Pulmonary static inflation with 50% xenon attenuates decline in tissue factor in patients undergoing Stanford type A acute aortic dissection repair
Introduction
Stanford type A acute aortic dissection (AAD) carries a high risk of mortality and morbidity and may require surgical correction to avoid cardiac tamponade and sudden aortic rupture (1,2). Often, patients undergoing surgery for AAD bleed excessively and require blood products and transfusions (3-5). Thus, early diagnosis and coagulation correction may improve patient prognosis. Recently, volatile anesthetics have been studied for their negative effects on platelet aggregation (6,7). Xenon as an inhaled anesthetic is under study, and animal and human data suggest that it has a lack of organ toxicity, allows for rapid recovery, and has cardiovascular-stabilizing effects (8-11). Abraini’s group (12) reported that xenon inhibits tissue plasminogen activator (tPA) using molecular modeling and in vitro and in vivo studies. de Rossi’s group (13) reported that xenon did not affect unstimulated or agonist-induced human platelet function in vitro, and Horn and his team (14) reported that xenon monoanesthesia did not cause pathological alterations in coagulation factors in healthy pigs. The effect of xenon on physiopathology and mechanisms of AAD and surgery-induced coagulopathy have not been studied preoperatively or during and after surgery. Thromboelastography (TEG) (15) is commonly used to assess whole blood coagulation and to provide information about platelet function and fibrinolysis. Thus, we studied the effects of xenon on coagulation using TEG and conventional hemostatic tests for patients with AAD undergoing aortic arch surgery with cardiopulmonary bypass (CPB)/deep hypothermic circulatory arrest (DHCA).
Methods
Study design
We studied perioperative clinical variables and serological results from 50 subjects who underwent pulmonary static inflation with 50% nitrogen/50% oxygen from January 2013 to January 2014 and 50 subjects who underwent pulmonary static inflation with 50% xenon/50% oxygen from January 2014 to December 2014 during CPB for Stanford type A AAD in a single center clinical trial. Some data came from previously published statistics (11). Investigators were not blinded to experimental groups but patients and technicians who performed assays were blinded to groups. The clinical trial was registered in the Chinese Clinical Trial Registry (ChiCTR-ICR-15006435) and approved by the Beijing Anzhen Hospital Clinical Research Ethics Committee (identifier: 2013027). A diagnosis of AAD was confirmed for all patients by history, chest radiography, transthoracic ultrasound, and contrast-enhanced computed tomography (CT) or magnetic resonance imaging (MRI). Dissection was considered AAD if the time from the onset of symptoms to surgery was 14 days or less. This study only included type A AAD according to the Stanford classification (2,16). Medical quality 50% xenon was provided by Wuhan Newradar Special Gas Co. LTD (Wuhan, China).
Subjects
Patients, aged 18–70 years-of-age, with acute type A AAD undergoing emergency surgery with CPB/DHCA in our hospital were enrolled. Exclusion criteria were coronary heart disease; heart failure; severe cardiac tamponade; unstable hemodynamics; nervous system abnormalities; clinically apparent malperfusion (17) including severe malperfusion in the lower limb, brain, heart, and kidney; visceral ischemia; and lack of written informed consent. In brief, all patients were class Aa according to the Penn Classification (18), which is the absence of branch vessel malperfusion or circulatory collapse. No patient was prescribed nonsteroidal anti-inflammatory drugs or corticosteroids before or after admission. After written informed consent, 100 patients were blinded to receiving either 50% xenon/50% oxygen (xenon group, n=50) or 50% nitrogen/50% oxygen (control group, n=50) during CPB.
Trial procedure
Demographic and hemodynamic data were recorded immediately after admission. To avoid aortic rupture, systolic blood pressure was controlled between 100 and 120 mmHg with oral β-blockers and infusion of vasodilators (e.g., nitroprusside) before surgery. In all cases, total intravenous anesthesia was performed with sufentanil and propofol, and BIS was maintained at 45–55. After CPB, the aorta was cross-clamped and mechanical ventilation was stopped, lungs were inflated with 50% xenon/50% oxygen (xenon group) or 50% nitrogen/50% O2 (control group) to maintain an airway pressure of 5 cmH2O. Pulmonary static inflation with 50% xenon or 50% O2 was stopped 15 min before aortic de-clamping and cardiac resuscitation. After CPB, tranexamic acid, lyophilized human fibrinogen and lyophilized human prothrombin complex concentrate were used to improve coagulation according to TEG and conventional hemostatic tests. Blood products were transfused to maintain a post-CPB Hb >7 g/L and to correct coagulopathy after normalizing TEG with fresh frozen plasma and platelets. Coagulation and fibrinolysis were measured prior to surgery and 10 min and 6 hours postoperatively.
