Late clinical outcomes after mechanical aortic valve replacement for aortic stenosis: old versus new prostheses
Introduction
Although mechanical prostheses are still the main valve substitutes for younger patients, risks of thromboembolism and bleeding complications associated with life-long anticoagulation are major concerns (1,2). However, low-dose anticoagulation has been suggested to improve the outcomes of mechanical AVR (3). Lowering the target international normalized ratio (INR) and self-INR management could reduce hemorrhagic events (4,5). Additionally, there have been contradictory results regarding AVR with mechanical prostheses compared with bioprostheses according to target INR in the middle aged-group (1,2,6).
Several new-generation mechanical prostheses have been released that yielded better hemodynamic performance than the earlier generations (7-9). However, little has been reported about comparative long-term results or effects of anticoagulation between old and new mechanical prostheses in the aortic position. This study aimed to evaluate the clinical and hemodynamic outcomes of old- and new-generation mechanical prostheses after AVR for aortic stenosis (AS).
Methods
Study population
We retrospectively reviewed data from 835 patients who underwent primary AVR for severe AS at our hospital from January 1995 to December 2013. Eighty-three patients who had main coronary artery disease with AS, rheumatic AS, or history of previous cardiac surgery were excluded. Patients with coronary artery disease incidentally found on preoperative angiographs were included. Among the remaining 752 patients, 494 who underwent AVR with bioprosthesis were excluded. Four patients who were >70 years old with mechanical valves were also excluded. The remaining 254 patients were then classified into two groups: old-valve group (n=65), which received an old-generation mechanical prosthesis (ATS standard or Medtronic-Hall even-sized valves), and new-valve group (n=189), which received a new-generation mechanical prosthesis (St. Jude Regent, On-X, or Sorin Overline valves). The median patient age was 58 years (Q1–Q3: 52–61). To balance the distribution of baseline risk factors between the old-valve and new-valve groups, propensity score matching was performed. Fifty-six patients from the old-valve group were matched with 177 patients from the new-valve group. The study protocol was approved by the hospital’s institutional review board, which waived the requirement for patient consent (IRB no. 2016-10-118).
Surgical procedures
The basic surgical techniques and strategies for AVR have been described previously and were maintained throughout the present study (10,11). Old-type valves were used before 2002. New-type valves were used after 2002 and were adopted because of their better hemodynamic performance than similarly sized old-type valves. In the presence of small aortic annulus, root widening was performed in five patients [3 (5.4%) in the old-valve group, 2 (1.1%) in the new-valve group, P=0.091]. All patients received warfarin starting on postoperative day 1. The target INR ranged from 2.0 to 2.5 for old valves and from 1.8 to 2.3 for new valves. The target INR was increased according to the presence of risk factors of thromboembolism, such as atrial fibrillation or history of cerebral infarction.
Follow-up
Mortality and morbidity were defined according to standard guidelines (12). Early mortality was defined as death during hospitalization or within 30 days after surgery. Late mortality was defined as death occurring >30 days after the operation. Cardiac-related events included any structural or nonstructural prosthesis dysfunction, valve thrombosis, embolism, bleeding, prosthetic valve endocarditis, reoperation, or permanent pacemaker insertion. Anticoagulation-related events were defined as all events including valve thrombosis, embolism, and bleeding.
The median follow-up duration was 91 months (Q1–Q3: 48–138) for the entire study population: 179 months (Q1–Q3: 146–200) for the old-valve group and 70 months (Q1–Q3: 34–106) for the new-valve group (P<0.001). We reviewed the medical records for 71.7% of these patients who were followed by cardiac surgeons at our hospital. We obtained data for another 9.4% patients who were followed-up at other hospitals via direct telephone interviews with the patients or their families. The remaining 18.9% of patients were lost to follow-up; however, we confirmed their survival from the National Registry database.
