Hydrogen gas inhalation protects against cigarette smoke-induced COPD development in mice
Introduction
Chronic obstructive pulmonary disease (COPD) is the fourth-leading cause of death in the world (1), imposing a substantial economic and social burden (2). Cigarette smoking is the most common risk factor for COPD. A strong relationship has been identified between continuous tobacco use, oxidative stress, and exacerbation of COPD symptoms (3). Cigarette smokers have a higher prevalence of respiratory symptoms, lung function abnormalities, and COPD mortality rate than non-smokers (4). Currently, COPD drug therapy is used to relieve symptoms, improve athletic ability and health status as well as reduce the frequency and severity of exacerbation. However, there is no known pharmaceutical therapy for COPD that is able to reverse lung function decline (5-8). Therefore, it is necessary to develop new and effective medicine to prevent or treat COPD with fewer side-effects.
It has been shown that H2, a physiologically regulatory gas molecule, exerts anti-inflammatory (9), antioxidant (10), anti-apoptotic (11) and signalling-regulating effects (12). Hydrogen gas is able to react with hydroxyl radicals (·OH) directly, which may contribute to its anti-inflammatory and anti-oxidative lesions. Clinical trials have confirmed that H2 can be used to treat cerebral ischaemia, uraemia (13), diabetes (14), metabolic syndrome (15), erythema skin disease, pressure ulcers, malignancies, rheumatism, arthritis (16,17), side-effects associated with tumor radiotherapy and chemotherapy (18), mitochondrial muscle disease, and Parkinson's disease (19). Furthermore, inhalation of H2 or ingestion of H2-rich water can effectively prevent acute lung injury in mice induced by oxygen poisoning, ventilator injury, ischaemia-reperfusion injury, or sepsis (20,21). H2-rich saline also attenuates cigarette smoke (CS)-induced airway mucus production in rats (22). Xiao et al. reported that H2 saturated water reduced airway remodelling and airway mucus hypersecretion by reducing NF-κB activation in asthmatic mice (23). H2 inhalation attenuated lung inflammatory responses in CS-induced COPD in rats. Furthermore, higher concentrations of H2 showed better outcome compared to lower concentrations of H2 (24). These findings strongly indicate that H2 treatment may be beneficial in treating COPD. In the present study, we employed mouse model of COPD with CS exposures, and evaluated the effects of hydrogen gas on the development of COPD. We also examined the effects of hydrogen gas exposure on the activation of ERK1/2 and NF-κB-dependent inflammatory responses induced by hydrogen peroxide (H2O2) in human bronchial epithelial cells.
Methods
Reagents
All cell culture reagents were purchased from Gibco (Carlsbad, CA, USA). Masson’s trichrome stain kit (HT15), Cell Counting Kit-8 [96992], and H2O2 solution (7722-84-1) were obtained from Sigma-Aldrich (St Louis, MO, USA). Periodic acid-Schiff (PAS) kit was purchased from Shanghai Sun Biotechnology Co., Ltd (Shanghai, China). β-actin antibody (sc-47778) was obtained from Santa Cruz Biotechnology (Dallas, TX, USA). NF-κB (ab32536) antibody was obtained from Abcam (Cambridge, UK). Antibodies against ERK1/2 (#4695), phosphorylated ERK1/2 (#8544), and histone H3 (#9728) were obtained from Cell Signaling Technology (Beverly, MA, USA).
Animals and CS exposure
Wild type C57BL/6J male mice (6–8 weeks old) were purchased from the Nanjing BioMedical Research Institute of Nanjing University (Nanjing, China). Animals were housed in the specific pathogen-free facilities and the Animal Care and Use Committee of Guangzhou Medical University approved all experimental protocols. And all methods were performed in accordance with the guidelines and regulations approved by the Animal Care and Use Committee of Guangzhou Medical University. Animals were randomized into the following three groups: control group (CTL, normal air inhalation without CS exposure), CS exposure (CS), CS exposure and H2 inhalation (CS + H2). To establish the COPD mouse model, mice were exposed to CS (9 cigarettes/h, 2 h per exposure, twice per day, 6 days per week) in a whole-body exposure chamber for 90 days, as we previously described (25,26). The cigarettes used in this study were the Plum brand, produced by Guangdong Tobacco Industry Co., Ltd. (Guangzhou, China). Each cigarette yields 11 mg tar, 1.0 mg nicotine, and 13 mg carbon monoxide (CO). For H2 treatment, after exposure to CS for 60 days, mice were treated with H2. H2 and oxygen (O2) were generated by electrolysing deionised water with a H2 apparatus (Shanghai Asclepius Meditech, Shanghai, China). Fresh mixture of H2 (66.7%) and O2 (33.3%) gases was diluted with nitrogen (N2) separated from air to a mixture containing the H2 (42%), O2 (21%) and N2 (37%) and delivered through a rubber tube at a flow of 3.8 L/min to the CS-exposed mice put in a sealed chamber connecting to the outside air through a hole. The H2 inhalation was performed for one hour per session, twice per day with interval of 6 to 8 hours. The mice in control group were put in the sealed chamber and given air. The concentrations of H2, O2 and CO2 in the chamber were monitored at the beginning and by the end of each inhalation to ensure the stability of each air component. The animals were then subjected to lung function assessment before dissection for further analysis on day 91.
