Cardiac contractility modulation improves left ventricular systolic function partially via miR-25 mediated SERCA2A expression in rabbit trans aortic constriction heart failure model
Introduction
Heart failure (HF) with severely impaired left ventricular (LV) systolic function, and progressive decrease in cardiac output has been associated with poor life quality and reduced life expectancy. Also it is one of the main causes of death in developed countries (1). However pharmacological therapy has shown limited efficacy in increasing the myocardial contractility as well as inability in preventing an advanced HF from ulterior deterioration (2,3).
Cardiac contractility modulation (CCM) is a novel therapeutic method which delivers electrical current stimulation during the ventricular absolute refractory period (4). It has proven to be effective in improving cardiac contractility, thus presenting advanced HF patients with narrow QRS duration a strategy, as they are not candidates for cardiac resynchronization therapy (CRT) (1,4-10).
Previous researches have revealed that CCM can partially restore HF-induced calcium handle protein sarcoplasmic reticulum calcium ATPase (SERCA2A) reduction (4,6). However, the mechanism is still unclear and need to be further investigated (4). SERCA2A is an important calcium handle protein associated with LV function changes (11). The cardiac systolic and diastolic function are determined by rapid changes of calcium in cardiomyocytes. During the systole period, extracellular calcium is transferred into the cytosol via voltage gated L-type calcium channel, further combined with ryanodine receptor (RyR), which in turn triggers calcium influx to the cytosol from sarcoplasmic reticulum (SR). During diastole period, calcium is removed mainly by SERCA2A (12,13). Thus, the abnormal expression of SERCA2A is regarded as a hallmark of cardiac dysfunction (11).
Recently, it has been discovered that microRNAs have an important role in the pathological process of HF (14,15). In addition, one important study demonstrated that the SERCA2A mRNA and protein expression levels are negatively correlated with the expression level of miR-25 in trans aortic constriction (TAC)-induced HF mice model (16). In the present research, we hypothesized that the inhibition of miR-25 (upregulation of SERCA2A) might be regulated by CCM, thus further improving TAC-induced HF LV systolic function.
Methods
Animals and reagents
New Zealand rabbits, 2.3–2.5 kg weight, were bought from China Medical University animal center. All the animals were housed in an environment with 22±1 °C, relative humidity of 50%±1% and a light/dark cycle of 12/12 hr. All animal studies (including the euthanasia procedure) were done in compliance with the regulations and guidelines of China Medical University institutional animal care (Committee on Care and Use of Laboratory Animals) and were conducted according to the Helsinki Declaration as revised in 2013.
All the chemicals were purchased from Sigma Aldrich Corporation (St Louis, MO, USA) unless an alternate source was specifically referenced.
Group setting
The experiment design included two parts. The first part consisted of dividing 25 rabbits into 5 groups: control group (CT, n=5), AAV9-miR-25 injection group (miR-25, n=5), AAV9-unloading injection group (miR-25 control, n=5), anti-miR-25 administration group (anti-miR-25, n=5), anti-miR-25 negative control group (anti-miR-25 control, n=5) to explore whether miR-25 can mediate SERCA2A expression in rabbits. The second part consisted of dividing 35 rabbits into 7 groups: sham operation group (SHM, n=5), 16 weeks of TAC-induced HF group (HF, n=5), 12 weeks of TAC-induced HF followed by 4 weeks of CCM group (HF + CCM, n=5), followed by 4 weeks of anti-miR-25 group (HF + anti-miR-25, n=5) or anti-miR-25 negative control group (HF + anti-miR-25 control, n=5), followed by 4 weeks of CCM and AAV9-miR-25 group (HF + CCM + miR-25, n=5) or AAV9-unloading injection group (HF + CCM + miR-25 control, n=5), to explore the mechanisms underlying the CCM treatment and LV systolic function improvement in TAC-induce HF models.
Animal operations
The TAC-induced HF and CCM models in this study were performed according to previously described approaches (6,17). Briefly, all animals were anesthetized using 3% pentobarbital sodium (30 mg/kg) via ear vein, a 25 mL volume of tidal and 50 breaths per minute on a respirator (Harvard Apparatus). This was followed by exposing the heart and dissecting the ascending aorta, then creating a constriction between the left common carotid and the innominate artery. Twelve weeks after operation, the rabbits with the LV ejection fraction (LVEF) ≤40% as HF successfully established (6). For the CCM model, one end of electrodes was sutured to LV free wall or right atrial free wall, while the other end was fixed to the neck for stimulation or sense. SHM group received the same surgical procedure without aortic arch ligation and ventricular pacing. In totally 37 out 45 rabbits survived for further experiment.