Two-dimensional echocardiography with Doppler was performed before discharge and at 1, 3, 5, and >7 years after surgery. Echocardiographic assessments after discharge by our hospital’s cardiologist were obtained for 83.0% patients at year 1, 69.1% at year 3, and 62.9% at year 5.
Left ventricular ejection fractions were calculated with Simpson’s biplane method. Aortic transprosthetic mean pressure gradients (TMPGs, in mmHg) were calculated by using the Bernoulli equation. The left ventricular mass was calculated by using Devereux and Reichek’s formula (13). Left ventricular mass index (LVMI, in g/m2) was defined as the left ventricular mass/body surface area. Reference data for the prosthetic effective orifice area were obtained from the literature for each valve size. The indexed effective orifice area (EOAI) was defined as the effective orifice area/body surface area. Prosthesis-patient mismatch (PPM) was defined as EOAI ≤ was cm2/m2. Moderate and severe PPM were defined as EOAI 0.65–0.85 and <0.65 cm2/m2, respectively (14).
Statistical methods
Data are summarized as median (Q1–Q3) for continuous variables, or event number (%) for categorical variables. In preliminary analysis, the two groups were compared by using two-sample t-test (or Mann-Whitney U-test if skewed normally) for continuous variables and Pearson chi-square test (or Fisher’s exact test if sparse) for categorical variables. The primary outcomes for comparison were cardiac-related mortality, cardiac related events, and anticoagulation-related events. We used the propensity score method to balance the two groups in baseline and preoperative characteristics including age, sex, body surface area, hypertension, diabetes mellitus, stroke, New York Heart Association functional class, coronary artery disease, atrial fibrillation, anemia, chronic renal failure, infective endocarditis, chronic lung disease, and left ventricular ejection fraction, . We determined that balance was successfully achieved if the standardized mean difference was within 10% of each group and if the variance ratios were near 1.0. To prevent data loss during matching, we used the 1:m propensity matching method. We also considered the inverse probability of a treatment-weighted (IPTW) method as an added robust analysis result. Longitudinal changes in TMPG and LVMI were analyzed by using the generalized estimating equation method to adjust for serial correlations in data. Cox survival regression was used to obtain the hazard ratios (HRs) of late clinical outcomes between the two groups for the original crude matched data and for the inverse probability of treatment-weighted analyses. We used Schoenfeld residuals to confirm the assumptions of the Cox proportional hazard model. All statistical analyses were performed with R3.1.3 for Windows.
Results
Baseline characteristics and echocardiographic data
In baseline characteristics, the prevalence of New York Heart Association class III–IV and anemia (hemoglobin <12 g/dL) were higher, whereas left ventricular ejection fraction was lower in the old-valve group. The aortic valve area was smaller in the old-valve group. The prevalence of more-than-mild tricuspid regurgitation was higher in the old-valve group (Table S1). However, no differences in demographic data were observed between groups after propensity score matching (Table 1). In echocardiographic data, the left atrial diameter was greater in the new-valve group after matching. Other echocardiographic parameters did not differ significantly between groups.
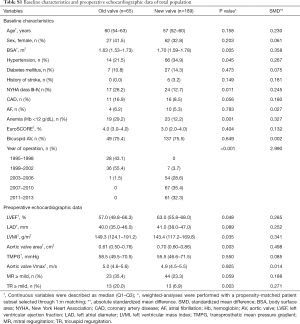
Full table
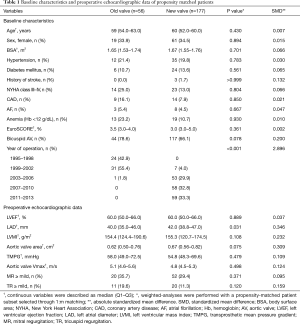
Full table
Operative data
The detailed valve types and sizes are shown in Table 2. The cardiopulmonary bypass time (P=0.814) and aortic cross-clamping time (P=0.686) did not differ significantly between groups. Concomitant surgical procedures and detailed operative data are described in Table 3. The number of concomitant root-widening procedures was not significantly different between groups; however, a higher trend was observed in the old-valve group (P=0.091).
