Mechanisms of tumor immunotherapy, with a focus on thoracic cancers
The basics of tumor immunology: learning from melanoma patients
Tumor immunology is a familiar concept for current oncologists, but demonstrating that cancer could be controlled by boosting inner immune defenses has been taking several decades of intense and hard research at preclinical and clinical setting. The process has fortunately allowed to collect a large amount of scientific and clinical evidence supporting the active role of immunity in human cancer, and to discover the therapeutic targets that we are presently using or testing in most solid cancers. As above mentioned, the trail has been traced in melanoma and permitted to gain pillar knowledge on the rules regulating tumor/immune interaction in human cancer (Figure 1).
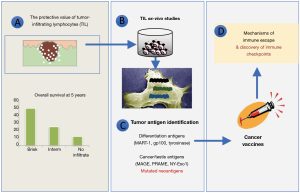
The role of tumor immune infiltrate
The first evidence about tumor immunogenicity in human setting stems from the observation about the presence of lymphocytes infiltrating primary melanoma skin lesions. These cells, sometimes showing tight interaction with cancer cells and entering deep into tumor nests (the so called “brisk” infiltrate), were noted to associate with better disease course and thus represent a positive prognostic factor in a pivotal study performed at our Center by Clemente et al. in the early 1990s (1). This evidence was followed by parallel multiple studies in melanoma and many other tumor histotypes (1,2), further characterizing infiltrating immune cells as CD3+ T lymphocytes, often of the cytolytic CD8+ subset, although the presence of natural killer (NK) or B cells was also reported in some instances but with less prognostic implications (3-7).
This local pre-existing adaptive antitumor immunity substantially indicates that immune responses occur spontaneously in cancer-bearing host; these responses slow down the disease and have generally a protective effect [with the only exception of renal cell carcinoma (RCC) and possibly luminal breast cancer (BCa)] (8-10); therapeutic approaches aimed at boosting this pre-existing immunity could contribute to further improve tumor control; and not all patients mount immune responses suggesting strong individual variabilities.
These immunohistochemical pioneering studies have been recently echoed by pivotal immunogenomics analyses on thousands of human tumors encompassing multiple cancer types, showing that specific immune response patterns have prognostic implications (11), confirming the key role of immunity in cancer.
Nature of tumor antigens
The discovery of IL-2 as major T cell growth factor, has allowed in the 1990s to isolate and expand ex-vivo tumor infiltrating lymphocytes (TIL), for functional and phenotypic characterization (12). This second key phase witnessed the discovery that TIL were actually in a blocked functional state (T cell anergy) (13) but that they could be reactivated in vitro by the culture with stimulatory factors (IL-2) and reacquire the ability to recognize and kill tumor cells (14). This discovery paved the way to the use of TIL, expanded in the laboratory at quite higher numbers (billions), for adoptive T cell transfer in advanced melanoma patients, a strategy pursued for decades by Steve Rosenberg’s team (15) with sometimes impressive clinical successes. Besides being the precursor to other more sophisticated forms of adoptive T cell therapy recently entering clinical practice for hematological disease [e.g., chimeric antigen receptor (CAR)-T cells] (16) and the proof of principle about the true antitumor nature of TIL, this approach provided tools for the identification and the study of antigenic determinants expressed by tumor cells and recognized by TIL as cancer antigens (17,18). A “gold rush” to discover these molecules in melanoma but also in other cancers through the screening of cDNA libraries allowed to identify a huge number of proteins that, processed and presented in the context of human leukocyte antigen (HLA) molecules, could be recognized by autologous TIL. Depending on the patterns of T cell tumor recognition that could involve multiple cancers of different or same histologies or be restricted to the autologous tumor, antigens were divided into shared and unique antigens. The former group includes molecules specific of a defined tissue (melanin-related antigens for melanoma, such as Mart-1 and gp100, or epithelial-related antigens like EpCam or Her2 for carcinomas) recognized by T cells because of the over-expression (19-21), or proteins typical of embryonic phases that are ectopically reactivated in cancer cells, like the antigens of the cancer-testis family (NY-Eso1, Mage and Prame), or finally viral proteins encoded by oncogenic viruses like HPV (17). Unique antigens are instead proteins expressed individually in the tumor of a specific patient that become antigenic because of the presence of mutations with respect to the parental protein expressed in normal cells. TIL recognizing these antigens are rather abundant within the TIL population because of the strong immunogenicity that a mutated protein, behaving as a “non-self” foreign target, exerts on our immune system. Some of these mutated antigens play also a role in the neoplastic process involving key pathways in cell growth regulation.
How to boost spontaneous tumor immunity: the use of cancer vaccines
One of the well-established tools to stimulate specific immunity is based on the concept of vaccines, meaning that the administration of antigenic determinants in the right immunological context should boost protective immunity. Despite this approach is developed to prevent infectious diseases and not for being used in therapeutic setting, the availability of shared tumor antigens and the significant tumor control that could be achieved in murine models, promoted an intense investigation on how to boost spontaneous antitumor immunity by specific vaccines. Multiple clinical trials testing diverse antigenic forms (from whole proteins to HLA binding peptides) with different types of immunological adjuvants, were assessed in phase I–II clinical trials mostly involving patients with advanced melanoma and prostate carcinoma. Significant immune responses, detected in peripheral blood as increase in the frequency and function of T cells specific for the vaccine antigens, could be observed albeit clinical efficacy was limited to scattered responses. However, it should be mentioned that in the few responding patients therapeutic benefit was often long-term, indicating the possible onset of immune memory and anticipating a hallmark of immune-mediated tumor control.