At the end of the experiment, the animals were sacrificed, hearts were quickly excised, and the LV myocardium was snap-frozen in liquid nitrogen.
CCM pacing protocols
After successfully established HF, groups assigned for CCM treatment were stimulated with electric current [2 ms, 7 V, Cardiac Stimulator EPS320 (MicroPace, Inc., USA)] during the ventricular absolute refractory period 30 ms after sensing the R-wave. The CCM stimulation was conducted 6 hours a day for total of 4 weeks (6).
Echocardiography examination of LV function
The echocardiography parameters were acquired at the end of 16th weeks, as we previously described (18). Briefly, after animals were anesthetized, two-dimensional images and M-mode tracings were acquired to record the LV end systolic diameters (LVESD), the LV end diastolic diameters (LVEDD), the LV ejection fraction (LVEF) and the LV shortening fractions (LVFS) (GE Vivid Vision).
AAV9-mediated miR-25 overexpression
AAV9-miR-25 and unloading control were established following plasmid transfection described previously (19). Briefly, before transfection, the 293-T cells were cultured for 24 hours in DMEM growth medium supplemented with 10% FBS. The cells were harvested 72 hours later, and the DNase resistant virus was purified with an iodixanol density gradient (Genechem, China), followed by mixing the purified virus into a lactated Ringer’s solution (Genechem, China) by 100K MWCO centrifugal concentrators (Xiangyi, China), and stored under −80 °C. A treatment consisting of 5×1011 vector genomes (vg) per rabbit or unloaded control was injected into the pericardial cavity of the groups assigned to the miR-25 treatment or miR-25 control groups. Two of these animals died during the procedure.
Anti-miR-25 administration
The anti-miR-25 (Genechem, China) and the negative control (Genechem, China) were diluted following the recommendations of the manufacturer. The oligonucleotide was incubated for 20 min at 37 °C before injection. Animals that were assigned the anti-miR-25 or anti-miR-25 control administration received 100 µL of oligonucleotide solution or negative control injection into the jugular vein once a day for 3 days followed by once a week for the following 3 weeks (20).
Immunohistochemistry
Paraffin-embedded cardiac tissue section was incubated in 3% H2O2 for 20 min to reduce endogenous peroxidase activity after being deparaffinized by xylene and rehydrated in graded series of ethanol. To retrieve the antigens, the sections were heated 15 min in the microwave in target retrieval solution (Dako). To block non-specific binding, the slides were incubated in 10% goat serum at room temperature for 2 hours. Slides were then incubated with SERCA2A primary antibody at 4 °C overnight, followed the secondary antibody at 37 °C for 20 min. Binding was visualized with 3,39-diaminobenzidine tetrahydrochloride (DAB).
Western blot
Myocardium was homogenized in lysis buffer. Equal amount of protein lysates was loaded into 10% SDS-polyacrylamide gel, then transferred to Hybond membranes (Amersham, Munich, Germany). Fat-free milk (5%) was used to block membranes at room temperature for 2 hours. Samples were then incubated with primary antibody SERCA2A (1:1,000, Santa Cruz, CA, USA) for 2 hours. The blots were then washed three times in TBST. They were then incubated in the secondary antibody (1:5,000, Proteintech, Proteintech Group) for additional 2 hours. β-actin (1:500, Proteintech, Proteintech Group) was used as control. An enhanced chemiluminescence system was used to visualize protein binding, following manufacturer’s protocol (Santa Cruz Biotechnology, Santa Cruz, CA, USA).
Reverse transcription-polymerase chain reaction (RT-PCR)
Total mRNA from myocardium tissue was isolated using TRIzol (Takara, Kyoto, Japan) following manufacturer’s instruction. Avian myeloblastosis virus reverse and random primers (Takara) were used in RT-PCR. Real-time quantitative PCR were performed for the cDNA amplification by a SYBR Premix Ex Taq™ II kit (Takara), with 18S rRNA used as the inner control. Measurement of miR-25 was done according to manufacturer’s instructions (Genechem, China), with U6 as inner control (Genechem, China). The comparative threshold cycle (Ct) method (2−ΔΔCt) were using for calculating the relative gene expression (21).