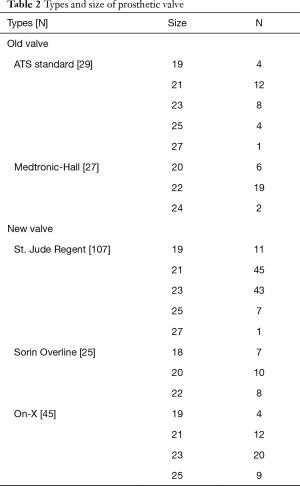
Full table
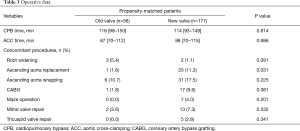
Full table
Clinical outcomes
There was no early mortality in either group. There were two bleeding episodes that required reoperation in the old-valve group; however, there were no bleeding complications in the new-valve group. There was one pacemaker insertion (1.8%) in the old-valve group and two pacemaker insertions (1.1%) in the new-valve group (Table 4).
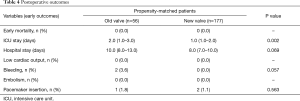
Full table
There were 28 late deaths in both groups combined. The causes of death included cerebral hemorrhage in three, cerebral infarction in two, alveolar hemorrhage in one, heart failure in one, ischemic cardiomyopathy in one, sudden death in one, cancer in 6, aging in one, and unknown in 10 patients. The 8-year overall survival did not differ significantly between groups [80.4% (old-valve group) vs. 93.1% (new-valve group), P=0.640; Figure 1]. However, freedom from cardiac-related mortality at 8 years was significantly higher in the new-valve group [90.6% (old-valve group) vs. 99.4% (new-valve group), P=0.047]. There were six reoperations. The causes of reoperation were infective endocarditis in three, pannus formation in two, and malfunction of mechanical valve in one patient.
Although cardiac-related 8-year events did not differ significantly between groups [79.5% (old-valve group) vs. 88.4% (new-valve group), P=0.161; Figure 1], anticoagulation-related 8-year events were significantly lower in the new-valve group [85.5% (old-valve group) vs. 94.3% (new-valve group), P=0.03]. Among anticoagulation-related events, bleeding events were significantly higher in the old-valve group [8.9% (old-valve group) vs. 1.1% (new-valve group), P=0.010]. Thromboembolic events did not differ between groups [7.1% (old-valve group) vs. 3.4% (new-valve group), P=0.258].
Table 5 summarizes the HRs between the two groups for clinical outcomes. Various statistical methods consistently indicated a lower cardiac-related mortality rate (0.121< HR <0.171) and anticoagulation-related events (0.229< HR <0.566) in the new-valve group than in the old-valve group. Matched analysis indicated that the new-valve group had lower hazards for developing cardiac-related mortality [HR =0.128, 95% confidence interval (CI), 0.017–0.980; P=0.048] and anticoagulation-related events (HR =0.259, 95% CI, 0.076–0.878; P=0.030).
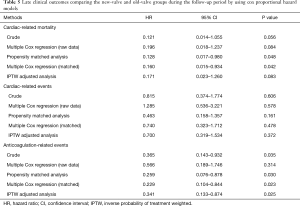
Full table
We compared the anticoagulation strategies between the groups because anticoagulation-related events, especially bleeding, were more common in the old-valve group. Follow-up INR was measured in all patients. INR was measured mean 32 time-points (47 time-points in the old valve group and 27 time points in the new valve group). The follow-up INRs were significantly higher in the old-valve group [2.23, Q1–Q3: 2.14–2.35 (old-valve group) vs. 2.08, Q1–Q3: 1.92–2.23 (new-valve group), P<0.001].
