Interatrial block predicts atrial fibrillation in patients with carotid and coronary artery disease
Introduction
Interatrial block (IAB) is a conduction delay between the activation of the atria, manifested as a P-wave duration ≥120 ms on the surface electrocardiograph (ECG) (1). IAB has been associated with the development of atrial fibrillation (AF) in diverse cardiac populations (2,3). IAB is thought to be a consequence of fibrosis of the Bachmann region, the largest interatrial conduction pathway. Prior studies have demonstrated an association between diffuse coronary artery disease and IAB (4). While the pathophysiologic mechanisms of IAB have not yet been entirely elucidated, it has been hypothesized that Bachmann region fibrosis due to atrial ischemia or necrosis may play a role. We analyzed a population of patients with coronary artery disease undergoing angiography who also received carotid ultrasonography as part of a study protocol to determine non-invasive predictors of AF as well as variables associated with IAB.
Methods
Patient selection
A total of 355 outpatients referred for clinically indicated coronary angiography were recruited from the cardiac catheterization laboratory at the Kingston Health Sciences Center (KHSC) as a part of an ongoing study of peripheral arterial and coronary artery disease and retrospectively analyzed (5). Inclusion criteria were defined as: (I) patients aged ≥18 years able to provide consent; (II) non-emergent outpatients referred for a clinically indicated angiogram for one of: nonspecific chest pain evaluation; stable or unstable angina pectoris; positive stress test; preoperative assessment; old or recent myocardial infarction (MI) (>2 days); (III) a follow-up ECG conducted at KHSC within 12 months of the index angiogram. Exclusion criteria included any contraindication to angiography or ultrasound, AF on initial ECG, lack of measurable P-waves in the inferior leads, and any device pacing the atrium. All patients underwent carotid ultrasound on the same day as their coronary angiography as part of the study protocol and had a baseline ECG completed within 1 month of the date of their angiogram and ultrasound. Ethics approval was obtained from the Queen’s University Health Sciences Research Ethics Board (file # 6019790).
Carotid ultrasound and angiography analysis
Carotid ultrasound and angiogram results were interpreted by separate investigators who were blinded to the results of the other study and the ECG analysis. Angiography was performed by an experienced interventional cardiologist at KHSC using the standard Judkins technique on a General Electric System 2100. Angiograms were quantified using a previously established scoring system (5). Significant coronary artery stenosis was defined as luminal diameter reduction of ≥70% within any segment of the main branches of the artery examined or ≥50% stenosis for the left main coronary artery.
Carotid ultrasound was performed immediately post-angiography during the recovery period with an average time to completion of 30 minutes according to a published protocol. Carotid intima-media thickness (CIMT) and plaque measurements were quantified by two independent investigators using the GE Healthcare EchoPAC software. Exact methods for the carotid quantification including intima-media thickness, plaque height and plaque area have been previously described by our group, in the literature (6).
ECG analysis
A standard 12-lead ECG (filter 150 Hz, 25 mm/s, 10 mm/mV) was obtained in all patients and images were amplified ×8 and analyzed using ICONICO semi-automatic calipers. Each ECG was blindly reviewed by two independent reviewers (S Haseeb, H van Rooy), different from the reviewers of the angiogram and carotid ultrasound. P-wave duration was measured according to the most recent consensus guidelines on IAB (1). The P-wave onset was defined as the point of initial upward or downward deflection from the isoelectric line and the offset was defined as the return of the waveform to the initial baseline. Partial IAB was defined as a prolongation of the P-wave duration ≥120 milliseconds in the inferior leads (II, III and aVF) and advanced IAB was defined as a prolongation of the P-wave duration ≥120 milliseconds with a biphasic (+/−) morphology in the inferior leads (7). This method has been previously described and validated with high interobserver and intraobserver correlation coefficients (8). P-wave voltage was measured from the peak of the P-wave to the isoelectric line of the TP interval in lead I.
Clinical variables/follow-up
Patient charts were reviewed for baseline demographic and clinical data. All available clinical records, ECG’s and Holter monitors were reviewed for the presence of AF. Patients were followed until their last available clinic appointment.
Statistical methods
Data were collected in Excel and imported into IBM SPSS (version 24.0 for Windows) for statistical analysis. Patient characteristics were compared for patients with IAB (either partial or advanced) versus those without IAB. Data were initially described using means and standard deviations for continuous data, and frequencies and percentages for categorical data. This was followed by a univariate analysis to assess the association of the collected data with the outcome using one-way ANOVA and independent sample t-test for the normally distributed continuous data, Mann-Whitney for continuous data that was not normally distributed and chi-square tests (Pearson or Fisher’s Exact as appropriate) for the categorical data.