Endpoint
The primary endpoint was to assess the perioperative changes in coagulation and fibrinolysis after intubation and 10 minutes, and 6 hours after the operation. The secondary endpoint was to assess the perioperative changes in serum level of tissue factor (TF), tissue factor pathway inhibitor (TFPI) and tPA after intubation and 10 minutes, and 6 hours after the operation.
Cardiac operation
Surgical procedures described by Sun were used (19,20). In brief, all patients underwent CPB with DHCA and unilateral selective antegrade cerebral perfusion (USACP). When the nasopharyngeal temperature reached 20–25 °C, circulatory arrest was established and a stent graft was implanted. Then, a four-branch prosthetic graft was used for arch replacement. Valve-sparing root resection (aortic valve plasty, Valsalva sinus plasty, or the David procedure) or composite valve graft replacement was performed according to the subtype of the aortic root (A1, A2, or A3).
Blood samples
Venous blood (10 mL) was drawn from the central vein catheter immediately after intubation, and 10 min and 6 hours after surgery. After rapid centrifugation, plasma was obtained and immediately stored at −70 °C for analysis. Prothrombin time (PT), PTA, activated partial thromboplastin time (APTT), median fibrinogen degradation product (FDP), international normalized ratio (INR), fibrinogen, D-dimer, leucocytes, hemoglobin, hematocrit and platelet counts were measured using standard assay techniques and an automated blood coagulation analyzer. TEG-R, TEG-K, TEG-angle and TEG-MA were assayed with a hemostatic analyzer (TEG 5000 hemostasis analyzer, Haemoscope Corporation, CITY MISSING, IL, USA). TF, TFPI, and tPA were assayed using an ELISA (Multiskan MK3, Thermo Fisher Scientific, CITY MISSING, MA, USA).
Statistical analysis
The sample size was selected to detect differences in perioperative TF values between the two groups. Sample allocation ratio was 1, and time- points were 3, and test for two means in a repeated measures design was used. With a 5% (two-sided) type I error rate, based on preliminary experiments, 50 patients per treatment group were needed to detect a 0.8 difference between two groups, assuming a standardized deviation of 1.5. The power was from 90–93% as an autocorrelation from 0.4 to 0.5 accordingly. The final sample size was 100 patients (50 patients/group) (Power Analysis and Sample Size, PASS v11.0, NCSS, LLC; Kaysville, UT, USA).
All data were analyzed using a commercially available statistical software package (SPSS for Windows, v18.0; Chicago, IL, USA). Quantitative variables are presented as means ± SD or medians (interquartile range) and categorical variables were presented as frequencies or percent. Normally distributed continued variables were compared using a two-tailed Student’s t-test. When parametric data were not normally distributed, Wilcoxon signed rank sum tests were used for intergroup comparisons. Categorical data were compared using the Pearson’s chi-squared test or Fisher’s exact test, as appropriate. Coagulation and fibrinolysis were analyzed using generalize additive mixed model (GAMM), which grouped as treatments or controls and time was a within-subject factor. Nonparametric and parametric tests were applied to demographics [age, sex, body mass index (BMI), smoking history, hypertension, diabetes], clinical (hemodynamics, onset of symptoms to surgery, aortic diameter, left ventricular ejection fraction (LVEF), left ventricular end diastolic diameter (LVEDd), EuroSCORE], surgical (surgery time, duration of surgery, intravenous colloid and crystalloid and transfusion), and postoperative variables [intravenous fluids (colloid or crystalloid), blood transfusions, urine volume, seroma drainage, in-hospital mortality]; P<0.05 were considered statistically significant.
Results
All patients underwent total arch replacement combined with frozen elephant trunk implantation. A modified Bentall procedure was performed in 54 patients with severe aorticre gurgitation. Demographics and preoperative clinical characteristics for subjects are presented in Table 1. Preoperative platelet counts below 100×109/L was found in 9% (9/100) AAD patients, and preoperative Hb below 10 g/L was found in 2% (2/100) AAD patients. The PT (>15 s) and APTT (>40 s) were prolonged in 3% (3/100) and 4% (4/100) patients, respectively. Hypofibrinogenemia (<2 g/L) was noticed in 5% (5/100) of patients. TEG-reaction time (R) (>10 min) and TEG-clot kinetic (k) time (>3 min) was prolonged in 2% (2/100) patients and in 6% (6/100) patients, respectively. TEG-alpha angle (<50°) was found to be decreased in 5% (5/100) patients. At the end of surgery, PT (>15 s) and APTT (>40 s) were prolonged in 3% (3/100) and 15% (15/100) patients, respectively; hypofibrinogenemia (<2 g/L) was noticed in 13% (13/100) of patients. TEG-reaction time (R) (>10 min) and TEG-clot kinetic (k) time (>3 min) was prolonged in 9% (9/100) patients and in 9% (9/100) patients, respectively; TEG-alpha angle (<50°) was found to be decreased in 11% (11/100) patients. Coagulation and fibrinolysis were noted between groups and TEG offered similar data for all patients. Median TEG-R values in the xenon group were greater than for controls prior to surgery.