Hemodynamic and echocardiographic outcomes
The PPM incidence was significantly higher in the old-valve group (P<0.001). There were 12 (21.4) moderate PPMs in the old-valve group: 6 in ATS standard valve cases and 6 in the Medtronic-Hall valve cases. However, there were no moderate PPMs in the new-valve group. There were no severe PPMs in either group. In multivariable analysis of the total population (non-matched), moderate PPM was a significant risk factor for cardiac-related events (HR =5.279, P=0.002). Although it was not statistically significant, moderate PPM showed a trend toward a higher risk for overall mortality (HR =2.500, P=0.068) and cardiac-related mortality (HR =3.546, P=0.082). Moderate PPM showed a trend toward a higher risk for overall mortality in a matched data (HR =3.082, P=0.076)
Our subgroup analysis shows EOAI was significantly larger in new-valve group in both smaller and larger size of valves. However, the incidence of PPM was significantly lower in especially small size of New valves (Table 6).
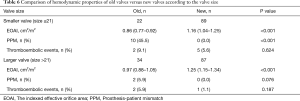
Full table
Figure 2 shows the serial changes in preoperative, postoperative (at discharge), and follow-up TMPG and LVMI for each group. TMPG was significantly higher in the old-valve group not only at discharge (P=0.034) but also during follow-up. Preoperatively, although LVMI tended to be higher in the old-valve group, it was not statistically significant (P=0.053). LVMI consistently decreased in both groups and did not differ significantly between groups during follow-up.
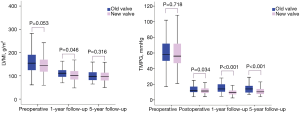
The incidence of tricuspid regurgitation progression was significantly higher in the old-valve group [10.2% (old-valve group) vs. 2.4% (new-valve group), P=0.041].
We analyzed hemorrhagic events, excluding patients with PPM, and found that they were significantly more common in the old-valve group (P=0.024).
Discussion
The current guidelines recommend a mechanical prosthesis for AVR in patients less than 50 to 60 years old, decreased from 60 to 65 years, considering their life expectancy and the structural valve deterioration of biological prostheses (15,16). This reflects recent trends in valve choice for AVR in middle-aged patients, and involves extending the age criteria for tissue-valve implantation and increased application of the Ross procedure owing to poor late outcomes after AVR with mechanical prostheses.
Several authors have reported favorable results from biological prostheses for AVR in middle-aged patients. McClure et al. described results from 361 propensity-matched patients aged <65 years with stented bioprostheses and mechanical prostheses; the overall survival did not differ between groups (P=0.75). Bleeding events were significantly more common in the mechanical prostheses group (P=0.002) (2). However, conventional INR management for which the target INR was 2.0–3.0, and even with aspirin, was used in this study.
Several recent reports have demonstrated that the Ross operation yields excellent results (17). However, the Ross operation for AVR is still not widely adopted because of its technical complexity and the possibility of complicated reoperations at the late follow-up stage.
In the last few years, TAVI has become a rapidly evolving therapeutic intervention that has been introduced for high-risk patients with conventional AVR (18). Additionally, valve-in-valve implantation procedures have been attempted for failed bioprosthetic valves; thus, TAVI may be an alternative treatment option for structural valve deterioration, avoiding repeat operations (19). However, serious complications such as valve malposition or migration, high transvalvular pressure gradients, coronary obstruction, and early structural valve failure can occur after transcatheter valve-in-valve implantation and lead to poor late outcomes (20).
