A novel model for dissecting roles of IL-17 in lung transplantation
Introduction
Lung transplantation is the final therapeutic option for patients with end-stage lung disease. However, chronic lung allograft dysfunction (CLAD), classified as azithromycin reversible allograft dysfunction (ARAD), bronchiolitis obliterans syndrome (BOS), and restrictive allograft syndrome (RAS), is the major cause of low long-term survival rates for those patients after lung transplantation (1). Fifty percent primary adult lung transplant recipients developed acute cellular rejection, which has been conformed to be associated with BOS and RAS (2). IL-17 is now recognized to play important roles in the development of CLAD (3), while the precise cellular and molecular mechanisms governing these processes remain to be explored.
Study of T cell immune responses in lung transplant rejection was limited by animal models until the development of mouse left orthotopic lung transplant model. Early studies with this model showed that WT recipients of major histocompatibility complex (MHC) mismatched allografts resulted acute allograft rejection with histological features of T help 1 (Th1) cell response but little obliterative airway lesions development (4-6). Our recent studies showed that Th17 cells and IL-17A plays crucial roles for development of obliterative airway lesions, and the occurrences were reduced with treatment of anti-IL-17A mAbs (7).
Studies on Th17 responses in transplantation have been hindered by interference from Th1 and Th2 responses (8). Direct evidence of Th17 in mediating allograft rejection had so far been mainly observed only when the master Th1 transcription factor T-bet is absent (9), however, absent T-bet also led to absence of IFN-γ production, whose signals are also required for graft acceptance and tolerance induction (10,11). Our previous studies demonstrated that mice lacking both T-bet and the key Th2 transcription factor STAT6 favored Th17 development, and importantly, allow IFN-γ production, indicating they might serve as a better model for examination Th17 in Lung transplantation while without interferences of IFN-γ mediated immunosuppression and tolerance induction (12-14).
Here, we performed orthotopic lung transplants in WT and T-bet/STAT6 DKO mice to examine IL-17 responses in allograft rejection. We showed there were high level of IL-17 expression in lung allografts of the DKO recipients, and allografts developed transplant acute rejection, neutrophilia and obliterative airway inflammation, closely resembling some key features of BOS pathogenesis, demonstrating that T-bet/STAT6 DKO recipients could provide a new viable model to dissect roles of Th17 and IL-17 in lung transplantation.
Methods
Mice
C57BL/6 and BALB/c mice were purchased from Weitong-Lihua Company (Beijing, China). STAT6 KO and T-bet KO (both are C57BL/6 background), were purchased from the Jackson Laboratories (Bar Harbor, ME, USA), and T-bet/STAT6 double knock out (DKO) mice were bred and selected by PCR. All animals were housed in the Laboratory Animal Center at Capital Medical University with safety management guidelines. 9–11 weeks old and 25–28 grams of mice were selected into four groups (5 per group): isograft recipients (isograft group; n=5), allograft WT recipients (allograft WT group; n=5), and allograft DKO recipients (allograft DKO group; n=5). The Laboratory Animal Center at Capital Medical University approved all animal protocols (AEEI-2016-082).
Orthotopic lung transplantation
Orthotopic lung transplantation was performed by using a cuffed technique in the strain combination of C57BL/6→C57BL/6 as the syngeneic group and BALB/c→C57BL/6 as the allogeneic group. All the animal lung transplantation was performed under the protocols of Okazaki et al. (15). Donor mice and recipient mice were sedated with pentobarbital sodium (60 mg/kg) by subcutaneously injection before surgery. All micro operations were performed under the stereoscope (SZ61, Olympus, Japan).
Cell preparations, flow cytometry
Recipient mice were sacrificed at 10 days after lung transplantation, lungs and spleens were harvested. Grafts and spleen were processed and cells were prepared as described previously (4). Antibodies phycoerythrin (PE)-labeled anti-CD3, allophycocyanin (APC)-labeled anti-CD4 and FITC-labeled anti-CD8 mAbs were purchased from BD Pharmingen (San Diego, CA, USA). Staining procedure was performed under the protocols described previously (16), and a Guava easy Cyteinstrument and Flowjo software were used to analyses the flow cytometry (Merck Millipore Co., Ltd., NH, USA).