Cox proportional hazard analysis was conducted to determine the difference in mean AF free survival between patients with IAB versus those without.
Logistic regression analysis was performed to select independent predictors of AF and IAB using all variables included in Table 1 as potential candidates, including demographics, comorbidities, medications, angiographic and ultrasonographic variables. Variables that were co-linear with other variables were removed from the model and the models were progressively refined until the strongest significant predictors remained.
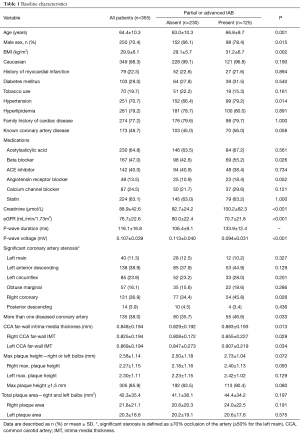
Full table
Baseline characteristics
Data are described as n (%) or mean ± SD. *, significant stenosis is defined as ≥70% occlusion of the artery (≥50% for the left main). CCA, common carotid artery; IMT, intima-media thickness.
Results
Population demographics
The study population consisted of 355 patients who met the inclusion/exclusion criteria and provided consent. The mean population age was 64.4 years, 70.4% male and had a mean BMI of 29.9 kg/m2. A total of 32.1% of the population had partial IAB and 3.1% of the population had advanced IAB. The population was normally distributed in terms of P-wave duration with a mean P-wave duration of 116.1±16.8 ms (Table 1). There was no mortality during the mean follow-up of 59.0 months.
Development of AF
The incidence of new-onset AF in this population was 19.4%. Significant multi-vessel coronary artery disease was associated with the development of new-onset AF (1.19 vs. 2.00 arteries; P<0.001). An increased carotid bulb maximum plaque height was associated with development of AF (2.44 vs. 2.99 mm; P=0.001). This effect was present individually in both the right carotid bulb (2.17 vs. 2.57 mm; P=0.015) and the left carotid bulb (2.20 vs. 2.55 mm; P=0.027). Increased total carotid plaque area was also associated with new-onset AF (38.0 vs. 53.9 mm2; P=0.001) (Table 2). Patients who developed AF had a longer mean P-wave duration than those who did not (114.5 vs. 120.6 ms; P=0.009). Patients who developed AF had a higher prevalence of IAB than those without (52.5% vs. 29.9%; P=0.001) (Table 2). Predictors for the development of AF on multivariate analysis can be seen in Table 3. There was a significant difference in AF free survival time between patients with IAB and those without IAB as seen in Figure 1.
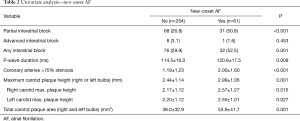
Full table
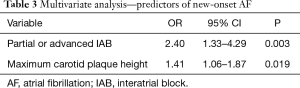
Full table
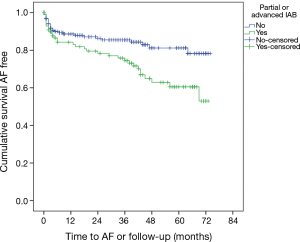
Clinical variables associated with IAB
Clinical variables of patients with and without IAB can be seen in Table 1. Patients with IAB had significantly greater carotid artery atherosclerotic burden determined by CIMT (0.883±0.193 vs. 0.829±0.192 mm; P=0.013). This effect was present individually in both the left common carotid artery (CCA) (0.907±0.219 vs. 0.847±0.273; P=0.034) and the right CCA (0.855±0.227 vs. 0.808±0.172 mm; P=0.029) (Table 1).
A logistic regression model for predictors of IAB revealed that multivessel coronary artery disease and CIMT were collinear with each other and therefore multivessel disease was removed from the model in favor of CIMT (Table 4). The strongest predictors of IAB on multivariate analysis were BMI ≥30 kg/m2 (OR =3.14, 95% CI: 1.14–6.71; P=0.003), P-wave voltage <0.10 mV in lead 1 (OR =1.97, 95% CI: 1.23–3.15, P=0.004), male sex (OR =1.78, 95% CI: 1.05–3.03; P=0.034), increased mean CIMT (per 0.1 mm increase) (OR =1.75, 95% CI: 1.00–3.07; P=0.050) and increased age (per 10-year increase) (OR =1.46, 95% CI: 1.14–1.88; P=0.003). This model was significant overall and explained 11.4% of the variability in the prevalence of IAB in this population (P≤0.001; R2=0.114).