Lung function measurement
Lung function was measured using a Forced Pulmonary Maneuver System (Buxco Research Systems, Wilmington, NC, USA) following the manufacturer’s protocol, as we previously described (25).
Hematocrit measurement
At the end of the chronic CS exposure, hematocrit was measured according to the method as we previously described (25).
Bronchoalveolar lavage fluid (BALF) collection and cell counting
Every mouse lung was lavaged with 0.6 mL of saline three times. Total cells in BALF were collected and counted using a haemocytometer. The cells were then subjected to giemsa staining for differential counting of neutrophils, macrophages, and lymphocytes under a microscope.
Histological staining and morphological analysis
After sacrifice, the left lungs of mice were fixed in 10% neutral buffered formalin for 24 h and then dehydrated and embedded in paraffin. The samples were cut into 4-µm sections and stained with H&E for histological examination. Lung sections were stained with PAS to determine goblet cell density or Masson’s trichrome stain for collagen deposition with commercial kits following the manufacturers’ instructions. Alveolar enlargement and destruction were determined by the average linear intercept as previously described (27). Image-Pro Plus version 6.0 software (Media Cybernetics, Rockville, MD, USA) was used to assess the mean linear intercept, reflecting the ratio of total length of alveoli to the number of alveoli per field under light microscopy. Similarly, to quantify collagen deposition in the small airway according to a previously described protocol (28), we used images of all airways with a diameter of 50–499 µm in lungs, including the blue layer outside the airway, which is the layer of deposited collagen, because larger airways are not associated with increased collagen deposition in CS-exposed mice. The area of the collagen layer deposited around small airways was calculated as follows: collagen thickness = collagen area/total bronchial area. Semi-quantitative analyses of the area stained positive area for goblet cells in the airway epithelium were defined using Image-Pro Plus. At least three fields per mouse were used.
Cell culture and treatment
16HBE were purchased from the Cell Bank of the Chinese Academy of Science (Shanghai, China) and cultured in DMEM medium containing 10% FBS, 100 U/mL penicillin and 100 µg/mL streptomycin in a humidified incubator at 37 °C with 95% (v/v) air and 5% (v/v) CO2. For treatment with H2O2 plus H2, 16HBE cells were seeded in 6-well plates at 60~70% confluence, then, the cells were serum starved for 12 h, and exposed to H2 (42%) for 1 h prior to 24 h of treatment with H2O2 (0.1 mM) in 2 mL medium containing 1% FBS. After all these treatments, cells were washed twice with PBS, cell lysates were harvested for Western blot analysis. The supernatant was stored at −80 °C for detecting the pro-inflammatory cytokines.
Cell viability assay
The cell viability of 16HBE cells upon H2O2 treatment was determined by using a CCK8 assay kit (Sigma, USA). The cells (5,000 cells/well) were seeded in 96-well plates, which were treated with H2O2 (0–0.8 mM in medium containing 1% FBS) for 24 h. Subsequently, 10 µL of CCK8 solution was added to each well for an additional 2 h. Finally, we recorded the absorbance at 450 nm using a microplate absorbance reader (Thermo Scientific, MA, USA). Cell viability was presented as percentage of cells without H2O2 treatment.
Preparation of nuclear protein extracts
Nuclear proteins from lung tissues or 16HBE cells were extracted using the NE-PER Nuclear and Cytoplasmic Reagents (Thermo Fisher Scientific) containing a protease inhibitor cocktail according to the manufacturer’s instructions. Histone H3 served as the loading control in Western blotting of nuclear proteins.