We now know that the reasons underlying the limited clinical efficacy of cancer vaccines are multiple and complex, as discussed in details elsewhere (22), and partially involve the same regulatory mechanisms that we are targeting with the present strategies of immunotherapy. Nevertheless, even in the present era of immune checkpoint inhibitors (ICI), the ability of cancer vaccines can increase the frequency of antigen-specific T cells in tumor site and could be exploited for cancers lacking spontaneous immunogenicity. This explains the efforts still ongoing on several fronts to develop and evaluate new strategies for improving the efficacy of cancer vaccines in synergy with novel immunotherapeutics.
The discovery of tumor immune escape
The coexistence of a growing tumor with a spontaneous or induced antitumor immune response opened a third phase in the tumor immunology research aimed at finding explanations for this intriguing paradox and for the failure of cancer vaccines. In studies performed mostly in metastatic melanoma patients, cancer cells present at tumor site were found to evade immune system by downmodulating the determinants required for T cell recognition, for instance HLA molecules or tumor antigens, or by secreting immunosuppressive factors halting T cell function (23). However, a decade of research on tumor immune escape has clearly revealed that the far most predominant mechanism of immune escape involves pathways physiologically devoted to the control of immune homeostasis during chronic stimulation. Indeed, with respect to normal tissue, tumor microenvironment (TME) is featured by: the upregulation of specific immunological receptors known as immune checkpoints on tumor, stromal and infiltrating immune cells, and the accrual of immunosuppressive and regulatory effectors associated with chronic inflammation. Both these pathways contribute to block T cell proliferation and function, thus allowing tumor cells to survive and progress in vivo.
T cell exhaustion and the role of immune checkpoints
The high burden and the rich repertoire of antigenic determinants expressed by certain tumors represent a strong stimulus for specific T cell priming, that may chronically persist in case the tumor is not eliminated. With time this process produces a T cell overstimulation with progressive loss of antitumor function and the onset of a well-defined immune status known as exhaustion. Exhausted T cells do not proliferate, secrete cytokines or kill target cells and enter a sort of functional paralysis featured by low glucose consumption. Fortunately, this state is reversible, meaning that T cells can regain activity if removed from the exhausting environment. A proof of this process is the evidence that TIL do not exert any antitumor activity when extracted from the tumor lesion but they reacquire antitumor activity when cultured ex-vivo in stimulating conditions.
T cell exhaustion is associated with a well-defined molecular pattern, that includes a relatively novel family of receptors defined immune checkpoints. Discovered in the early 1990s as molecules involved in T cell growth control and autoimmunity, immune checkpoints such as PD-1 and CTLA-4 have been then found to play a role in feto-maternal tolerance, chronic viral infections and transplantation (24). Evidence about the expression of these immune checkpoints in TME was then reported, indicating that tumor cells could exploit them to promote immune escape by inducing T cell exhaustion (25).
Reactivating effective antitumor immunity
The discovery that TIL expressed higher levels of CTLA-4 and PD-1, along with the presence of the cognate ligand PD-L1 expressed by tumor cells, opened a totally new era of cancer immunotherapy based on inhibiting the negative signals delivered by immune checkpoints (11,26). The production of human antagonist antibodies binding to these molecules with higher affinity than the natural counterparts, provided with a tool to effectively reactivate tumor-specific T cells in vivo in cancer patients, and possibly regain immune-mediated tumor control. Indeed, tumor lesions biopsied during treatment with ICI, display a higher TIL frequency with respect to baseline lesion, the induction of proliferative and activation markers, and gene expression signatures compatible with acute and full-fledged immune responses (27). Despite the subsets of T cells predominantly responding may depend on the type of ICI (with CD8+ early effector and effector memory cytotoxic T cells for PD-1/PD-L1 axis, and ICOS+Th1+CD4+ helper T cells for CTLA-4) (28), the final effect is a switch-on of the immune microenvironment that eventually involves the concerted activation of diverse infiltrating leukocytes in a sort of bystander effect. Signs of a rebalance of immune cell subsets, together with a raise in T cells specific for defined tumor antigenic determinants, can also be detected in peripheral blood and draining lymph nodes, in support of the systemic nature of cancer immunity (29).
The clinical testing performed in melanoma patients provided revolutionary results in the treatment of this cancer, soon fostering the translation of this therapeutic strategies to other cancer histologies including non-small cell lung cancer (NSCLC). In such a setting, immunological features of lung cancer were rapidly investigated in parallel with clinical trials, in order to strengthen the rational of using immunomodulation for controlling lung cancer and developing potential predictive biomarkers for patients’ selection.