Histologic analysis and rejection pathology scoring
Grafts were cut into squares and fixed in 4% formaldehyde, embedded in paraffin, sectioned into 5µm ply, stained with hematoxylin-eosin, Masson’s Trichrome and antimyeloperoxidase which were purchased from Abcam (Cambridge, MA, USA).
Rejection and fibrosis pathology scoring of stained sections was concluded by four blinded viewers with the criterion described previously, and the following show is detail points (0–4) and symptoms of scoring for criterion (“S” scores): S0= no apparent inflammation cell invasion, S1= inflammation cell invades around peribronchial or perivascular, S2= inflammatory cell invades in lung interstitium, S3= inflammatory cell invades in pulmonary, S4= inflammatory cell invades in lumens of bronchus (17). Total number of neutrophils in per 400× field on sections will be account to analyze the levels of neutrophilic inflammation. For quantification of fibrosis, a previously published method was used (18).
Quantitative real-time RT-PCR
Total RNA was extracted from grafts by using Axy Prep Multisource Total RNA Miniprep Kit 250-prep (Axygn Co., Ltd., SF, USA) according to manufacturers’ instructions, reversed transcribed into cDNA by ProtoScript II Fist Strand cDNA Synthesis Kit (New England Biolabs, Ipswich, MA, USA). The primers sequence of IL-17A, IL-6, IFN-γ, IL-22, IL-4 and cyclophilin A (a housekeeping gene using as control) synthesized by Invitrogen were listed below: IL-17A: sense strand 5'-TTT AAC TCC CTT GGC GCA AAA-3', antisense strand 5'-CTT TCC CTC CGC ATT GAC AC-3', IL-6: sense strand 5'-GTC ACA GAA GGA GTG GCT A-3', antisense strand 5'-AGA GAA CAA CAT AAG TCA GAT ACC-3', IFN-γ: sense strand 5'-ATG AAC GCT ACA CAC TGC ATC-3', antisense strand 5'-CCA TCC TTT TGC CAG TTC CTC-3', IL-22: sense strand 5'-ATG AGT TTT TCC CTT ATG GGG AC-3', antisense strand 5'-GCT GGA AGT TGG ACA CCT CAA-3', IL-4: sense strand 5'-GGT CTC AAC CCC CAG CTA GT-3', antisense strand 5'-GCC GAT GAT CTC TCT CAA GTG AT-3'. Reactions were performed in the Roter Gene Q System (Qiagen, Valencia, CA, USA) with SYBR Green PCR kit (Qiagen, Valencia, CA, USA), and repeated at least three times.
Statistical analysis
Statistics were performed with Graphpad Prism Software version 5.0 (San Diego, CA, USA) and SPSS19.0 (SPSS, Inc., Chicago, IL, USA). Student t-test and one-way analysis of variance (ANOVA) were used for analyzing variables. P<0.05 was considered statistically significant.
Results
Modification of surgery techniques and outcomes
Mouse orthotopic lung transplantations were performed as we previously described (7), with further modification and simplification. For the study period, we accomplished 80 lung transplantations with a 90% success rate. Mice in failed cases died of different reasons including venous tearing (three in syngeneic group), artery torsion and artery aeroembolism (two in allogeneic WT group), and severe lung and thoracic cavity infection on day one or two (one in allogeneic WT and two in DKO allogeneic groups). The whole operation time was controlled within two hours. The warm ischemia time of donor lung was about 15 minutes and the cold ischemia time was about one hour.
Lung grafts of recipients were harvested at 10 days after transplantation. Lung isografts showed a normal appearance (Figure 1A), whereas allografts of WT recipients displayed edema and hyperaemia (Figure 1B). Allografts of DKO recipients showed different characteristics compared to that of WT recipients, with vigorous rejection, inflammatory response sand fibrosis development (Figure 1C).
