significant improvement in contractility, without any variation in the infarct size. NCT00260338 trial demonstrated that intramyocardial delivery of BM-MSCs, in patients with CHD and refractory angina, improved exercise time, angina class, and weekly number of angina attacks. In NCT00474461, intracoronary infusion of autologous CSCs in patients with heart failure, after MI, improved left ventricular systolic function, reduced the infarct size and enhanced the viability of the myocardium. In NCT00587990, autologous MSCs have been injected intramyocardially in patients with chronic ischemic l LVD secondary to MI, and undergoing coronary artery bypass grafting. As result, the treatment reduced the scar size and improved the perfusion and contractile properties of the injected myocardium areas. In NCT01291329 trial, 116 patients with acute ST-elevation MI (STEMI) were subjected to intracoronary injection of umbilical cord Wharton's jelly MSCs. As result, the treatment led, at 18 months, to an increase in myocardial viability and perfusion in the ischemic area, to an increase in LVEF and a decrease in left ventricular end- systolic volumes (LVESV) and end-diastolic volumes. NCT00326989 trial showed that, in patients with HF, application of BMCs, after cardiac shock wave pretreatment, led to an increased LVEF, regional wall thickening, and reduction in the frequency of major adverse cardiac events. In NCT00644410 trial, intramyocardial injection of BM-MSCs in patients with congestive heart failure (CHF) caused a reduction in LVESV, and an improvement in LVEF, stroke volume, and myocardial mass. In NCT00810238 trial, BM-MSCs were differentiated into the cardiac lineage and injected intramyocardially in patients with chronic heart failure. As result, LVESV was reduced, and LVEF, 6-min walk distance, composite clinical score, physical performance, and event-free survival were improved. NCT01350310 trial demonstrated that transendocardial (TEC) administration of CD34+ cells in patients with ischemic cardiomyopathy led to an improvement in LVEF, an increase in 6-minute walk distance and a decrease in plasma concentration of the N-terminal pro B-type natriuretic peptide. In another trial with CD34+ cells (NCT01508910), intramyocardial injection of autologous cells in patients with refractory angina led to an improvement in total exercise time at 12 months and a reduced frequency of angina. In NCT02425358 trial, BM-MNCs were delivered into the coronary arteries of MI patients with ST-elevation, who underwent percutaneous coronary intervention. In the first 7 days following percutaneous coronary intervention, cell therapy increased LVEF, decreased LVESV, and improved myocardial perfusion. Intracoronary injection of BM-MNCs in MI patients enrolled in the clinical trial NCT01234181 showed improvement in left ventricular end-diastolic volume (LVEDV) and LVESV at 6 and 12 months. On the contrary, no enhancement was recorded in the LVEF.
In contrast with the previously cited clinical trials, seven published studies presented negative results for the use of cell therapy in ischemia-derived MI conditions: NCT00684021, NCT00418418, NCT00462774, NCT00984178, NCT00355186, NCT00765453, NCT00824005 (Table 1). These studies transplanted mainly BMCs either by intracoronary or intramyocardial delivery routes, and were applied mostly to patients affected by ischemic cardiomyopathies.
Ongoing clinical trials
Interestingly, the ongoing clinical trials (Table 2), adopt the same delivery routes which have been used in the recently published studies, that is intramyocardial and intracoronary injections. On the contrary, cell type and sources that have been used in these more recent studies are clearly different from the past. In fact, many running clinical trials are based on the use of BMCs, but a significant percentage of studies (28%) utilizes different cell types and sources, such as cardiac stem cells, cardiac progenitor cells, adipose-derived stem cells and MSCs derived from umbilical cord blood. The clinical trial NCT01458405 is based on the injection of allogeneic cardiosphere-derived cells (CAP-1002) in the coronary artery of MI patients. This study is aimed at verifying the safeness and effectiveness of the procedure, as measured by the size of the infarcted area. Intracoronary administration of allogeneic CSCs is the therapeutic strategy to treat patients with STEMI in NCT02439398 trial. Three clinical trials are based on the use of UC-MSCs: NCT02666391, NCT02323477 and NCT03180450. The study NCT02666391 is assessing the safety and efficacy of the intracoronary injection of UC-MSCs in patients with ischemic heart diseases; the clinical trial NCT02323477 is evaluating the efficacy of intramyocardial injection of UC-MSCs in patients with MI; in the clinical trial NCT03180450, allogeneic umbilical cord mesenchymal stem cells will be transplanted intravenously in patients with heart failure.