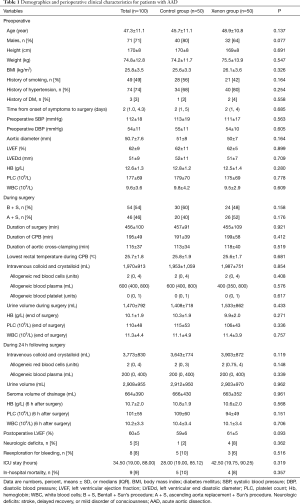
Full table
Perioperative coagulation and fibrinolysis data appear in Table 2. PT, APTT, INR, FDP, D-dimer increased and peaked at the end of surgery and then decreased until 6 hours after surgery. TEG also revealed similar change of perioperative coagulation and fibrinolysis system profile. Median TEG-R increased and peaked at the end of surgery and then decreased until 6 hours after surgery. Median TEG-K increased until 6 hours after surgery, and TEG-angle and TEG-MA were decreased until that time. TEG values did not differ between groups at the end of surgery. There was median maximal increase in TEG-R of 22% and 45% in the xenon and control groups at the end of surgery respectively. However, there was no significant of treatment-time effects for coagulation and fibrinolysis between two groups.
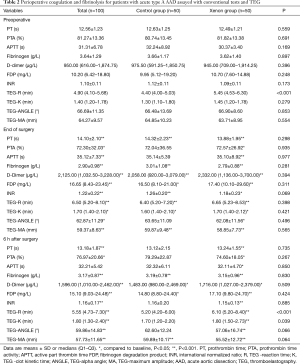
Full table
Perioperative coagulation and fibrinolysis for patients with AAD was accompanied with TF depression and decreased tPA at end of surgery and 6 hours later. These data appear in Table 3. Figure 1 depicts TF changes from preoperative to postoperative 6 hours time points and xenon had a significant effect on TF. From preoperative to end of surgery and from preoperative to postoperative 6 hours, the mean level of TF decreased significantly in control group (β −2.61, P<0.001 and β −2.83, P<0.001, respectively), but was maintained relatively stable in xenon group (β −0.5, P<0.001 and β −0.96, P<0.001, respectively).
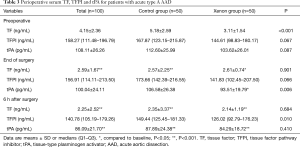
Full table
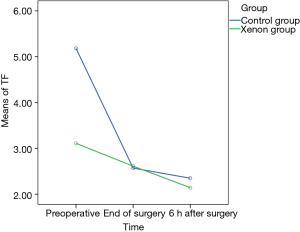
Discussion
In present study, we noted an inhibition of anticoagulation and activation of fibrinolysis during the perioperative period for AAD patients. A few other reports have focused their attention to this topic (3-5,21), but none of them described the state of AAD patients using TEG and conventional hemostatic tests before and immediately after the emergent operation. In fact, AAD itself is associated with a high risk for coagulation disorders before surgery. In our study, preoperative values of TEG and conventional hemostatic tests showed a certain level of coagulation disorders. After surgery, there existed the consumption of clotting factors as well as the further activation of coagulation and fibrinolysis system, which was accompanied with TF depression and decreased tPA at end of surgery and 6 hours later. Thus, at the end of surgery, coagulation disorders tended to worsen with course of surgery.
AAD is characterized by the rapid development of an intimal flap separating the true and false lumen (16), blood flow through the nonendothelialized false lumen, tissue damage, and turbulence, which are all triggers for coagulation activation (22). During surgery, CPB-induced coagulopathy, platelet activation and dysfunction and promoted coagulation factor consumption and excessive fibrinolysis, increasing postoperative blood loss and blood product transfusion. After surgery, deterioration of coagulation function and active fibrinolysis was confirmed by conventional tests and TEG analysis. We noted that TF was lower at the end of surgery and 6 hours post-surgery, tPA was also diminished 6 hours after surgery, and D-dimer and FDP was evaluated at the end of surgery in AAD patients. Thus, coagulopathy and excessive fibrinolysis is associated with postoperative bleeding in AAD patients.