Statistical analysis
Statistical analysis was undertaken by SPSS software version 17.0. Mean ± SD was used for continuous variables. For multi-group comparisons, the data was analyzed using either a one-way ANOVA or a M-W U test, LSD was used for post-hoc test. A P value <0.05 was considered as statistically significant.
Results
miR-25 mediated rabbit cardiac SERCA2A expression
Cardiac miR-25 expression was significantly improved in the miR-25 group, while the anti-miR-25 group showed a reduced expression, both compared to the control group (CT group) (Figure 1A). Moreover, the mRNA and protein of SERCA2A expression decreased in the miR-25 group and increased in the anti-miR-25 group, compared to CT group (Figure 1). This result indicates that miR-25 can mediate rabbit cardiac SERCA2A expression in vivo.
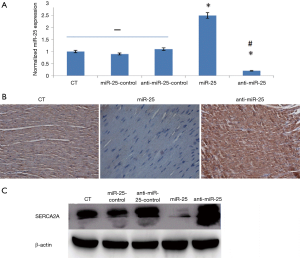
CCM partially resorted TAC-induced LV systolic dysfunction
We further investigated the link between miR-25, SERCA2A, and LV function changes in the SHM group, the HF group and the HF + CCM group (Figure 2). The miR-25 expression was highest in the HF group when compared to SHM and HF + CCM (Figure 2A). Moreover, both mRNA and protein expression of SERCA2A were reduced in the HF group compared to SHM group; contrary, those levels were partially improved by CCM (Figure 2B). Furthermore, the LVEF was significantly reduced in HF group, but partially improved after CCM treatment (Figure 2C, Table 1).
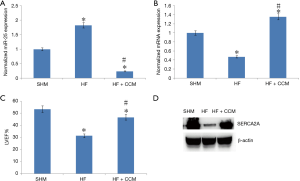
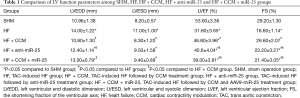
Full table
CCM improved LV systolic function via inhibition of miR-25 expression
The HF, HF + CCM and HF + anti-miR-25 groups were used to investigate the effect of CCM on miR-25 expression, and the relationship among miR-25, SERCA2A, and LV function (Figure 3). Reduced expression of miR-25 was observed in the HF + CCM group and the HF + anti-miR-25 groups compared to HF group (Figure 3A). Conversely, the mRNA and protein of SERCA2A expression was improved in both the HF + anti-miR-25 and the HF + CCM group compared to the HF group, while additional improvement was found in the HF + CCM group (Figure 3B). Moreover, both the HF + anti-miR-25 and the HF + CCM groups partially restored LVEF reduction induced by TAC, with the greatest improvement seen in the HF + CCM group (Figure 3C, Table 1). The results reveal that CCM inhibited miR-25 expression, potentially leading to the improvement of SERCA2A expression and LV function. Also, CCM treatment appears superior to anti-miR-25 treatment in improving SERCA2A expression and LV function.
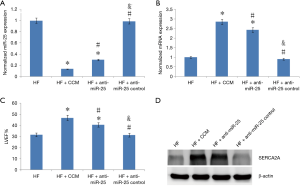
Effect of CCM on LV systolic function after the upregulation of miR-25
In order to further confirm that CCM improves LV function by inhibiting miR-25 mediated SERCA2A expression, samples from the HF group, the HF + CCM group, the HF + CCM + miR-25 group were used (Figure 4). The miR-25 expression was significantly improved in the HF + CCM + miR-25 group compared to the HF + CCM group (Figure 4A). Moreover, the mRNA and protein of SERCA2A expression and LVEF were significantly increased in the HF + CCM group compared to HF group. This improvement was partially neutralized in the HF + CCM + miR-25 group (Figure 4B,C, Table 1).
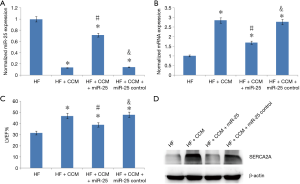
Discussion
The major findings of the present study as follows: (I) the expression of miR-25 was increased while the expression of SERCA2A was decreased in the TAC-induced HF model; (II) miR-25 might play an important role in mediating mRNA and protein of SERCA2A expression, which might further lead to LV function alteration; (III) CCM improves TAC-induced HF LV systolic function, which may be partially related to inhibiting miR-25 expression.