As for the use of AdSCs for the treatment of patients with LVEF ≤45% and heart failure, there are two clinical trials: NCT02673164 and NCT03092284. In these studies, the regenerative potential of intramyocardial injection of AdSCs will be evaluated. As already stated, the choice of cell type and source is of primary importance for the outcome of any cell-based strategy, as also highlighted by Cogle and collaborators, who studied the cellular composition of BM in patients with ischemic heart disease and severe LVD (53). Therefore, further knowledge is needed, but the enrichment of this research field with new factors and methods could push forward the use of cell therapy in CVD.
Novel strategies for cardiac repair
Given the limited efficacy of current cell therapies for MI, several different approaches are being investigated (Figure 1). As an example, the group of Wai and collaborators has recently studied the role of follistatin-like-protein 1 (FSTL1) in the activation of vascular remodeling and cardiomyocyte proliferation (54). Based on the evidence that cardiac FSTL1 expression is lost upon MI, authors hypothesized that the recovery of the myocardial activity of FSTL1 would activate cardiomyocyte proliferation, thus restoring the function of the damaged heart (54). Indeed, transplantation of collagen patches loaded with human FSTL1 to the epicardial surface of mouse infarcted hearts increased the formation of new vessels, the number of dividing cardiomyocytes and partially restored myocardial functions. This evidence highlights the potential of biomaterial-based strategies to treat patients affected by MI. In MI mice, the subcutaneous inguinal injection of tolerogenic dendritic cells (bone marrow-derived dendritic cells treated with tumor necrosis factor-α and cardiac lysate from MI mice) improved wound remodeling, left ventricular systolic function and survival (55). Very recently, the combination of genetic engineering with cell therapy has led to an improvement in the treatment of MI. Indeed, in rat models of MI, both the transplantation of eNOS-overexpressing BM-MSCs and HGF gene-engineered skeletal myoblasts significantly improved the repair and the functional recovery of the infarcted hearts (56,57). Interestingly, epigenetics has been clearly demonstrated to participate to the pro-fibrotic response of injured myocardium (58).
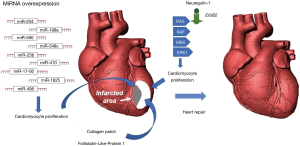
Several in vivo and in vitro studies demonstrated the role of miRNAs, in promoting cardiac muscle repair by stimulating cardiomyocyte proliferation and differentiation (59-61). In particular, miR-17-92, miR-23b, miR-199a, miR-204, miR-410, miR-495, miR-548c, miR-590, and miR-1825 can activate cardiomyocyte proliferation (59,60,62). Moreover, the inhibition of miRNA-23a and miRNA-92a expression reduced apoptotic death of rat cardiomyocytes after MI (63). Recent therapeutic approaches on MI explored also the use of exosomes to promote the repair of infarcted myocardium.
Exosomes are small endosomal membrane-bound vesicles secreted by cells for exchanging proteins, mRNAs and miRNAs. As reported in preclinical studies, exosomes from cardiac cells, MSCs and stem cells can be effective in the functional recovery of cardiac tissue from MI (64-67). Another very promising approach is based on the delivery of pro-repair factors, such as neuregulin, into infarcted hearts using biodegradable microparticles (68,69). These microparticles can escape the immune system and constantly release the active compound for 12 weeks, until they degrade. Additionally, a very interesting research led to the development of a spraying system for a mini-invasive application of platelet fibrin gel on the surface of infarcted mouse hearts. The layered polymerizable biomaterial patch attenuated LV remodeling, reduced cardiomyocyte death and stimulated myocardial repair, thus ameliorating heart function (70). However, powerful methods to monitor the effect of cell therapy are also needed (71).
Conclusions
The clinical use of cell therapy in CHD has not reached a mature stage yet, where defined and standardized procedures are available. A consensus on cell types, number of cells to implant, delivery routes, transplantation timing is still lacking, and the number of variables to account for is huge. Moreover, despite the increasing amount of data resulting from all the preclinical and clinical studies realized so far, it is difficult to compare different studies and to extract conclusive information from them, because of the differences in the strategies adopted in the studies. Nevertheless, an important point to underline is that autologous cell therapy is safe, and successful results have been collected anyway by different groups. The potential benefits of an effective cell therapy in CHD are so important that even if its efficacy has been, so far, variable and limited, it is still very worthwhile to invest in this field.
Acknowledgements
None.