Western blotting
Mouse lung tissues and cells were homogenized in RIPA lysis buffer containing 1% protease inhibitor cocktail (Sigma-Aldrich), 5 µM EDTA, and 200 µM 4-(2-aminoethyl) benzenesulfonyl fluoride hydrochloride to extract the total protein. Equal amounts of protein in homogenate samples were separated in SDS-PAGE (Bio-Rad Laboratories, Hercules, CA, USA) and blotted with primary antibodies and corresponding peroxidase-conjugated secondary antibodies. The bound antibody signal was developed using an Immun-Star HRP chemiluminescent kit (Bio-Rad Laboratories). Western blot image was obtained by Tanon 5200 Chemiluminescence Imaging System (Shanghai Tanon Science & Technology, Shanghai, China). Semi-quantitative analyses of immunoblots were performed using the Image J.
Enzyme-linked immunosorbent assay (ELISA)
Levels of interleukin (IL)-6,keratinocyte chemoattractant (KC), tumour necrosis factor-α (TNF-α), IL-8, Muc5ac and Muc5b in BALF or in cell culture medium were measured by ELISA. Briefly, human or mouse IL-6 (88-7066/88-7064), mouse TNF-α (88-7324), and human IL-8 (88-8086) were measured using the ELISA kits from eBioscience (San Diego, CA, USA) following the manufacturer’s protocols. KC in BALF was detected with ELISA using capture and detection antibodies obtained from R&D Systems (Minneapolis, MN, USA) according to a protocol described previously (29). Muc5ac and Muc5b in BALF was also detected as described previously (30). The capture antibodies for Muc5ac (sc-21701) and Muc5b (sc-135508) were obtained from Santa Cruz Biotechnology (Santa Cruz, CA), and the corresponding detection antibody was obtained from KPL, Inc. (Gaithersburg, MD, USA). The signal was developed using a TMB Substrate Reagent Set (BD Biosciences, Franklin Lakes, NJ, USA).
Statistical analysis
Data were processed with Graphpad Prism 5 software and presented as mean ± SEM. In the animal experiment, “n” represents animal number, while in the cell experiment, “n” represents the number of experiments repeated. The significance of difference among groups was evaluated by One-way ANOVA and Bonferroni-Holms test. P<0.05 was considered statistically significant.
Results
H2 inhalation attenuates CS-induced lung function decline in mice
COPD mice were established by CS exposure for 90 days (Figure 1A). Compared with control mice with normal air inhalation, the CS exposed mice presented typical COPD-like lung function decline (Figure 1B,C,D,E,F,G) indicated by increases in FRC, total lung capacity (TLC), Chord compliance (Cchord), FVC, and resistance (RI), as well as a decrease in the FEV50/FVC ratio. Although difference of FVC and RI between CS group and CS+H2 group is not significant, CS-caused increases in FRC, TLC, Cchord and decrease in the FEV50/FVC were attenuated by H2 inhalation. As shown in Figure 1H, CS exposures significantly increased hematocrit value in blood, which was ameliorated by H2 administration. Altogether, these results demonstrate that H2 inhalation ameliorates CS-induced mouse lung function decline and hypoxia-induced hematocrit elevation.
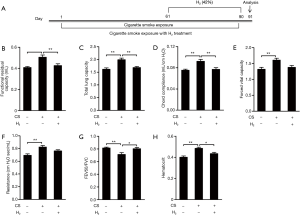
H2 inhalation attenuates CS-induced emphysema, collagen deposition in the small airway and goblet cell hypertrophy and hyperplasia of airway epithelium
Figure 2A showed a typical pathological presentation of COPD, such as damaged alveolar walls and pulmonary bullae, in mouse lungs exposed to CS. Inhalation of H2 significantly reduced structural damage of the lung and accumulation of leukocytes in both the alveolar walls and spaces. Goblet cells from the airway epithelium of CS-exposed mice, identified by PAS staining, contained large granular stores of PAS-positive substances, which was attenuated in the H2 treatment group (Figure 2B). Blue staining displayed severe collagen deposition in the small airway (50–499 µm diameter) in CS-exposed mice, and these effects were reduced by H2inhalation (Figure 2C). These results suggest that H2 inhalation ameliorates CS-induced COPD pathological changes in mouse lung.