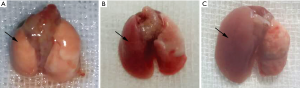
Transplant rejection analysis and flow cytometry evaluation
Cellular infiltration and levels of fibrosis of grafts were assessed by histopathological analysis. No obvious cellular inflammatory appearance, fibrosis and airway lesions were observed in the lung grafts from syngeneic group 10 days after lung transplantation. Comparison of allografts of WT and DKO recipients revealed that inflammatory cellular infiltrate of pulmonary interstitium was similar (Figure 2A). However, graft infiltrating cells of WT recipients were mainly lymphocytes while that of DKO recipients displayed a mixed phenotype with numerous polymorphonuclear cells (Figure 2B). Grafts of DKO recipients showed very distinct BOS lesions with fibrotic and mix cells plugs growing in the airways, whereas neither lesions of airway epithelium nor prominent development of BOS were observed in that of WT recipients (Figure 2C,D). Allografts of WT recipients largely maintained intact airway epithelium, despite developing an acute rejection. Allografts Rejection of WT recipients appeared less vigorous than that of DKO recipients which developed additional luminal obliteration (Figure 3A). Allografts of DKO recipients displayed severer perivascular and peribronchiolar fibrosis as compared with WT recipients (Figure 3B). However, levels of parenchymal fibrosis in the two groups were similar (Figure 3C). Embolus in luminal obliteration of DKO recipients were mainly composed of neutrophils (Figure 4A,B), as well as higher numbers of neutrophils infiltration in grafts compared to that of WT recipients 10 days after transplantation (Figure 4C). Analysis of infiltrating lymphocytes from grafts in DKO group also revealed the ratio of CD8:CD4 increased compared to WT allografts or isografts (Figure 4D,E). In summary, these data demonstrated pathologic characteristics of allografts rejection of DKO recipients differed from WT recipients as they developed pathological features more closely resembling to BOS pathogenesis.
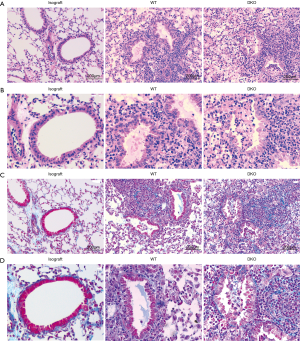
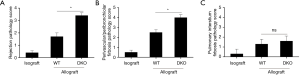
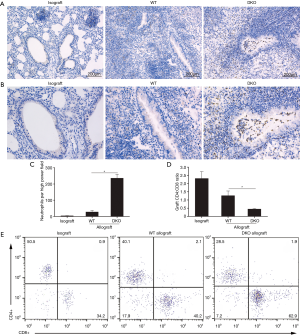
Cytokine expression analysis
To further determine cellular and molecular mechanisms of transplant immunity, we measured cytokines expression of grafts at 10 days after lung transplantation. As shown in Figure 5A, levels of IL-17A expression increased in allografts of WT recipients compared to isografts, and allografts of DKO recipients showed markedly even higher IL-17A expression than that of WT recipients; Expression of Th17 family cytokine IL-22 showed a similar fashion with IL-17A (Figure 5B). IFN-γ expression were increased in allografts than that of isografts, and expressed at comparable level of DKO and WT recipients. Although not as dramatic as IL-17A or IL-22 (Figure 5C), expression of inflammatory cytokine IL-6 was also significantly higher in DKO recipients compared to WT recipients and the controls (Figure 5D), while reduced IL-4 production was observed in allografts of DKO than that of WT recipients (Figure 5E). Together, our data demonstrated lung allografts of T-bet/STAT6 DKO recipients result in IL-17-dominant transplant immunity, retain IFN-γ responses, and develop obliterative airway inflammation and acute transplant rejection, indicating that T-bet/STAT6 DKO mice serving as allograft recipients could be utilized as a new viable model to study the roles of IL-17 in lung transplantation.
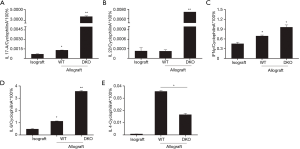
Discussion
Chronic lung allograft dysfunction (CLAD) is the main factor to limit the long-term success after lung transplantation (19,20). In addition to classical bronchiolitis obliterans syndrome (BOS), new forms of CLAD including azithromycin reversible allograft dysfunction (ARAD) and restrictive allograft syndrome (RAS, rCLAD) have been recognized (21). ARAD is characterized as an obstructive decline in pulmonary function, increased neutrophils in bronchoalveolar lavage, and reversible by azithromycin treatment (22). RAS displays restrictive functional changes with fibrotic processes in peripheral lung tissue. thirty percent of CLAD patients after lung transplantation develop RAS, and their survival rates were significantly worse than patients diagnosed with BOS (23,24).