Footnote
Conflicts of Interest: The authors have no conflicts of interest to declare.
References
- Sommese L, Zullo A, Schiano C, et al. Possible muscle repair in the human cardiovascular system. Stem Cell Rev 2017;13:170-91. [Crossref] [PubMed]
- Stehlik J, Edwards LB, Kucheryavaya AY, et al. The registry of the international society for heart and lung transplantation: twenty-eighth adult heart transplant report--2011. J Heart Lung Transplant 2011;30:1078-94. [Crossref] [PubMed]
- Behfar A, Crespo-Diaz R, Terzic A, et al. Cell therapy for cardiac repair - lessons from clinical trials. Nat. Rev. Cardiol 2014;11:232-46. [Crossref] [PubMed]
- Grimaldi V, Mancini FP, Casamassimi A, et al. Potential benefits of cell therapy in coronary heart disease. J Cardiol 2013;62:267-76. [Crossref] [PubMed]
- Rabbani S, Soleimani M, Sahebjam M, et al. Effects of endothelial and mesenchymal stem cells on improving myocardial function in a sheep animal model. J Tehran Heart Cent 2017;12:65-71. [PubMed]
- Sharp TE, Schena GJ, Hobby AR, et al. Cortical bone stem cell therapy preserves cardiac structure and function after myocardial infarction. Circ Res 2017;121:1263-78. [Crossref] [PubMed]
- Pennella S, Reggiani Bonetti L, Migaldi M, et al. Does stem cell therapy induce myocardial neoangiogenesis? Histological evaluation in an ischemia/reperfusion animal model. J Cardiovasc Med (Hagerstown) 2017;18:277-82. [Crossref] [PubMed]
- Mathiasen AB, Haack-Sørensen M, Jørgensen E, et al. Autotransplantation of mesenchymal stromal cells from bone-marrow to heart in patients with severe stable coronary artery disease and refractory angina--final 3-year follow-up. Int J Cardiol 2013;170:246-51. [Crossref] [PubMed]
- Assmus B, Walter DH, Seeger FH, et al. Effect of shock wave-facilitated intracoronary cell therapy on LVEF in patients with chronic heart failure: the CELLWAVE randomized clinical trial. JAMA 2013;309:1622-31. Erratum in: JAMA 2013;309:1994. [Crossref] [PubMed]
- Sürder D, Manka R, Lo Cicero V, et al. Intracoronary injection of bone marrow-derived mononuclear cells early or late after acute myocardial infarction: effects on global left ventricular function. Circulation. 2013;127:1968-79. [Crossref] [PubMed]
- Sürder D, Manka R, Moccetti T, et al. Effect of bone marrow-derived mononuclear cell treatment, early or late after acute myocardial infarction: twelve months CMR and long-term clinical results. Circ Res 2016;119:481-90. [Crossref] [PubMed]
- Lu M, Liu S, Zheng Z, et al. A pilot trial of autologous bone marrow mononuclear cell transplantation through grafting artery: a sub-study focused on segmental left ventricular function recovery and scar reduction. Int J Cardiol 2013;168:2221-7. [Crossref] [PubMed]
- Pätilä T, Lehtinen M, Vento A, et al. Autologous bone marrow mononuclear cell transplantation in ischemic heart failure: a prospective, controlled, randomized, double-blind study of cell transplantation combined with coronary bypass. J Heart Lung Transplant 2014;33:567-74. [Crossref] [PubMed]
- Nasseri BA, Ebell W, Dandel M, et al. Autologous CD133+ bone marrow cells and bypass grafting for regeneration of ischaemic myocardium: the Cardio133 trial. Eur Heart J 2014;35:1263-74. [Crossref] [PubMed]
- Chugh AR, Beache GM, Loughran JH, et al. Administration of cardiac stem cells in patients with ischemic cardiomyopathy: the SCIPIO trial: surgical aspects and interim analysis of myocardial function and viability by magnetic resonance. Circulation 2012;126:S54-64. [Crossref] [PubMed]
- Bolli R, Chugh AR, D'Amario D, et al. Cardiac stem cells in patients with ischaemic cardiomyopathy (SCIPIO): initial results of a randomised phase 1 trial. Lancet 2011;378:1847-57. [Crossref] [PubMed]