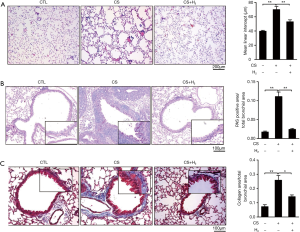
H2 inhalation reduces CS-induced airway inflammation and mucus hypersecretion in COPD mice
Figure 3A,B,C,D showed that CS exposure resulted in airway and lung inflammation, indicated by increases in total leukocyte number (i.e., neutrophils, macrophages, and lymphocytes) and higher levels of IL-6, TNF-α, and KC in BALF. H2 treatment attenuated these effects. Levels of Muc5ac and Muc5b were increased significantly in BALF from the CS-exposed group compared to control mice, which was reduced significantly by H2 treatment (Figure 3E,F). These results demonstrate that H2 inhalation attenuates CS-induced airway inflammation and mucus hypersecretion in COPD mice.
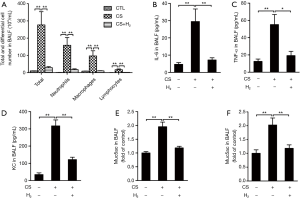
H2 decreases H2O2-induced pro-inflammatory cytokine release in human bronchial epithelial cells
Under the exposure of 42% H2, concentration of H2 in cell culture medium gradually increased with the exposure time, and H2 concentration in cell culture medium was saturated at 60 min (445±36 ppb) (Figure 4A). H2O2, an important factor inducing oxidative stress, is elevated in expired breath condensate of COPD patients compared with control subjects (31), we therefore we investigated the anti-inflammatory and antioxidant abilities of H2 against H2O2-induced oxidative injury in human bronchial epithelial cells (16HBE). After incubating the cells with H2O2 for 24 h at concentrations of 0.1, 0.2, 0.4, and 0.8 mM, we observed a concentration-dependent decrease in cell viability (Figure 4B). While high concentrations of H2O2 (0.2–0.8 mM) decreased cell viability, lower concentration of H2O2 (0.1 mM) did not reduce cell viability, but enhanced the release of IL-6 and IL-8 to culture supernatant (Figure 4C,D). However, lower levels of IL-6 and IL-8 were observed in the supernatant from 16HBE cells pre-treated with H2, compared with levels in the H2O2 group. These findings suggest that H2 treatment prevented H2O2-induced pro-inflammatory cytokine release from 16HBE cells.
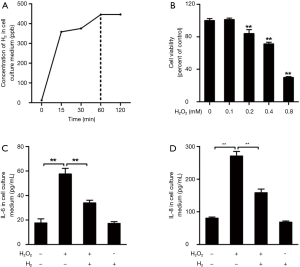
H2 inhalation reduces activation of ERK1/2 and NF-kB in CS-exposed mouse lungs and H2O2-treated 16HBE cells
Oxidative stress and inflammation play a key role in the pathogenesis of COPD. MAPKs and NF-κB signal pathways play important roles in regulating CS-induced the secretion of pro-inflammatory mediators (32-34), To further elucidate how H2 modulates CS-induced inflammation, we measured the action of ERK1/2 and NF-κB in lung tissue. As shown in Figure 5A, the phosphorylation of ERK1/2 and intranuclear accumulation of NF-κB were remarkably increased in lung tissue from the CS group. H2 treatment significantly reduced these levels. In 16HBE cells, we observed that ERK1/2 phosphorylation and nuclear accumulation of NF-κB were significantly increased when treated with H2O2, while pretreatment with H2 attenuated these molecular changes (Figure 5B). Altogether, these results suggest that H2 protects against CS and oxidative stress-induced activation of NF-κB and MAPK signal pathways.
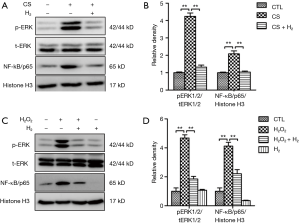
Discussion
COPD is a leading cause of morbidity and mortality worldwide (1,35). The major risk factor for COPD is tobacco smoke, including exposure to second-hand smoke (36). In the present study, we showed that inhalation of high-concentration hydrogen gas ameliorated lung function decline and COPD histopathological changes caused by CS exposure, including pulmonary emphysema, chronic bronchitis, and small airway remodelling. These histopathological changes lead to gas trapping and progressive airflow limitation. The protective role of H2 in CS-exposed mouse lungs is attributable at least in part to its anti-inflammatory and anti-oxidant activities. CS-induced phosphorylation of ERK1/2 and nuclear accumulation of NF-κB in lungs were abolished by H2 treatment. Furthermore, H2 protection against H2O2-induced ERK1/2 and NF-κB-dependent inflammatory responses in 16HBE cells. Based on our findings, H2 is a potential drug for COPD prevention, which could be conveniently delivered by inhalation.