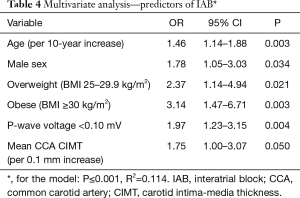
Full table
Discussion
The primary finding of this study is that IAB is a predictor of new-onset AF in a population of patients with carotid and coronary artery disease. In addition, several associations with IAB have been identified, including increased CIMT and multivessel coronary artery disease. IAB is important due to its wide presence in the older population and has been previously associated with AF. The prevalence of IAB increases with age, and in the general population is over 40% in septuagenarians and over 50% in octogenarians (9). IAB has been associated with development or recurrence of AF in many different cardiac populations (10). The mechanism connecting IAB to AF is thought be a result of fibrosis of the Bachmann region, leading to the hypothesis that advanced atherosclerotic processes could be involved in the pathogenesis of IAB (11,12). A registry of patients with IAB is currently ongoing to better characterize the association of IAB and AF (13).
The ability of fibrosis to delay or block cardiac conduction has been well documented (14). Evidence for the Bachmann region being the major atrial structure involved has been demonstrated through experimental ligation of the Bachmann bundle in canine models. This experimental ligation produced the classic prolonged biphasic P-wave of advanced IAB (15). In addition, electrophysiological study of patients with IAB has demonstrated that the timing of activation over the left atrial roof is consistent with blockade of the Bachmann region (16). Recent studies have demonstrated a link between ischemia, fibrosis and delay of conduction in the Bachmann region. A study by Álvarez-García et al. prospectively evaluated the effects of selective atrial coronary artery occlusion in humans (17). Patients who had accidental occlusion of the atrial branches during coronary angioplasty were found to have a three-time greater incidence of new-onset IAB. Other studies have demonstrated a link between coronary artery disease and IAB (18), or have shown structural remodeling of the Bachmann region in patients with IAB (19).
A recent study by our group has shown an association between multi-vessel coronary artery disease and IAB in a population of patients presenting with NSTEMI (69.8% with IAB had multi-vessel disease vs. 48.1% without IAB had multivessel disease; P=0.026) (4). These findings indicate a potential link between diffuse coronary atherosclerosis and IAB. The current study sought to expand the link between diffuse atherosclerosis and IAB to include atherosclerosis found outside of the coronary artery tree. Interestingly, patients with IAB had a significantly higher BMI, an increased age, and were more likely to be male on multivariate analysis; factors that have traditionally been known as risk factors for atherosclerotic disease. Patients who were identified as having IAB in this study had significantly increased markers of carotid disease as well as a higher prevalence of multi-vessel coronary artery disease. However due to substantial collinearity, carotid and coronary artery markers were not able to be included in the same multivariate model. An interesting observation from this study is that IAB, which is in part due to a fibrotic process, is associated with CIMT and not plaque. However, this finding is consistent with the thought that CIMT and plaque are different phenotypes. An increased CIMT is mostly due to increased medial hypertrophy that is associated with cardiac risk factors such as hypertension leading to sclerosis or stiffness of the arterial wall (20,21). While such vessel wall damage can proceed to plaque deposition, plaque itself is thought to be an intimal process. This may explain why IAB is more closely associated with a potentially fibrotic process of the arterial wall (sclerosis) as opposed to atherosis. In patients with advanced atherosclerotic processes diastolic dysfunction is another possible mechanism of both increased CIMT and AF development. The findings of this study indicate that carotid bulb plaque height in combination with IAB are possible early and non-invasive predictors of AF occurrence in the studied population.
This study was performed at a single center with a large Caucasian population. The retrospective nature of the study implies a potential for inherent bias. While patients with prior documented episodes of AF were excluded from the study, it is possible that patients with prior undocumented episodes were erroneously included. In addition, because AF was captured only from follow-up appointments (ECG and Holters) it is possible that silent AF episodes may have gone unreported. The absence of systematic ECG/Holter follow-up decreases the sensitivity of this study to identify incident AF.
Conclusions
IAB is a predictor of new-onset AF in patients with carotid and coronary artery disease. Both carotid and coronary artery disease are associated with a higher prevalence of IAB.
Acknowledgements
None.
Footnote
Conflicts of Interest: The authors have no conflicts of interest to declare.
Ethical Statement: The study was approved by the Queen’s University Health Sciences Research Ethics Board (file # 6019790) and written informed consent was obtained from all patients.