- Karantalis V, DiFede DL, Gerstenblith G, et al. Autologous mesenchymal stem cells produce concordant improvements in regional function, tissue perfusion, and fibrotic burden when administered to patients undergoing coronary artery bypass grafting: The Prospective Randomized Study of Mesenchymal Stem Cell Therapy in Patients Undergoing Cardiac Surgery (PROMETHEUS) trial. Circ Res 2014;114:1302-10. [Crossref] [PubMed]
- Mathiasen AB, Qayyum AA, Jørgensen E, et al. Bone marrow-derived mesenchymal stromal cell treatment in patients with severe ischaemic heart failure: a randomized placebo-controlled trial (MSC-HF trial). Eur Heart J 2015;36:1744-53. [Crossref] [PubMed]
- Traverse JH, Henry TD, Ellis SG, et al. Effect of intracoronary delivery of autologous bone marrow mononuclear cells 2 to 3 weeks following acute myocardial infarction on left ventricular function: the LateTIME randomized trial. JAMA 2011;306:2110-9. [Crossref] [PubMed]
- Traverse JH, Henry TD, Pepine CJ, et al. One-year follow-up of intracoronary stem cell delivery on left ventricular function following ST-elevation myocardial infarction. JAMA 2014;311:301-2. Erratum in: JAMA 2015;314:86. [Crossref] [PubMed]
- Schutt RC, Trachtenberg BH, Cooke JP, et al. Bone marrow characteristics associated with changes in infarct size after STEMI: a biorepository evaluation from the CCTRN TIME trial. Circ Res 2015;116:99-107. [Crossref] [PubMed]
- Choudry F, Hamshere S, Saunders N, et al. A randomized double-blind control study of early intra-coronary autologous bone marrow cell infusion in acute myocardial infarction: the REGENERATE-AMI clinical trial. Eur Heart J 2016;37:256-63. [Crossref] [PubMed]
- Bartunek J, Behfar A, Dolatabadi D, et al. Cardiopoietic stem cell therapy in heart failure: the C-CURE (Cardiopoietic stem Cell therapy in heart failURE) multicenter randomized trial withlineage-specified biologics. J Am Coll Cardiol. 2013;61:2329-2338. Erratum in: J Am Coll Cardiol 2013;62:2457-8. [Crossref] [PubMed]
- Perin EC, Willerson JT, Pepine CJ, et al. Effect of transendocardial delivery of autologous bone marrow mononuclear cells on functional capacity, left ventricular function, and perfusion in chronic heart failure: the FOCUS-CCTRN trial. JAMA 2012;307:1717-26. [Crossref] [PubMed]
- Honold J, Fischer-Rasokat U, Seeger FH, et al. Impact of intracoronary reinfusion of bone marrow-derived mononuclear progenitor cells on cardiopulmonary exercise capacity in patients with chronic postinfarction heart failure. Clin Res Cardiol 2013;102:619-25. [Crossref] [PubMed]
- San Roman JA, Sánchez PL, Villa A, et al. Comparison of different bone marrow-derived stem cell approaches in reperfused STEMI. a multicenter, prospective, randomized, open- labeled TECAM Trial. J Am Coll Cardiol 2015;65:2372-82. [Crossref] [PubMed]
- Hu X, Huang X, Yang Q, et al. Safety and efficacy of intracoronary hypoxia-preconditioned bone marrow mononuclear cell administration for acute myocardial infarction patients: The CHINA-AMI randomized controlled trial. Int J Cardiol 2015;184:446-51. [Crossref] [PubMed]
- Gao LR, Chen Y, Zhang NK, et al. Intracoronary infusion of Wharton's jelly-derived mesenchymal stem cells in acute myocardial infarction: double-blind, randomized controlled trial. BMC Med 2015;13:162. [Crossref] [PubMed]
- Poglajen G, Sever M, Cukjati M, et al. Effects of transendocardial CD34+ cell transplantation in patients with ischemic cardiomyopathy. Circ Cardiovasc Interv 2014;7:552-9. [Crossref] [PubMed]
- Povsic TJ, Henry TD, Traverse JH, et al. The RENEW Trial: Efficacy and Safety of Intramyocardial Autologous CD34(+) Cell Administration in Patients With Refractory Angina. JACC Cardiovasc Interv 2016;9:1576-85. [Crossref] [PubMed]