A large number of end-stage HF patients are refractory to standard medical therapy, which has given rise to the development of novel strategies. One of the most prospective applicable approaches is CCM (4). Both preclinical and clinical studies suggest that CCM therapy can improve cardiac contractility (22,23). Animal research has revealed the acute effects of CCM in improving cardiac function might via the stimulating of norepinephrine release, and the chronic effects might be due to the restoration of HF induced SERCA2A, RyR, miR-133, and α-myosin heavy chain (gene) reduction (3,17). However, the underlying mechanism for CCM in remodeling the HF associated molecules is still unclear.
Both CCM and CRT studies suggested that the improvement of LV systolic function might be related to the restoring of HF induced SERCA2A gene changes and calcium channel remodeling, since SERCA2A is an important cardiomyocyte calcium handling protein (1,4-10). The levels of its expression are positively correlated with calcium decay from cytosol. Knockout SERCA2A animals develop LV dysfunction, and when obstructing SERCA2A expression in cardiomyocytes, the HF phenotype was produced (24-28). In our study, decreased SERCA2A expression (both mRNA and protein) were found in the HF group compared with the SHM group, while higher SERCA2A expression accompanied with LV systolic function improvement was observed in the HF + CCM group compared to the HF group. Therefore, we hypothesized that CCM potentially via upregulates SERCA2A expression, and in turn improves TAC-induced HF LV systolic function.
Previous research has shown that miR-25 plays a pivotal role in mediating SERCA2A expression. At least 7 target sites at the 3’UTR of SERCA2A were identified that matched exactly the 2–8 nucleotides of the 5’-end of miR-25 (TargetScan Human v6.2) (Figure 5) (16). We addressed that transfect AAV9-miR-25 to the rabbit cardiac resulted in SERCA2A reduction. Conversely, SERCA2A was upregulated after anti-miR-25 transfected. Our study also revealed that the miR-25 expression improved and SERCA2A expression reduced in the HF group compared to the SHM group, both the HF + CCM group and the HF + anti-miR-25 group had reduced miR-25 expression compared to the HF group. Conversely the SERCA2A expression and LVEF improved in the HF + CCM group and the HF + anti-miR-25 group compared to the HF group. Moreover, upregulating miR-25 expression partially neutralized the CCM effects in improving LV systolic function in the HF + CCM + miR-25 group compared to the HF + CCM group. The present study has demonstrated that CCM might inhibit miR-25 expression, further upregulating SERCA2A expression, which may lead to the improvement of LV systolic function. However, LVEF in the HF + CCM + miR-25 group was only partially neutralized compared to the HF + CCM group, which implies that CCM improves LV systolic function partially via miR-25 mediated SERCA2A expression.
miR-25 is an evolutionarily conserved microRNA which has been demonstrated to possess many target genes, for example insulin, AMOAP1 and ATXN3 genes (29,30). SERCA2A is one of the most important target genes related to cardiac function. In our study, we found the expression of miR-25 increased in the HF + CCM group compared to the HF group. Whether the electric current delivered during the ventricular absolute refractory period by CCM induces the improvement of miR-25 is yet unclear. Interestingly, previous study has found CCM also can improve cardiac miR-133 expression levels, but the specific mechanisms under these changes need to be further studied.
Clinically, researches have demonstrated that CCM therapy can improve cardiac contractility and patients exercise tolerance, without increasing oxygen consumption (22,23). Moreover, contrary to CRT, CCM might be a useful option for both narrow and wide QRS duration HF patients (9,31). However, due to the invasive character, OPTIMIZERTM system (Impulse Dynamics Inc., Orangeburg, SC, USA) is the only clinically CCM to date (3). Moreover, the delivery of CCM signal wastes the battery which shortens the OPTIMIZERTM system use time (3). Therefore, additional studies regarding the mechanism and effect of CCM should be performed to support the wide use of the procedure.
This research also has limitations. This mechanism requires further validation by SERCA2A gene mutation and knock down animal models. Moreover, miR-25 and SERCA2A might be one of the mechanisms that underlie CCM treatment improving LV systolic function. The sample size in this study was also small, and thus large-scale animal and clinical studies are required to further evaluate the safety and mechanism of this promising procedure.