The previous studies that compared mechanical and biological prostheses were concerned with anticoagulation-related complications of mechanical prostheses, primarily bleeding complications (1,2). Lowering the target INR and self-INR management have been previously suggested to improve the outcomes of mechanical prostheses by reducing anticoagulation-related events, especially bleeding complications (3-5). Furthermore, several studies with a lower target INR yielded better results for mechanical prostheses than bioprostheses. Stassano et al. presented results from a randomized study of 310 patients who underwent AVR (155 biological vs. 155 mechanical prostheses), in which the target INR was 2.0–2.5. Although the overall mortality (P=0.2) and cardiac-related mortality (P=0.12) did not statistically differ between groups, they tended to be lower for mechanical prostheses (1). Nishida et al. reported results from an age-matched analysis of mechanical prostheses and bioprostheses. The target INR was 1.8–2.4. The actual survival (P=0.458) and freedom from valve-related death (P=0.740) did not differ between groups for patients aged 60–69 years. However, actual survival (P=0.014) and freedom from valve-related death (P=0.004) were higher in patients with mechanical prostheses who were <60 years old (6). In our study, anticoagulation-related events and bleeding events were significantly more common in the old-valve group. The follow-up INR was significantly lower in the new-valve group [2.23, Q1–Q3: 2.1–2.35 (old-valve group) vs. 2.08, Q1–Q3: 1.92–2.23 (new-valve group), P<0.001]. Our results confirmed that the lower target INR of the new-valve group affected better clinical outcomes, especially in lowering anticoagulation-related events and bleeding events.
Although the current American Heart Association/American College of Cardiology guidelines suggest that the target INR should be 3.0 if combined with the presence of risk factors, or 2.5 with no risk factors (15), and the European Society of Cardiology (ESC) guidelines suggest that target INRs should range from 2.5 to 4.0 according to prosthesis thrombogenicity or patient-related risk factors (16), we argue for target INRs to be lowered to 1.8–2.3 (2.0) for Asian patients in whom bleeding events are relatively more common than in Western patients, owing to their different diets. We also suggest that the optimal target INR in Western patients should be lowered to 2.0–2.5. However, Puskas et al. showed that lower target INRs (1.5–2.0) yielded fewer bleeding events (P=0.002) than standard target INRs (2.0–3.0) in 375 patients who underwent AVR with On-X valves. However, although not statistically significant, thromboembolic events tended to be higher in the lower target INR group (P=0.128) in their study (3). Therefore, lowering the target INR to 1.5 should be deliberated.
The impact of PPM is still a controversial issue (21,22). However, several authors are concerned that PPM can yield worse outcomes after AVR. In our study, we used even-sized Medtronic-Hall valves (tilting disc valves). These valves have thinner sewing cuff, and their effective orifice area was one size larger than that of the standard Medtronic-Hall valve. Even-sized Medtronic-Hall valves have shown better hemodynamic performance than standard ATS valves (23). Furthermore, only standard ATS valves were used in our study. AP (advanced-performance)-ATS valves which has better hemodynamics was not available at that time in our hospital. Meanwhile, several new-generation mechanical prostheses have been released that have been reported to offer better hemodynamic performance and lower TMPGs despite implanting small-sized prostheses, because of their advanced design and supra-annular position (7-9). Especially, the Sorin Overline valve was designed for a totally supra-annular position, which can provide an advantage of one size over the intra-annular valves in patients with AS, and has shown excellent hemodynamic performance (7). In our study, most AVRs were performed with the supra-annular technique. Therefore, although the incidence of root widening procedure was low, the follow-up TMPG was within an acceptable range (Figure 2). The newest type of mechanical prostheses also offered better hemodynamic performance for late TMPG and lower PPM incidence, consistent with previous studies. Although the follow-up LVMI did not differ between the two groups, moderate PPM was a significant risk factor for cardiac-related events (HR =5.279, P=0.002) and was associated with higher risks for overall (HR =2.500, P=0.068) and cardiac-related (HR =3.546, P=0.082) mortality. The results of our study may indicate that better hemodynamic performance improves long-term clinical outcomes. Our subgroup analysis showed these hemodynamic improvements of new valves seemed to be more prominent in small size of valves (Table 6).