- Huang R, Yao K, Sun A, et al. Timing for intracoronary administration of bone marrow mononuclear cells after acute ST-elevation myocardial infarction: a pilot study. Stem Cell Res Ther 2015;6:112. [Crossref] [PubMed]
- Hotkar AJ, Balinsky W. Stem cells in the treatment of cardiovascular disease - an overview. Stem Cell Rev 2012;8:494-502. [Crossref] [PubMed]
- Faiella W, Atoui R. Therapeutic use of stem cells for cardiovascular disease. Clin Transl Med 2016;5:34. [Crossref] [PubMed]
- Buckingham M, Montarras D. Skeletal muscle stem cells. Curr Opin Genet Dev 2008;18:330-6. [Crossref] [PubMed]
- Durrani S, Konoplyannikov M, Ashraf M, et al. Skeletal myoblasts for cardiac repair. Regen Med 2010;5:919-32. [Crossref] [PubMed]
- Cuende N, Rico L, Herrera C. Concise review: bone marrow mononuclear cells for the treatment of ischemic syndromes: medicinal product or cell transplantation? Stem Cells Transl Med 2012;1:403-8. [Crossref] [PubMed]
- Lee JW, Lee SH, Youn YJ, et al. A randomized, open-label, multicenter trial for the safety and efficacy of adult mesenchymal stem cells after acute myocardial infarction. J Korean Med Sci 2014;29:23-31. [Crossref] [PubMed]
- Hager G, Holnthoner W, Wolbank S, et al. Three specific antigens to isolate endothelial progenitor cells from human liposuction material. Cytotherapy 2013;15:1426-35. [Crossref] [PubMed]
- Cheng Y, Jiang S, Hu R, et al. Potential mechanism for endothelial progenitor cell therapy in acute myocardial infarction: Activation of VEGF- PI3K/Akte-NOS pathway. Ann Clin Lab Sci 2013;43:395-401. [PubMed]
- Henry TD, Pepine CJ, Lambert CR, et al. The Athena trials: Autologous adipose-derived regenerative cells for refractory chronic myocardial ischemia with left ventricular dysfunction. Catheter Cardiovasc Interv 2017;89:169-77. [Crossref] [PubMed]
- Weiss ML, Troyer DL. Stem cells in the umbilical cord. Stem Cell Rev 2006;2:155-62. [Crossref] [PubMed]
- Beltrami AP, Barlucchi L, Torella D, et al. Adult cardiac stem cells are multipotent and support myocardial regeneration. Cell 2003;114:763-76. [Crossref] [PubMed]
- Laugwitz KL, Moretti A, Lam J, et al. Postnatal isl1+ cardioblasts enter fully differentiated cardiomyocyte lineages. Nature 2005;433:647-53. [Crossref] [PubMed]
- Smith RR, Barile L, Cho HC, et al. Regenerative potential of cardiosphere-derived cells expanded from percutaneous endomyocardial biopsy specimens. Circulation 2007;115:896-908. [Crossref] [PubMed]
- Kasai-Brunswick TH, Costa AR, Barbosa RA, et al. Cardiosphere-derived cells do not improve cardiac function in rats with cardiac failure. Stem Cell Res Ther 2017;8:36. [Crossref] [PubMed]
- Armulik A, Genove G, Betsholtz C. Pericytes: developmental, physiological, and pathological perspectives, problems, and promises. Dev Cell 2011;21:193-215. [Crossref] [PubMed]
- Chen CW, Montelatici E, Crisan M, et al. Perivascular multi-lineage progenitor cells in human organs: regenerative units, cytokine sources or both? Cytokine Growth Factor Rev 2009;20:429-34. [Crossref] [PubMed]
- Katare R, Riu F, Mitchell K, et al. Transplantation of human pericyte progenitor cells improves the repair of infarcted heart through activation of an angiogenic program involving micro-RNA- 132. Circ Res 2011;109:894-906. [Crossref] [PubMed]
- Chen CW, Okada M, Proto JD, et al. Human pericytes for ischemic heart repair. Stem cells 2013;31:305-16. [Crossref] [PubMed]
- Avolio E, Meloni M, Spencer HL, et al. Combined intramyocardial delivery of human pericytes and cardiac stem cells additively improves the healing of mouse infarcted hearts through stimulation of vascular and muscular repair. Circ Res 2015;116:e81-94. [Crossref] [PubMed]
- Hao M, Wang R, Wang W. Cell Therapies in Cardiomyopathy: Current Status of Clinical Trials. Anal Cell Pathol (Amst) 2017;2017. [Crossref] [PubMed]