In this study, the mouse model was established by CS exposure, a method that mimics the development of human COPD. Inflammation induced by CS is a critical factor in disease development. CS inhalation elicits acute and chronic inflammatory responses, which can potentially cause alveolar destruction. Once lung tissue damage exists and patients has difficulty in breathing. Smoking cessation may not significantly prevent further lung damage (37). The mechanisms for this amplified inflammation are not yet fully understood. Oxidative stress in the lung is likely to further trigger inflammation in COPD (37-39). Even with the present anti-inflammatory medications, we find it difficult to control airway inflammation Unlike the long-acting bronchodilators, it is not easy to find safe and effective anti-inflammatory treatment of COPD (40). So far, there is no treatments to effectively inhibit chronic inflammation in COPD.
Recent several studies have indicated that H2 is a new type of medical treatment gas with anti-oxidant and anti-inflammatory properties by alleviating the secretion of inflammatory cytokines, such as IL-6 and TNF-a and some phosphorylating signal factors (41,42). Adding H2 to haemodialysis solutions ameliorated inflammatory reactions by reducing the levels of MCP-1 and myeloperoxidase in plasma of haemodialysis patients (13), which brings the potential therapeutic effect to prevent lung diseases.
Our findings demonstrated that inhalation of 42% H2 ameliorated COPD histopathological changes and improved lung function in CS-induced mouse COPD model. COPD is an inflammatory disease of respiratory system, and oxidative stress plays an important role in the process (39). The beneficial effects of inhaled H2 are undoubtedly due, in part, to its antioxidant property (9). However, information on H2 regulation in vivo is limited, and the signalling molecules involved require further investigation. Several studies have shown that NF-κB plays a pivotal role in COPD inflammation (37). Moreover, ERK1/2 has been reported to be involved in CS-induced inflammation by modulating NF-κB DNA binding activity in A549 cells (43). In the present study, we found that 16HBE cells pretreated with H2 before exposure to H2O2 released lower levels of IL-6 and IL-8 and displayed reduced activation ofERK1/2 and NF-κB, suggesting that H2 attenuated H2O2-induced inflammation. In vivo, CS-induced ERK1/2 phosphorylation and NF-κB nuclear translocation were abolished by H2 treatment in mouse lung. Therefore, H2 inhalation attenuates CS-induced oxidative stress, leading to reduced inflammatory responses and subsequent emphysema.
Pulmonary function analysis is an important tool in the evaluation of mouse respiratory disease models. However, most commonly used pulmonary function variables of human are not routinely applied in mice. An invasive pulmonary function device (forced maneuvers system from Buxco Research Systems) was used to evaluate the mouse model of CS-induced COPD. CS exposure for 3 months caused airspace enlargement in mice. Similarly, statistically significant increases in FRC, Cchord, FVC, TLC and RI, as well as a decrease in the FEV50/FVC ratio were obtained in CS-exposed mice, which consisted with our previous studies (25,26). Furthermore, the CS-induced lung function decline in mice was attenuated by H2 inhalation. From a clinical perspective, FVC is usually reduced in actual cases of CS-induced COPD. So it was remarkable that FVC was increased in CS-exposed mice. The mechanism for this is as follows. First, human lung function is measured during active respiration, however, mouse lung function was measured during forced passive respiration. Second, a statistically significant increase in TLC was obtained in CS-exposed mice when compared with the control group, which is similar in humans. Third, due to the larger lung volume upon maximal inflation in CS-induced mice, forced expiration maneuver resulted in significant increase of FVC, whereas FEV50/FVC (parameter for airflow obstruction) during expiration was significantly reduced.
Mucus hypersecretion is one of the most important factors in predicting the morbidity and mortality in COPD (44). In the present study, we demonstrated that H2 inhalation significantly ameliorated CS-induced airway mucus production, which were identified by decreased hyperplasia of airway goblet cells and reduced levels of Muc5ac and Muc5b in BALF. This protective effect of H2 on CS-induced mucus hypersecretion was, at least partially, due to its antioxidant ability (22).