Conclusions
In summary, CCM is an expected therapy for advanced HF, which might work by inhibiting of miR-25 expression, which inhibiting the expression of SERCA2A mRNA and protein expression, thus improving the cardiac contractility. Our research partially revealed the mechanism underlying CCM in improving TAC-induced HF LV systolic function, as well as provided biochemical basis for it to be clinically implement.
Acknowledgements
Funding: This study was supported by National Natural Science Foundation of China (No. 81401413, 81701699) and the Doctoral Initiate Foundation of Liaoning (grant 20170520053).
Footnote
Conflicts of Interest: The authors have no conflicts of interest to declare.
Ethical Statement: The study was approved by the Ethics Committee of China Medical University (AF-SOP-07-1.1-01).
References
- Morita H, Suzuki G, Haddad W, et al. Long-term effects of non-excitatory cardiac contractility modulation electric signals on the progression of heart failure in dogs. Eur J Heart Fail 2004;6:145-50. [Crossref] [PubMed]
- Packer M. The development of positive inotropic agents for chronic heart failure: how have we gone astray? J Am Coll Cardiol 1993;22:119A-26A. [Crossref] [PubMed]
- Winter J, Brack KE, Ng GA. Cardiac contractility modulation in the treatment of heart failure: initial results and unanswered questions. Eur J Heart Fail 2011;13:700-10. [Crossref] [PubMed]
- Butter C, Rastogi S, Minden HH, et al. Cardiac contractility modulation electrical signals improve myocardial gene expression in patients with heart failure. J Am Coll Cardiol 2008;51:1784-9. [Crossref] [PubMed]
- Pappone C, Rosanio S, Burkhoff D, et al. Cardiac contractility modulation by electric currents applied during the refractory period in patients with heart failure secondary to ischemic or idiopathic dilated cardiomyopathy. Am J Cardiol 2002;90:1307-13. [Crossref] [PubMed]
- Ning B, Qi X, Li Y, et al. Biventricular pacing cardiac contractility modulation improves cardiac contractile function via upregulating SERCA2 and miR-133 in a rabbit model of congestive heart failure. Cell Physiol Biochem 2014;33:1389-99. [Crossref] [PubMed]
- Morita H, Suzuki G, Haddad W, et al. Cardiac contractility modulation with nonexcitatory electric signals improves left ventricular function in dogs with chronic heart failure. J Card Fail 2003;9:69-75. [Crossref] [PubMed]
- Neelagaru SB, Sanchez JE, Lau SK, et al. Nonexcitatory, cardiac contractility modulation electrical impulses: feasibility study for advanced heart failure in patients with normal QRS duration. Heart Rhythm 2006;3:1140-7. [Crossref] [PubMed]
- Muller D, Remppis A, Schauerte P, et al. Clinical effects of long-term cardiac contractility modulation (CCM) in subjects with heart failure caused by left ventricular systolic dysfunction. Clin Res Cardiol 2017;106:893-904. [Crossref] [PubMed]
- Kloppe A, Mijic D, Schiedat F, et al. A randomized comparison of 5 versus 12 hours per day of cardiac contractility modulation treatment for heart failure patients: A preliminary report. Cardiol J 2016;23:114-9. [Crossref] [PubMed]
- Chaanine AH, Nonnenmacher M, Kohlbrenner E, et al. Effect of bortezomib on the efficacy of AAV9.SERCA2a treatment to preserve cardiac function in a rat pressure-overload model of heart failure. Gene Ther 2014;21:379-86. [Crossref] [PubMed]
- Gorski PA, Ceholski DK, Hajjar RJ. Altered myocardial calcium cycling and energetics in heart failure--a rational approach for disease treatment. Cell Metab 2015;21:183-94. [Crossref] [PubMed]
- Bers DM. Calcium cycling and signaling in cardiac myocytes. Annu Rev Physiol 2008;70:23-49. [Crossref] [PubMed]
- Melman YF, Shah R, Danielson K, et al. Circulating MicroRNA-30d Is Associated With Response to Cardiac Resynchronization Therapy in Heart Failure and Regulates Cardiomyocyte Apoptosis: A Translational Pilot Study. Circulation 2015;131:2202-16. [Crossref] [PubMed]