- Chong JJ, Murry CE. Cardiac regeneration using pluripotent stem cells--progression to large animal models. Stem Cell Res 2014;13:654-65. [Crossref] [PubMed]
- Cogle CR, Wise E, Meacham AM, et al. A Detailed Analysis of Bone Marrow from Patients with Ischemic Heart Disease and Left Ventricular Dysfunction: BM CD34, CD11b and clonogenic capacity as biomarkers for clinical outcomes. Circ Res 2014;115:867-74. [Crossref] [PubMed]
- Wei K, Serpooshan V, Hurtado C, et al. Epicardial FSTL1 reconstitution regenerates the adult mammalian heart. Nature. 2015;525:479-85. [Crossref] [PubMed]
- Choo EH, Lee JH, Park EH, et al. Infarcted myocardium-primed dendritic cells improve remodeling and cardiac function after myocardial infarction by modulating the regulatory T cell and macrophage polarization. Circulation 2017;135:1444-57. [Crossref] [PubMed]
- Chen L, Zhang Y, Tao L, et al. Mesenchymal stem cells with eNOS over-expression enhance cardiac repair in rats with myocardial infarction. Cardiovasc Drugs Ther 2017;31:9-18. [Crossref] [PubMed]
- Rong SL, Wang XL, Zhang CY, et al. Transplantation of HGF gene-engineered skeletal myoblasts improve infarction recovery in a rat myocardial ischemia model. PLoS One 2017;12. [Crossref] [PubMed]
- Grimaldi V, De Pascale MR, Zullo A, et al. Evidence of epigenetic tags in cardiac fibrosis. J Cardiol 2017;69:401-8. [Crossref] [PubMed]
- Chen J, Huang ZP, Seok HY, et al. Mir-17-92 cluster is required for and sufficient to induce cardiomyocyte proliferation in postnatal and adult hearts. Circ Res 2013;112:1557-66. [Crossref] [PubMed]
- Pandey R, Ahmed RPH. MicroRNAs inducing proliferation of quiescent adult cardiomyocytes. Cardiovasc Regen Med 2015.2. [PubMed]
- Liang D, Li J, Wu Y, et al. miRNA204 drives cardiomyocyte proliferation via targeting Jarid2. Int J Cardiol 2015;201:38-48. [Crossref] [PubMed]
- Clark AL, Naya FJ. MicroRNAs in the myocyte enhancer factor 2 (MEF2)-regulated Gtl2-Dio3 noncoding RNA locus promote cardiomyocyte proliferation by targeting the transcriptional coactivator Cited2. J Biol Chem 2015;290:23162-72. [Crossref] [PubMed]
- Song YS, Joo HW, Park IH, et al. Bone marrow mesenchymal stem cell-derived vascular endothelial growth factor attenuates cardiac apoptosis via regulation of cardiac miRNA-23a and miRNA-92a in a rat model of myocardial infarction. PLoS One 2017;12. [Crossref] [PubMed]
- Kishore R, Khan M. More than tiny sacks: Stem cell exosomes as cell-free modality for cardiac repair. Circ Res 2016;118:330-43. [Crossref] [PubMed]
- Gallet R, Dawkins J, Valle J, et al. Exosomes secreted by cardiosphere-derived cells reduce scarring, attenuate adverse remodelling, and improve function in acute and chronic porcine myocardial infarction. Eur Heart J 2017;38:201-11. [PubMed]
- Zhang H, Xiang M, Meng D, et al. Inhibition of myocardial ischemia/reperfusion injury by exosomes secreted from mesenchymal stem cells. Stem Cells Int 2016;2016. [Crossref] [PubMed]
- Boulanger CM, Loyer X, Rautou PE, Amabile N. Extracellular vesicles in coronary artery disease. Nat Rev Cardiol 2017;14:259-72. [Crossref] [PubMed]
- Pascual-Gil S, Simón-Yarza T, Garbayo E, et al. Tracking the in vivo release of bioactive NRG from PLGA and PEG–PLGA microparticles in infarcted hearts. J Control Release 2015;220:388-96. [Crossref] [PubMed]
- Yutzey KE. Regenerative biology: Neuregulin 1 makes heart muscle. Nature 2015;520:445-6. [Crossref] [PubMed]
- Tang J, Vandergriff A, Wang Z, et al. A regenerative cardiac patch formed by spray painting of biomaterials onto the heart. Tissue Eng Part C Methods 2017;23:146-55. [Crossref] [PubMed]
- Grimaldi V, Schiano C, Casamassimi A, et al. Imaging techniques to evaluate cell therapy in peripheral artery disease: state of the art and clinical trials. Clin Physiol Funct Imaging 2016;36:165-78. [Crossref] [PubMed]