Compared with other anti-inflammatory drugs for treating COPD, H2 offers several advantages. First, it is nontoxic and reacts with the hydroxyl radical (·OH) to produce water in the body. Second, H2 can easily penetrate membranes and diffuse into the cytosol and nucleus, making it highly effective in reducing cytotoxic substances (45). Third, there is no influence of H2 on the physiologic properties (24,46). Fourth, H2 treatment is simple and inexpensive. Altogether, inhaled H2 is a promising, easily delivered and straightforward therapeutic option for COPD.
In conclusion, high-concentration H2 inhalation abrogated CS-induced pulmonary emphysema, chronic bronchitis, and small airway remodelling in mice, as well as reduced lung function decline. This protective action is attributable to the anti-inflammatory and anti-oxidant actions of H2. The inhibition of the ERK1/2 and NF-κB signalling pathways may be implicated in H2’s anti-inflammatory effects. This study provides a foundation for further investigation of the protective effects of H2 on COPD. Although we have demonstrated the beneficial effects of H2 on COPD in a mouse model, its efficacy in humans should be assessed in clinical trials.
Acknowledgements
Funding: This work was supported by grants from the National Natural Science Foundation of China (81670041, 81520108001, 81220108001, 81170052), the 973 Key Scheme of China (2015CB553406), the National Key R&D Project (2016YFC0903700), Guangdong Province Universities and Colleges Pearl River Scholar Funded Scheme (2014, W Lu), Guangdong Province Universities and Colleges Key Grant for Innovative Research (cxzd1142), Guangzhou Municipal Research Project (201607020030), Guangzhou Department of Education Innovative Team (13C08), and Guangzhou Department of Education Yangcheng Scholarship (12A001S).
Footnote
Conflicts of Interest: The authors have no conflicts of interest to declare.
Ethical Statement: Animals were housed in the specific pathogen-free facilities and the Animal Care and Use Committee of Guangzhou Medical University approved all experimental protocols. And all methods were performed in accordance with the guidelines and regulations approved by the Animal Care and Use Committee of Guangzhou Medical University.
References
- Lozano R, Naghavi M, Foreman K, et al. Global and regional mortality from 235 causes of death for 20 age groups in 1990 and 2010: a systematic analysis for the Global Burden of Disease Study 2010. Lancet 2012;380:2095-128. [Crossref] [PubMed]
- Jinjuvadia C, Jinjuvadia R, Mandapakala C, et al. Trends in Outcomes, Financial Burden, and Mortality for Acute Exacerbation of Chronic Obstructive Pulmonary Disease (COPD) in the United States from 2002 to 2010. Copd 2017;14:72-9. [Crossref] [PubMed]
- Zuo L, He F, Sergakis GG, et al. Interrelated role of cigarette smoking, oxidative stress, and immune response in COPD and corresponding treatments. Am J Physiol Lung Cell Mol Physiol 2014;307:L205-18. [Crossref] [PubMed]
- Kohansal R, Martinez-Camblor P, Agusti A, et al. The natural history of chronic airflow obstruction revisited: an analysis of the Framingham offspring cohort. Am J Respir Crit Care Med 2009;180:3-10. [Crossref] [PubMed]
- Burge PS, Calverley PM, Jones PW, et al. Randomised, double blind, placebo controlled study of fluticasone propionate in patients with moderate to severe chronic obstructive pulmonary disease: the ISOLDE trial. BMJ 2000;320:1297-303. [Crossref] [PubMed]
- Anthonisen NR, Connett JE, Kiley JP, et al. Effects of smoking intervention and the use of an inhaled anticholinergic bronchodilator on the rate of decline of FEV1. The Lung Health Study. JAMA 1994;272:1497-505. [Crossref] [PubMed]
- Tashkin DP, Celli B, Senn S, et al. A 4-year trial of tiotropium in chronic obstructive pulmonary disease. N Engl J Med 2008;359:1543-54. [Crossref] [PubMed]