References
- Bayés de Luna A, Platonov P, Cosio FG, et al. Interatrial blocks. A separate entity from left atrial enlargement: A consensus report. J Electrocardiol 2012;45:445-51. [Crossref]
- Tse G, Wong CW, Gong M, et al. Predictive value of inter-atrial block for new onset or recurrent atrial fibrillation: A systematic review and meta-analysis. Int J Cardiol 2018;250:152-6. [Crossref]
- Bernal E, Bayés-Genís A, Ariza-Solé A, et al. Interatrial block, frailty and prognosis in elderly patients with myocardial infarction. J Electrocardiol 2018;51:1-7. [Crossref]
- Alexander B, MacHaalany J, Lam B, et al. Comparison of the Extent of Coronary Artery Disease in Patients With Versus Without Interatrial Block and Implications for New-Onset Atrial Fibrillation. Am J Cardiol 2017;119:1162-5. [Crossref]
- Johri AM, Calnan CM, Matangi MF, et al. Focused Vascular Ultrasound for the Assessment of Atherosclerosis: A Proof-of-Concept Study. J Am Soc Echocardiogr 2016;29:842-9. [Crossref]
- Johri AM, Behl P, Hétu MF, et al. Carotid Ultrasound Maximum Plaque Height-A Sensitive Imaging Biomarker for the Assessment of Significant Coronary Artery Disease. Echocardiography 2016;33:281-9. [Crossref]
- Baranchuk A, Bayés de Luna A. The P-wave morphology: what does it tell us? Herzschrittmacherther Elektrophysiol 2015;26:192-9. [Crossref]
- Kizilirmak F, Demir GG, Gokdeniz T, et al. Changes in Electrocardiographic P Wave Parameters after Cryoballoon Ablation and Their Association with Atrial Fibrillation Recurrence. Ann Noninvasive Electrocardiol 2016;21:580-7. [Crossref]
- Martínez-Sellés M. Prevalence and incidence of interatrial block in global population and in different clinical situations. J Geriatr Cardiol 2017;14:158-60.
- Baranchuk A, Enriquez A, Antiperovitch P, et al. Advanced interatrial block as a key marker for atrial fibrillation recurrence : Bayés’ syndrome. J Geriatr Cardiol 2017;14:169-73.
- Legato MJ, Bull MB, Ferrer MI. Atrial ultrastructure in patients with fixed intra-atrial block. Chest 1974;65:252-61. [Crossref]
- Becker AE. How structurally normal are human atria in patients with atrial fibrillation? Hear Rhythm 2004;1:627-31. [Crossref]
- Martínez-Sellés M, Baranchuk A, Elosua R, et al. Rationale and design of the BAYES (Interatrial Block and Yearly Events) registry. Clin Cardiol 2017;40:196-9. [Crossref]
- Marrouche NF, Wilber D, Hindricks G, et al. Association of atrial tissue fibrosis identified by delayed enhancement MRI and atrial fibrillation catheter ablation: the DECAAF study. JAMA 2014;311:498-506. [Crossref]
- Waldo AL, Bush HL Jr, Gelband H, et al. Effects on the canine P wave of discrete lesions in the specialized atrial tracts. Circ Res 1971;29:452-67. [Crossref]
- Cosío F, Martín-Peñato A, Pastor A, et al. Atrial Activation Mapping in Sinus Rhythm in the Clinical Electrophysiology Laboratory: Observations During Bachmann’s Bundle Block. J Cardiovasc Electrophysiol 2004;15:524-31. [Crossref]
- Álvarez-García J, Vives-Borrás M, Gomis P, et al. Electrophysiological Effects of Selective Atrial Coronary Artery Occlusion in Humans. Circulation 2016;133:2235-42. [Crossref]
- Ariyarajah V, Fernandes J, Apiyasawat S, et al. Angiographic Localization of Potential Culprit Coronary Arteries in Patients With Interatrial Block Following a Positive Exercise Tolerance Test. Am J Cardiol 2007;99:58-61. [Crossref]
- Saremi F, Channual S, Krishnan S, et al. Bachmann Bundle and its arterial supply: imaging with multidetector CT--implications for interatrial conduction abnormalities and arrhythmias. Radiology 2008;248:447-57. [Crossref]
- Spence JD, Rundek T. Toward Clinical Applications of Carotid Ultrasound: Intima-Media Thickness, Plaque Area, and Three-Dimensional Phenotypes. In: Nicolaides A, Beach KW, Kyriacou E, et al. editors. Ultrasound and Carotid Bifurcation Atherosclerosis. London: Springer-Verlag, 2011:431-48.
- Johri AM, Hétu MF, Nambi V. Carotid Plaque or CIMT: What is the Future for Carotid US Imaging? Curr Cardiovasc Risk Rep 2014;8:1-8. [Crossref]