Th17 immunity has been shown to contribute to all phases of allograft rejection including acute, chronic and antibody-mediated rejections (25). Studies have demonstrated that Th17 cells play an important role in CLAD development. IL-17 is critical in mediating early post-transplant lesions after trachea allotransplantation (26). IL-17 deficient recipients treated with cyclosporine A are protected against developing obliterative airway disease after heterotopic allogeneic trachea transplantation (27). Neutralization of IL-17 has been shown to prevent the development of BOS (3), and inhibition of IL-17 by halofuginone, a plant derivative, ameliorates the features of chronic lung allograft dysfunction in a minor alloantigen-mismatched murine orthotopic lung transplant model (28). Th17 immunity is critical for autoimmunity against collagen type V in the progression of BOS (29). IL-17 down-regulates complement regulatory protein, and enhances complement activation in the development of BOS (30). However, a very recent report showed that IL-17A-mediated immunity is only involved in the inflammatory component after lung transplantation (31).
Our results here showed, as compared with WT recipients, that recipients deficient in both T-bet and STAT6 resulted in significantly higher levels of IL-17 expression, which led to stronger inflammatory responses, supporting the notion that IL-17 plays a dominant role in the inflammatory responses during lung transplant rejection. Infiltrating neutrophils are commonly observed in chronically rejecting allografts and IL-17 is a potent driver of neutrophil infiltration. Our observation that lung allografts of DKO recipients display heavier neutrophil infiltration and bronchovascular inflammation and severer airway fibrosis further confirms the role of IL-17 in CLAD pathogenesis, and also consists with a recent report demonstrating that IL-17 signals are crucial to murine airway fibrosis (32).
In agreement with the observation by Yamada et al. that IL-17 had little influence on the degree of pulmonary fibrosis (31), elevated levels of IL-17 expression in DKO recipients did not lead to significant parenchymal fibrosis. The differential effects of IL-17 on pulmonary air way and interstitial tissue could be due to their distinct cellular composition and immune microenvironment, also suggesting that the IL-17- associated airway fibrosis likely indirect and requires engagement of several cell types and their associated molecules. The different influences of IL-17 on airway fibrosis between our observation and those by Yamada et al. may be due to the different levels of IL-17 expression and neutrophil infiltration, as they did observe a trend that deficiency of IL-17 reduced neutrophil infiltration and fibrosis pathology, albeit not significantly. Recent studies demonstrated that Th1/IFN-γ signals could also play important roles in the development of fibrosis (33,34), and it is known that Th1 and Th17 signals and responses are counter-regulated (35). Thus, the absence of significant amelioration of fibrosis in IL-17 deficient recipients after lung transplantation (31) could be due to the compensation of Th1 signals.
In summary, our results showed that T-bet/STAT6 DKO recipients of lung allografts result in IL-17-dominant transplant immunity and meanwhile retain Th1/IFN-γ responses, without altering the characteristics of acute rejection. There is no significant difference in the inflammatory infiltration of pulmonary interstitium between DKO and WT groups, indicating that T-bet/STAT6 double deficiency does not influence the process of acute rejection after lung transplantation. The higher levels of IL-17 expression lead to heavier neutrophil infiltration and severer inflammatory responses, and display some key features of CLAD. Our results indicate that T-bet/STAT6 DKO mice serving as allograft recipients could be utilized as a new viable model to study the roles of IL-17 in lung transplantation.
Conclusions
Due to interference from Th1 and Th2 responses, study of Th17 responses during transplantation has been difficult. Here, we showed, by eliminating potential influence from elevated Th2 responses in T-bet deficiency or increased Th1 responses in STAT6 deficiency, that the T-bet/STAT6 double deficiency allows for robust Th17 development, separates it from Th1 and Th2 interferences, and thus provides the opportunity to study Th17 responses. Our results showed that T-bet/STAT6 DKO recipients of lung allografts result in IL-17-dominant transplant immunity and meanwhile retain Th1/IFN-γ responses, without altering the characteristic of acute rejection after transplantation. The higher levels of IL-17 expression lead to heavier neutrophil infiltration and severer inflammatory responses, and display some key features of BOS. Our results indicate that T-bet/STAT6 DKO mice serving as allograft recipients could be utilized as a new viable model to study the roles of IL-17 in lung transplantation.
Study limitations
Our observation showed that lung allografts of T-bet/STAT6 DKO recipients display some typical features of airway luminal obliteration pathology, however it is the result of strong immune responses with mixed acute rejection and amplified Th17 immunity, which is significantly different from clinical chronic lung allograft rejection. This DKO model is not able to resemble key features of RAS either. Even so, our model will still be very useful for dissecting and understanding the cellular and molecular mechanisms of IL-17/Th17 mediated alloimmunity after lung transplantation, and gain valuable scientific knowledge for eventual clinical application.