- Vestbo J, Sorensen T, Lange P, et al. Long-term effect of inhaled budesonide in mild and moderate chronic obstructive pulmonary disease: a randomised controlled trial. Lancet 1999;353:1819-23. [Crossref] [PubMed]
- Gharib B, Hanna S, Abdallahi OM, et al. Anti-inflammatory properties of molecular hydrogen: investigation on parasite-induced liver inflammation. C R Acad Sci III 2001;324:719-24. [Crossref] [PubMed]
- Xie K, Yu Y, Pei Y, et al. Protective effects of hydrogen gas on murine polymicrobial sepsis via reducing oxidative stress and HMGB1 release. Shock 2010;34:90-7. [Crossref] [PubMed]
- Sun Q, Kang Z, Cai J, et al. Hydrogen-rich saline protects myocardium against ischemia/reperfusion injury in rats. Exp Biol Med (Maywood) 2009;234:1212-9. [Crossref] [PubMed]
- Itoh T, Hamada N, Terazawa R, et al. Molecular hydrogen inhibits lipopolysaccharide/interferon gamma-induced nitric oxide production through modulation of signal transduction in macrophages. Biochem Biophys Res Commun 2011;411:143-9. [Crossref] [PubMed]
- Nakayama M, Nakano H, Hamada H, et al. A novel bioactive haemodialysis system using dissolved dihydrogen (H2) produced by water electrolysis: a clinical trial. Nephrol Dial Transplant 2010;25:3026-33. [Crossref] [PubMed]
- Kajiyama S, Hasegawa G, Asano M, et al. Supplementation of hydrogen-rich water improves lipid and glucose metabolism in patients with type 2 diabetes or impaired glucose tolerance. Nutr Res 2008;28:137-43. [Crossref] [PubMed]
- Nakao A, Toyoda Y, Sharma P, et al. Effectiveness of hydrogen rich water on antioxidant status of subjects with potential metabolic syndrome-an open label pilot study. J Clin Biochem Nutr 2010;46:140-9. [Crossref] [PubMed]
- Ishibashi T, Sato B, Rikitake M, et al. Consumption of water containing a high concentration of molecular hydrogen reduces oxidative stress and disease activity in patients with rheumatoid arthritis: an open-label pilot study. Med Gas Res 2012;2:27. [Crossref] [PubMed]
- Ishibashi T, Sato B, Shibata S, et al. Therapeutic efficacy of infused molecular hydrogen in saline on rheumatoid arthritis: a randomized, double-blind, placebo-controlled pilot study. Int Immunopharmacol 2014;21:468-73. [Crossref] [PubMed]
- Kang KM, Kang YN, Choi IB, et al. Effects of drinking hydrogen-rich water on the quality of life of patients treated with radiotherapy for liver tumors. Med Gas Res 2011;1:11. [Crossref] [PubMed]
- Yoritaka A, Takanashi M, Hirayama M, et al. Pilot study of H(2) therapy in Parkinson's disease: a randomized double-blind placebo-controlled trial. Mov Disord 2013;28:836-9. [Crossref] [PubMed]
- Sun Q, Cai J, Liu S, et al. Hydrogen-rich saline provides protection against hyperoxic lung injury. J Surg Res 2011;165:e43-9. [Crossref] [PubMed]
- Huang CS, Kawamura T, Peng X, et al. Hydrogen inhalation reduced epithelial apoptosis in ventilator-induced lung injury via a mechanism involving nuclear factor-kappa B activation. Biochem Biophys Res Commun 2011;408:253-8. [Crossref] [PubMed]
- Ning Y, Shang Y, Huang H, et al. Attenuation of cigarette smoke-induced airway mucus production by hydrogen-rich saline in rats. PLoS One 2013;8. [Crossref] [PubMed]
- Xiao M, Zhu T, Wang T, et al. Hydrogen-rich saline reduces airway remodeling via inactivation of NF-kappaB in a murine model of asthma. Eur Rev Med Pharmacol Sci 2013;17:1033-43. [PubMed]
- Liu X, Ma C, Wang X, et al. Hydrogen coadministration slows the development of COPD-like lung disease in a cigarette smoke-induced rat model. Int J Chron Obstruct Pulmon Dis 2017;12:1309-24. [Crossref] [PubMed]
- Li D, Wang J, Sun D, et al. Tanshinone IIA sulfonate protects against cigarette smoke-induced COPD and down-regulation of CFTR in mice. Sci Rep 2018;8:376. [Crossref] [PubMed]
- Shu J, Li D, Ouyang H, et al. Comparison and evaluation of two different methods to establish the cigarette smoke exposure mouse model of COPD. 2017;7:15454.