This study has several limitations. First, because it was a retrospective study, it may have been influenced by a selection bias Therefore, we performed propensity matching to compensate for the potential bias in patient selection. However, an unidentified confounding bias may influence the results. In addition, the loss of significant number of patients in the old-valve group was inevitable in matching patients. However, we additionally analyzed based on IPTW method using propensity score. We also analyzed crude and multiple cox regression analysis and observed consistent results, as reported. Second, target INR was determined by surgeon’s preference. We applied different target INRs to the new-valve group because of different time periods. We maintained INR at the 2.0–2.5 range for the old-valve group and lowered the target INR to 1.8–2.3 for the new-valve group. Therefore, further comparisons between conventional INR and lower INR strategies for new-generation valves are required. Third, we included several different valve types in our study because of the long study duration. In our study, 27 Medtronic-Hall valves (even-sized) were included. Although the Medtronic-Hall valve was of a different type (monoleaflet valve) from the other valves, we did not have concerns about different anticoagulation strategies. According to the ESC guidelines, the Medtronic-Hall valve was categorized as low thrombogenic prosthesis, like the St. Jude Medical and On-X valves (16). We analyzed the PPM by using a reference EOAI because the effective orifice area at the 1-year follow-up point has been only recently measured at our institution. Lastly, our sample size was small, and the follow-up duration was limited. The follow-up duration for the new-valve group was shorter than that for the old-valve group because we started to use new valves in 2003 and new valves have been subsequently replace the old valves. In addition, although the patients were completely followed up through their survival, clinical follow-up data was not available in all patients (81.1%). A larger and longer follow-up study is needed to confirm our findings. Finally, follow-up echocardiographic data was not available in all patients; thus it is possible that sampling bias may have affected long-term hemodynamic parameters.
Conclusions
In conclusion, new mechanical valves in the aortic position significantly improved late outcomes compared with old types of mechanical valves. New mechanical valves showed better hemodynamic performance and lower incidence of PPM. A lower target INR (1.8–2.3) after AVR with new mechanical valves would decrease the incidence of bleeding complications. These findings should be considered for selecting prosthetic valve in middle-aged patients with AS. Further study is necessary to evaluate the outcomes of new mechanical prostheses with anticoagulation strategy to lower the target INR compared with Ross operation or bioprostheses in middle-aged patients.
Acknowledgements
None.
Footnote
Conflicts of Interest: The authors have no conflicts of interest to declare.
Ethical Statement: The study protocol was approved by the hospital’s institutional review board, which waived the requirement for patient consent (IRB no. 2016-10-118).
References
- Stassano P, Di Tommaso L, Monaco M, et al. Aortic valve replacement: a prospective randomized evaluation of mechanical versus biological valves in patients ages 55 to 70 years. J Am Coll Cardiol 2009;54:1862-8. [Crossref] [PubMed]
- McClure RS, McGurk S, Cevasco M, et al. Late outcomes comparison of nonelderly patients with stented bioprosthetic and mechanical valves in the aortic position: a propensity-matched analysis. J Thorac Cardiovasc Surg 2014;148:1931-9. [Crossref] [PubMed]
- Puskas J, Gerdisch M, Nichols D, et al. Reduced anticoagulation after mechanical aortic valve replacement: interim results from the prospective randomized on-X valve anticoagulation clinical trial randomized Food and Drug Administration investigational device exemption trial. J Thorac Cardiovasc Surg 2014;147:1202-10; discussion 1210-1. [Crossref] [PubMed]
- Koertke H, Zittermann A, Wagner O, et al. Efficacy and safety of very low-dose self-management of oral anticoagulation in patients with mechanical heart valve replacement. Ann Thorac Surg 2010;90:1487-93. [Crossref] [PubMed]
- Badhwar V, Ofenloch JC, Rovin JD, et al. Noninferiority of closely monitored mechanical valves to bioprostheses overshadowed by early mortality benefit in younger patients. Ann Thorac Surg 2012;93:748-53. [Crossref] [PubMed]
- Nishida T, Sonoda H, Oishi Y, et al. Long-term results of aortic valve replacement with mechanical prosthesis or carpentier-edwards perimount bioprosthesis in Japanese patients according to age. Circ J 2014;78:2688-95. [Crossref] [PubMed]
- Reyes G, Badia S, Alvarez P, et al. Results of aortic valve replacement with the supra-annular Sorin Bicarbon Overline prosthesis. J Heart Valve Dis 2012;21:358-63. [PubMed]
- Mizoguchi H, Sakaki M, Inoue K, et al. Mid-term results of small-sized St. Jude Medical Regent prosthetic valves (21 mm or less) for small aortic annulus. Heart Vessels 2013;28:769-74. [Crossref] [PubMed]
- Chambers JB, Pomar JL, Mestres CA, et al. Clinical event rates with the On-X bileaflet mechanical heart valve: a multicenter experience with follow-up to 12 years. J Thorac Cardiovasc Surg 2013;145:420-4. [Crossref] [PubMed]
- Jeong DS, Sung K, Kim WS, et al. Fate of functional tricuspid regurgitation in aortic stenosis after aortic valve replacement. J Thorac Cardiovasc Surg 2014;148:1328-33 e1.