Recently, volatile anesthetics have been studied for their effects on coagulation, especially platelet aggregation. Wacker‘s group reported that inhaling low-dose sevoflurane (<1 vol% end-tidal) delayed agonist-induced granulocyte-platelet interactions in humans (7). Horn’s group found that sevoflurane enhanced binding of platelets to lymphocytes, neutrophils and monocytes, and increased expression of P-selectin on platelets, especially in stimulated samples, but desflurane decreased lymphocyte-, neutrophil- and monocyte-platelet conjugates in unstimulated samples (6). Yuki and colleagues reported that isoflurane and sevoflurane reduced activation of the platelet receptor integrin αIIbβ3 (23). Isoflurane also blocked the platelet ADP pathway (24).
Xenon anesthesia is associated with more stable intraoperative blood pressure, lower heart rate, faster emergence and faster recovery from anesthesia than volatile and propofol anesthesia (8,9). Previous studies document that xenon did not affect platelet function in vitro but inhibited tPA (12-14). In vitro (13), whole blood was collected from healthy volunteers and was incubated with 65% xenon did not alter unstimulated or agonist-induced platelet glycoprotein expression or platelet-related hemostasis function. In vivo (14), 20 healthy pigs underwent xenon monoanaesthesia (65 vol%), and xenon monoanaesthesia did not alter significantly any coagulation parameter. While xenon is an inhibitor of tPA activator in a rat model of thromboembolic stroke (12). In our study, pulmonary static inflation with 50% xenon during CPB halted decreases in TF and also inhibited tPA at the end of surgery, which may attenuate increase in TEG-R after surgery for patients undergoing AAD repair.
Although the above-described changes were statistically significant, none of the clinical parameters such as blood transfusion or duration of surgery were significant between groups. Such condition might due to correct coagulopathy after normalizing TEG with fresh frozen plasma, platelets and hemostatics (tranexamic acid, lyophilized human fibrinogen and lyophilized human prothrombin complex concentrate).
Study limitations
First, our study was limited by being non-randomized, so the data are biased. Thus, there were differences in the patient groups with respect to the levels of TF in the treatment over control group at baseline. However, such differences in baseline did not affect the trends of perioperative level of TF. Second, xenon was given the period of CPB, which was short, and any potential advantages of xenon with respect to blood coagulation and fibrinolysis may have been masked during surgery or with post-operative interventions. Third, the xenon was applied statically to the lungs during CPB in which blood flow is specifically diverted from the pulmonary circulation, and we could not explain how xenon diffuse into the systemic circulation to affect tPA/TF. Previously report that might partly account for xenon effect on tPA/TF by triggering anti-inflammatory responses and suppressing proinflammatory and oxidative effects (11). Finally, Stanford type A AAD demonstrated the similar volume of aortic dissection, however we were not able to obtain highly detailed information to evaluate the volume of false lumen and thrombogenesis which significantly affected coagulation results.
Conclusions
Deterioration of coagulation function and activated fibrinolysis was confirmed by conventional tests and TEG analysis after Stanford type A AAD repair. Pulmonary static inflation with 50% xenon attenuates decline in tissue factor in patients undergoing Stanford type A AAD repair.
Acknowledgements
We thank Accdon (www.accdon.com) for linguistic assistance during the preparation of this manuscript.
Funding: This study was supported by grants from the Beijing Municipal Science & Technology Commission (Z151100004015133, Z161100000513067, and Z171100001017083) and the National Science and Technology Support Program of China (2015BAI12B03). Sponsors had no influence on the design, analysis, or publication of this study.
Footnote
Conflicts of Interest: The authors have no conflicts of interest to declare.
Ethical Statement: The study was approved by the Beijing Anzhen Hospital Clinical Research Ethics Committee (identifier: 2013027) and written informed consent was obtained from all patients.