Acknowledgements
Funding: This work was supported by the National Natural Science Foundation of China Grants 81370188(to Y Ding) and 81770092(to J Xu).
Footnote
Conflicts of Interest: The authors have no conflicts of interest to declare.
Ethical Statement: The Laboratory Animal Center at Capital Medical University approved all animal protocols (AEEI-2016-082).
References
- Lodhi SA, Lamb KE, Meier-Kriesche HU. Solid organ allograft survival improvement in the United States: the long-term does not mirror the dramatic short-term success. Am J Transplant 2011;11:1226-35. [Crossref] [PubMed]
- Yusen RD, Edwards LB, Kucheryavaya AY, et al. The registry of the International Society for Heart and Lung Transplantation: thirty-first adults lung and heart-lung transplant report—2014; focus theme: retransplantation. J Heart Lung Transplant 2014;33:1009-24. [Crossref] [PubMed]
- Fan L, Benson HL, Vittal R, et al. Neutralizing IL-17 prevents obliterative bronchiolitis in murine orthotopic lung transplantation. Am J Transplant 2011;11:911-22. [Crossref] [PubMed]
- Dodd-o JM, Lendermon EA, Miller HL, et al. CD154 blockade abrogates allospecific responses and enhances CD4(+) regulatory T-cells in mouse orthotopic lung transplant. Am J Transplant 2011;11:1815-24. [Crossref] [PubMed]
- Okazaki M, Gelman AE, Tietjens JR, et al. Maintenance of airway epithelium in acutely rejected orthotopic vascularized mouse lung transplants. Am J Respir Cell Mol Biol 2007;37:625-30. [Crossref] [PubMed]
- Okazaki M, Sugimoto S, Lai J, et al. Costimulatory Blockade–Mediated Lung Allograft Acceptance Is Abrogated by Overexpression of Bcl-2 in the Recipient. Transplant Proc 2009;41:385-7. [Crossref] [PubMed]
- Chen QR, Wang LF, Xia SS, et al. Role of interleukin-17A in early graft rejection after orthotopic lung transplantation in mice. J Thorac Dis 2016;8:1069-79. [Crossref] [PubMed]
- Yi T, Chen YL. Reciprocal differentiation and tissue-specific pathogenesis of Th1, Th2, and Th17 cells in graft-versus-host disease. Blood 2009;114:3101-12. [Crossref] [PubMed]
- Burrell BE, Csencsits K, Lu G, et al. CD8+ Th17 mediate costimulation blockade-resistant allograft rejection in T-bet-deficient mice. J Immunol 2008;181:3906-14. [Crossref] [PubMed]
- Konieczny BT, Dai Z, Elwood ET, et al. IFN-gamma is critical for long-term allograft survival induced by blocking the CD28 and CD40 ligand T cell costimulation pathways. J Immunol 1998;160:2059-64. [PubMed]
- Kishimoto K, Sandner S, Imitola J, et al. Th1 cytokines, programmed cell death, and alloreactive T cell clone size in transplant tolerance. J Clin Invest 2002;109:1471-9. [Crossref] [PubMed]
- Yang Y, Xu J, Niu Y, et al. T-bet and eomesodermin play critical roles in directing T cell differentiation to Th1 versus Th17. J Immunol 2008;181:8700-10. [Crossref] [PubMed]
- Xu J, Yang Y, Qiu G, et al. c-Maf regulates IL-10 expression during Th17 polarization. J Immunol 2009;182:6226-36. [Crossref] [PubMed]
- Xu J, Yang Y, Qiu G, et al. Stat4 is critical for the balance between Th17 cells and regulatory T cells in colitis. J Immunol 2011;186:6597-606. [Crossref] [PubMed]
- Okazaki M, Krupnick AS, Kornfeld CG, et al. A mouse model of orthotopic vascularized aerated lung transplantation. Am J Transplant 2007;7:1672-9. [Crossref] [PubMed]
- Prussin C, Metcalfe DD. Detection of intracytoplasmic cytokine using flow cytometry and directly conjugated anti-cytokine antibodies. J Immunol Methods 1995;188:117-8. [Crossref] [PubMed]