- Wang R, Huang Q, Zhou R, et al. Sympathoexcitation in Rats with Chronic Heart Failure Depends on Homeobox D10 and MicroRNA-7b Inhibiting GABBR1 Translation in Paraventricular Nucleus. Circ Heart Fail 2016;9. [Crossref] [PubMed]
- Wahlquist C, Jeong D, Rojas-Munoz A, et al. Inhibition of miR-25 improves cardiac contractility in the failing heart. Nature 2014;508:531-5. [Crossref] [PubMed]
- Zhang F, Dang Y, Li Y, et al. Cardiac Contractility Modulation Attenuate Myocardial Fibrosis by Inhibiting TGF-beta1/Smad3 Signaling Pathway in a Rabbit Model of Chronic Heart Failure. Cell Physiol Biochem 2016;39:294-302. [Crossref] [PubMed]
- Ansalone G, Giannantoni P, Ricci R, et al. Doppler myocardial imaging to evaluate the effectiveness of pacing sites in patients receiving biventricular pacing. J Am Coll Cardiol 2002;39:489-99. [Crossref] [PubMed]
- Zolotukhin S, Byrne BJ, Mason E, et al. Recombinant adeno-associated virus purification using novel methods improves infectious titer and yield. Gene Ther 1999;6:973-85. [Crossref] [PubMed]
- He X, Zhang K, Gao X, et al. Rapid atrial pacing induces myocardial fibrosis by down-regulating Smad7 via microRNA-21 in rabbit. Heart Vessels 2016;31:1696-708. [Crossref] [PubMed]
- Livak KJ, Schmittgen TD. Analysis of relative gene expression data using real-time quantitative PCR and the 2(-Delta Delta C(T)) Method. Methods 2001;25:402-8. [Crossref] [PubMed]
- Pappone C, Augello G, Rosanio S, et al. First human chronic experience with cardiac contractility modulation by nonexcitatory electrical currents for treating systolic heart failure: mid-term safety and efficacy results from a multicenter study. J Cardiovasc Electrophysiol 2004;15:418-27. [Crossref] [PubMed]
- Lawo T, Borggrefe M, Butter C, et al. Electrical signals applied during the absolute refractory period: an investigational treatment for advanced heart failure in patients with normal QRS duration. J Am Coll Cardiol 2005;46:2229-36. [Crossref] [PubMed]
- Meyer M, Schillinger W, Pieske B, et al. Alterations of sarcoplasmic reticulum proteins in failing human dilated cardiomyopathy. Circulation 1995;92:778-84. [Crossref] [PubMed]
- Behar JM, Jackson T, Hyde E, et al. Optimized Left Ventricular Endocardial Stimulation Is Superior to Optimized Epicardial Stimulation in Ischemic Patients With Poor Response to Cardiac Resynchronization Therapy: A Combined Magnetic Resonance Imaging, Electroanatomic Contact Mapping, and Hemodynamic Study to Target Endocardial Lead Placement. JACC Clin Electrophysiol 2016;2:799-809. [Crossref] [PubMed]
- Andersson KB, Birkeland JA, Finsen AV, et al. Moderate heart dysfunction in mice with inducible cardiomyocyte-specific excision of the Serca2 gene. J Mol Cell Cardiol 2009;47:180-7. [Crossref] [PubMed]
- Davia K, Davies CH, Harding SE. Effects of inhibition of sarcoplasmic reticulum calcium uptake on contraction in myocytes isolated from failing human ventricle. Cardiovasc Res 1997;33:88-97. [Crossref] [PubMed]
- Hayward C, Banner NR, Morley-Smith A, et al. The Current and Future Landscape of SERCA Gene Therapy for Heart Failure: A Clinical Perspective. Hum Gene Ther 2015;26:293-304. [Crossref] [PubMed]
- Wu T, Chen W, Kong D, et al. miR-25 targets the modulator of apoptosis 1 gene in lung cancer. Carcinogenesis 2015;36:925-35. [Crossref] [PubMed]
- Huang F, Zhang L, Long Z, et al. miR-25 alleviates polyQ-mediated cytotoxicity by silencing ATXN3. FEBS Lett 2014;588:4791-8. [Crossref] [PubMed]
- Muto C, Solimene F, Gallo P, et al. A randomized study of cardiac resynchronization therapy defibrillator versus dual-chamber implantable cardioverter-defibrillator in ischemic cardiomyopathy with narrow QRS: the NARROW-CRT study. Circ Arrhythm Electrophysiol 2013;6:538-45. [Crossref] [PubMed]