References
- Hagan PG, Nienaber CA, Isselbacher EM, et al. The International Registry of Acute Aortic Dissection (IRAD): new insights into an old disease. JAMA 2000;283:897-903. [Crossref] [PubMed]
- Golledge J, Eagle KA. Acute aortic dissection. Lancet 2008;372:55-66. [Crossref] [PubMed]
- Paparella D, Rotunno C, Guida P, et al. Hemostasis alterations in patients with acute aortic dissection. Ann Thorac Surg 2011;91:1364-9. [Crossref] [PubMed]
- Guan XL, Wang XL, Liu YY, et al. Changes in the Hemostatic System of Patients With Acute Aortic Dissection Undergoing Aortic Arch Surgery. Ann Thorac Surg 2016;101:945-51. [Crossref] [PubMed]
- Liu Y, Han L, Li J, et al. Consumption coagulopathy in acute aortic dissection: principles of management. J Cardiothorac Surg 2017;12:50. [Crossref] [PubMed]
- Horn NA, de Rossi L, Robitzsch T, et al. The effects of sevoflurane and desflurane in vitro on platelet-leukocyte adhesion in whole blood. Anaesthesia 2003;58:312-9. [Crossref] [PubMed]
- Wacker J, Lucchinetti E, Jamnicki M, et al. Delayed inhibition of agonist-induced granulocyte-platelet aggregation after low-dose sevoflurane inhalation in humans. Anesth Analg 2008;106:1749-58. [Crossref] [PubMed]
- Law LS, Lo EA, Gan TJ. Xenon Anesthesia: A Systematic Review and Meta-Analysis of Randomized Controlled Trials. Anesth Analg 2016;122:678-97. [Crossref] [PubMed]
- Hou B, Li F, Ou S, et al. Comparison of recovery parameters for xenon versus other inhalation anesthetics: systematic review and meta-analysis. J Clin Anesth 2016;29:65-74. [Crossref] [PubMed]
- Winkler DA, Thornton A, Farjot G, et al. The diverse biological properties of the chemically inert noble gases. Pharmacol Ther 2016;160:44-64. [Crossref] [PubMed]
- Jin M, Yang Y, Pan X, et al. Effects of pulmonary static inflation with 50% xenon on oxygen impairment during cardiopulmonary bypass for stanford type A acute aortic dissection: A pilot study. Medicine (Baltimore) 2017;96. [Crossref] [PubMed]
- David HN, Haelewyn B, Risso JJ, et al. Xenon is an inhibitor of tissue-plasminogen activator: adverse and beneficial effects in a rat model of thromboembolic stroke. J Cereb Blood Flow Metab 2010;30:718-28. [Crossref] [PubMed]
- de Rossi LW, Horn NA, Baumert JH, et al. Xenon does not affect human platelet function in vitro. Anesth Analg 2001;93:635-40. [Crossref] [PubMed]
- Horn NA, Hecker KE, Bongers B, et al. Coagulation assessment in healthy pigs undergoing single xenon anaesthesia and combinations with isoflurane and sevoflurane. Acta Anaesthesiol Scand 2001;45:634-8. [Crossref] [PubMed]
- Wikkelsø A, Wetterslev J, Møller AM, et al. Thromboelastography (TEG) or thromboelastometry (ROTEM) to monitor haemostatic treatment versus usual care in adults or children with bleeding. Cochrane Database Syst Rev 2016. [PubMed]
- Nienaber CA, Eagle KA. Aortic dissection: new frontiers in diagnosis and management: Part I: from etiology to diagnostic strategies. Circulation 2003;108:628-35. [Crossref] [PubMed]
- Yan TD, Tian DH, Lemaire SA, et al. Standardizing clinical end points in aortic arch surgery: a consensus statement from the International Aortic Arch Surgery Study Group. Circulation 2014;129:1610-6. [Crossref] [PubMed]
- Kimura N, Ohnuma T, Itoh S, et al. Utility of the Penn classification in predicting outcomes of surgery for acute type a aortic dissection. Am J Cardiol 2014;113:724-30. [Crossref] [PubMed]
- Sun L, Qi R, Zhu J, et al. Total arch replacement combined with stented elephant trunk implantation: a new “standard” therapy for type a dissection involving repair of the aortic arch? Circulation 2011;123:971-8. [Crossref] [PubMed]
- Sun L, Qi R, Zhu J, et al. Repair of acute type A dissection: our experiences and results. Ann Thorac Surg 2011;91:1147-52. [Crossref] [PubMed]
- Guan X, Li J, Gong M, et al. The hemostatic disturbance in patients with acute aortic dissection: A prospective observational study. Medicine (Baltimore) 2016;95. [Crossref] [PubMed]
- Idell S. Coagulation, fibrinolysis, and fibrin deposition in acute lung injury. Crit Care Med 2003;31:S213-20. [Crossref] [PubMed]
- Yuki K, Bu W, Shimaoka M, et al. Volatile anesthetics, not intravenous anesthetic propofol bind to and attenuate the activation of platelet receptor integrin alphaIIbbeta3. PLoS One 2013;8. [Crossref] [PubMed]
- Harr JN, Moore EE, Stringham J, et al. Isoflurane prevents acute lung injury through ADP-mediated platelet inhibition. Surgery 2012;152:270-6. [Crossref] [PubMed]