- Lendermon EA, Doddo JM, Coon TA, et al. CD8+IL-17+ T Cells Mediate Neutrophilic Airway Obliteration in T-bet–Deficient Mouse Lung Allograft Recipients. Am J Respir Cell Mol Biol 2015;52:622-33. [Crossref] [PubMed]
- Hübner RH, Gitter W, El Mokhtari NE, et al. Standardized quantification of pulmonary fibrosis in histological samples. Biotechniques 2008;44:507-11. [Crossref] [PubMed]
- Mauck KA, Hosenpud JD. The bronchial epithelium: a potential allogeneic target for chronic rejection after lung transplantation. J Heart Lung Transplant 1996;15:709-14. [PubMed]
- Knoop C, Estenne M. Acute and chronic rejection after lung transplantation. Semin Respir Crit Care Med 2006;27:521-33. [Crossref] [PubMed]
- Gauthier JM, Hachem RR, Kreisel D. Update on Chronic Lung Allograft Dysfunction. Curr Transplant Rep 2016;3:185-91. [Crossref] [PubMed]
- Vos R, Verleden SE, Ruttens D, et al. Azithromycin and the treatment of lymphocytic airway inflammation after lung transplantation. Am J Transplant 2014;14:2736-48. [Crossref] [PubMed]
- Sato M, Waddell TK, Wagnetz U, et al. Restrictive allograft syndrome (RAS): A novel form of chronic lung allograft dysfunction. J Heart Lung Transplant 2011;30:735-42. [Crossref] [PubMed]
- Ofek E, Sato M, Saito T, et al. Restrictive allograft syndrome post lung transplantation is characterized by pleura parenchymal fibroelastosis. Mod Pathol 2013;26:350-6. [Crossref] [PubMed]
- Abadja F, Sarraj B, Ansari MJ. Significance of T helper 17 immunity in transplantation. Curr Opin Organ Transplant 2012;17:8-14. [Crossref] [PubMed]
- Lemaître PH, Vokaer B, Charbonnier LM, et al. IL-17A mediates early post-transplant lesions after heterotopic trachea allotransplantation in Mice. PLoS One 2013;8. [Crossref] [PubMed]
- Lemaître PH, Vokaer B, Charbonnier LM, et al. Cyclosporine A drives a Th17- and Th2-mediated posttransplantobliterative airway disease. Am J Transplant 2013;13:611-20. [Crossref] [PubMed]
- Oishi H, Martinu T, Sato M, et al. Halofuginone treatment reduces interleukin-17A and ameliorates features of chronic lung allograft dysfunction in a mouse orthotopic lung transplant model. J. Heart Lung Transplant 2016;35:518-27. [Crossref] [PubMed]
- Burlingham WJ, Love RB, Jankowska-Gan E, et al. IL-17-dependent cellular immunity to collagen type V predisposes to obliterative bronchiolitis in human lung transplants. J Clin Invest 2007;117:3498-506. [Crossref] [PubMed]
- Suzuki H, Lasbury ME, Fan L, et al. Role of complement activation in obliterative bronchiolitis post-lung transplantation. J Immunol 2013;191:4431-9. [Crossref] [PubMed]
- Yamada Y, Vandermeulen E, Heigl T, et al. The role ofrecipient derived interleukin-17A in a murine orthotopic lung transplant model of restrictive chronic lung allograft dysfunction. Transpl Immunol 2016;39:10-7. [Crossref] [PubMed]
- Yanagisawa H, Hashimoto M, Minagawa S, et al. Role of IL-17A in murine models of COPD airway disease. Am J Physiol Lung Cell Mol Physiol 2017;312:L122-30. [Crossref] [PubMed]
- Fielding CA, Jones GW, McLoughlin RM, et al. Interleukin-6 Signaling Drives Fibrosis in Unresolved Inflammation. Immunity 2014;40:40-50. [Crossref] [PubMed]
- Nevers T, Salvador AM, Velazquez F, et al. Th1 effector T cells selectively orchestrate cardiac fibrosis in nonischemic heart failure. J Exp Med 2017;214:3311-29. [PubMed]
- Patel DD, Kuchroo VK. Th17 Cell Pathway in Human Immunity: Lessons from Genetics and Therapeutic Interventions. Immunity 2015;43:1040-51. [Crossref] [PubMed]