- Luthje L, Raupach T, Michels H, et al. Exercise intolerance and systemic manifestations of pulmonary emphysema in a mouse model. Respir Res 2009;10:7. [Crossref] [PubMed]
- Laucho-Contreras ME, Taylor KL, Mahadeva R, et al. Automated measurement of pulmonary emphysema and small airway remodeling in cigarette smoke-exposed mice. J Vis Exp 2015.52236. [PubMed]
- Zhang K, Wang J, Jiang H, et al. Tanshinone IIA inhibits lipopolysaccharide-induced MUC1 overexpression in alveolar epithelial cells. Am J Physiol Cell Physiol 2014;306:C59-65. [Crossref] [PubMed]
- Shao MX, Ueki IF, Nadel JA. Tumor necrosis factor alpha-converting enzyme mediates MUC5AC mucin expression in cultured human airway epithelial cells. Proc Natl Acad Sci U S A 2003;100:11618-23. [Crossref] [PubMed]
- Murata K, Fujimoto K, Kitaguchi Y, et al. Hydrogen peroxide content and pH of expired breath condensate from patients with asthma and COPD. Copd 2014;11:81-7. [Crossref] [PubMed]
- Kumar A, Takada Y, Boriek AM, et al. Nuclear factor-kappaB: its role in health and disease. J Mol Med (Berl) 2004;82:434-48. [Crossref] [PubMed]
- Chang Y, Al-Alwan L, Alshakfa S, et al. Upregulation of IL-17A/F from human lung tissue explants with cigarette smoke exposure: implications for COPD. Respir Res 2014;15:145. [Crossref] [PubMed]
- Simone RE, Russo M, Catalano A, et al. Lycopene inhibits NF-kB-mediated IL-8 expression and changes redox and PPARgamma signalling in cigarette smoke-stimulated macrophages. PLoS One 2011;6. [Crossref] [PubMed]
- Vos T, Flaxman AD, Naghavi M, et al. Years lived with disability (YLDs) for 1160 sequelae of 289 diseases and injuries 1990-2010: a systematic analysis for the Global Burden of Disease Study 2010. Lancet 2012;380:2163-96. [Crossref] [PubMed]
- Mahesh PA, Jayaraj BS, Chaya SK, et al. Variation in the prevalence of chronic bronchitis among smokers: a cross-sectional study. Int J Tuberc Lung Dis 2014;18:862-9. [Crossref] [PubMed]
- Yoshida T, Tuder RM. Pathobiology of cigarette smoke-induced chronic obstructive pulmonary disease. Physiol Rev 2007;87:1047-82. [Crossref] [PubMed]
- Barnes PJ. Inflammatory mechanisms in patients with chronic obstructive pulmonary disease. J Allergy Clin Immunol 2016;138:16-27. [Crossref] [PubMed]
- Domej W, Oettl K, Renner W. Oxidative stress and free radicals in COPD--implications and relevance for treatment. Int J Chron Obstruct Pulmon Dis 2014;9:1207-24. [Crossref] [PubMed]
- Barnes PJ. Development of new drugs for COPD. Curr Med Chem 2013;20:1531-40. [Crossref] [PubMed]
- Huang CS, Kawamura T, Toyoda Y, et al. Recent advances in hydrogen research as a therapeutic medical gas. Free Radic Res 2010;44:971-82. [Crossref] [PubMed]
- Ohta S. Molecular hydrogen is a novel antioxidant to efficiently reduce oxidative stress with potential for the improvement of mitochondrial diseases. Biochim Biophys Acta 2012;1820:586-94. [Crossref] [PubMed]
- Zhao J, Harper R, Barchowsky A, et al. Identification of multiple MAPK-mediated transcription factors regulated by tobacco smoke in airway epithelial cells. Am J Physiol Lung Cell Mol Physiol 2007;293:L480-90. [Crossref] [PubMed]
- Curran DR, Cohn L. Advances in mucous cell metaplasia: a plug for mucus as a therapeutic focus in chronic airway disease. Am J Respir Cell Mol Biol 2010;42:268-75. [Crossref] [PubMed]
- Qiu X, Li H, Tang H, et al. Hydrogen inhalation ameliorates lipopolysaccharide-induced acute lung injury in mice. Int Immunopharmacol 2011;11:2130-7. [Crossref] [PubMed]
- Ohsawa I, Ishikawa M, Takahashi K, et al. Hydrogen acts as a therapeutic antioxidant by selectively reducing cytotoxic oxygen radicals. Nat Med 2007;13:688-94. [Crossref] [PubMed]