- You JH, Jeong DS, Sung K, et al. Aortic Valve Replacement With Carpentier-Edwards: Hemodynamic Outcomes for the 19-mm Valve. Ann Thorac Surg 2016;101:2209-16. [Crossref] [PubMed]
- Akins CW, Miller DC, Turina MI, et al. Guidelines for reporting mortality and morbidity after cardiac valve interventions. J Thorac Cardiovasc Surg 2008;135:732-8. [Crossref] [PubMed]
- Devereux RB, Reichek N. Echocardiographic determination of left ventricular mass in man. Anatomic validation of the method. Circulation 1977;55:613-8. [Crossref] [PubMed]
- Pibarot P, Dumesnil JG. Prosthesis-patient mismatch: definition, clinical impact, and prevention. Heart 2006;92:1022-9. [Crossref] [PubMed]
- Nishimura RA, Otto CM, Bonow RO, et al. 2017 AHA/ACC Focused Update of the 2014 AHA/ACC Guideline for the Management of Patients With Valvular Heart Disease: A Report of the American College of Cardiology/American Heart Association Task Force on Clinical Practice Guidelines. J Am Coll Cardiol 2017;70:252-89. [Crossref] [PubMed]
- Baumgartner H, Falk V, Bax JJ, et al. 2017 ESC/EACTS Guidelines for the management of valvular heart disease. Eur Heart J 2017;38:2739-91. [Crossref] [PubMed]
- Karaskov A, Sharifulin R, Zheleznev S, et al. Results of the Ross procedure in adults: a single-centre experience of 741 operations. Eur J Cardiothorac Surg 2016;49:e97-e104. [Crossref] [PubMed]
- Leon MB, Smith CR, Mack M, et al. Transcatheter aortic-valve implantation for aortic stenosis in patients who cannot undergo surgery. N Engl J Med 2010;363:1597-607. [Crossref] [PubMed]
- Dvir D, Webb JG, Bleiziffer S, et al. Transcatheter aortic valve implantation in failed bioprosthetic surgical valves. JAMA 2014;312:162-70. [Crossref] [PubMed]
- Dvir D, Webb J, Brecker S, et al. Transcatheter aortic valve replacement for degenerative bioprosthetic surgical valves: results from the global valve-in-valve registry. Circulation 2012;126:2335-44. [Crossref] [PubMed]
- Medalion B, Blackstone EH, Lytle BW, et al. Aortic valve replacement: is valve size important? J Thorac Cardiovasc Surg 2000;119:963-74. [Crossref] [PubMed]
- Howell NJ, Keogh BE, Barnet V, et al. Patient-prosthesis mismatch does not affect survival following aortic valve replacement. Eur J Cardiothorac Surg 2006;30:10-4. [Crossref] [PubMed]
- Sung K, Park PW, Park KH, et al. Comparison of transprosthetic mean pressure gradients between Medtronic Hall and ATS valves in the aortic position. Int J Cardiol 2005;99:29-35. [Crossref] [PubMed]