Spontaneous immunity in NSCLC
The accessibility of tumor lesions, together with the feasibility in obtaining TIL and stable tumor cell lines for in vitro studies, have consented to directly characterize the phenotypic and functional features of melanoma-associated T cells, along with assessing their antigenic specificity and true antitumor activity. These complex and elegant data stemmed from emerging techniques of DNA libraries/antigen cloning (17) and paralleled by novel methodologies of harmonized immunomonitoring (30), shed the bases of our current view of tumor immunology and the knowledge about cancer/immune cross-talk.
Tumor immune infiltrate as a window to patient’s immunity
Despite the evidence of the prognostic role of TIL emerged in melanoma in the 1990s (1), and the immunoscore formulated in the same years in colon cancer (31), convincing evidence about a prognostic role of T cell infiltrate in lung cancer was provided only few years ago in the era of ICI (32). Counting of total CD8+ T cells, and not of other immune cell subsets including CD20+ B cells or CD4+ helper lymphocytes, was found to be associated with a higher survival rate in terms of overall survival (OS) and progression-free survival (PFS) (32), with studies by other teams validating the evidence independently (33,34) Importantly, lymphocytes defined by just H&E morphology, as applied originally in melanoma, were not adequate to detect a protective immunity, indicating the relevance of selective cell subsets in controlling tumor growth. More detailed studies also indicated that T cell infiltrate density at the stroma-tumor interface is predictive of a better outcome in NSCLC (34), as lymphocytes present within the tumor nests are scantly. Such an evidence suggests that, at difference with melanoma whose prognostic immune infiltrate has to be “brisk”, antigen-specific T cells in NSCLC might encounter a more hostile milieu impeding them to enter into the core of lesion at baseline conditions.
More recent studies addressing in detail the phenotypic features of NSCLC immune infiltrate depicted a complex scenario, with enrichment of clonal CD8+ T cells, regulatory T cells (Treg) and B cells, and decrease of NK and NKT cell subsets, with respect to surrounding non-tumor lung tissue.
Tumor antigens triggering T cell activation
Unexpectedly, with respect to the previous dogma that only melanoma and renal carcinoma were immunogenic tumors in human setting, specific studies unraveled that the NSCLC expresses a large array of antigens recognized by T cells. In terms of neoantigens, NSCLC is among the top cancers expressing non-synonymous mutations and frameshift alterations giving rise to strong-binder neoantigens. Mutated proteins can be also generated from insertion-deletion DNA alterations (35) although these modifications are less frequent in NSCLC. Despite these are considered the most relevant antigens in mediating clinically effective antitumor immunity (36), it should be underlined that NSCLC is among the top expressors of cancer-testis antigens, a family of germ cell-antigens, which are not expressed in normal cells but are reactivated in cancer. Both adenocarcinoma and squamous NSCLC express high levels of these antigens (MAGEC2, MAGEB6, PAGE2, CT45A2, SAGE1 and MAGE8) (37,38), which contain both T cell and antibody epitopes and may also generate neoantigens through non-synonymous point mutations (37). An additional promising feature of this antigenic family from an immunotherapy point-of-view is that their expression is mostly regulated by methylation, hence it could be raised by demethylating agents (37), as it might possibly occur in patients treated with azacytidine. Multiple are also the surface tumor-associated antigens expressed by NSCLC, that could be target of CAR-T cell therapy. CEA, EGFR, GD2, and Muc1 are only some of the membrane proteins recognizable by high affinity monoclonal antibodies (mAbs) presently being studied for the synthesis of chimeric receptors able to provide T cells with the ability to recognize NSCLC for therapeutic application in clinical setting (18).
Deeper into NSCLC immune infiltrate: biomarkers of antitumor and protumor immunity
More comprehensive approaches for the analysis of the TME have recently revealed the complexity and the heterogeneity of the immune response that patients develop toward their own cancer cells. Recently, high through-put approaches for the analysis of tumor immune microenvironment have been applied to NSCLC to gain insights into the immunological features of this cancer and their prognostic implications. TIL isolated from highly infiltrated lesions were found to display a strongly activated gene-expression signature, expressing anti-tumor cytolytic functions, together with signalling pathways leading to the release of acute inflammation-related cytokines, chemokines and related receptors (including IFN-γ, CXCR6, CCL4) capable of sustaining effective immune responses (39). As such, and because of the chronic stimulation caused by highly immunogenic tumor, the upregulation of a broad panel of negative (CTLA-4, PD-1, LAG3, TIM3) and positive (4-1BB, CD40, OX40) immune checkpoints is usually detected in T cells from highly with respect to poorly infiltrated tumors, to indicate the onset of immune escape mechanisms consenting tumor growth despite immune responses. Interestingly, a subset of lesions was found to be enriched in a specific CD8+ T cell subtype expressing the integrin CD103, along with a strong effector signature (IFN-γ, granzyme B, STAT1, RAB27, CCL4, CD107a, CD38, NOTCH and OX40) and representing a resident memory immune cell subset (39). The frequency of these cells within tumor lesions is strongly associated with a better prognosis in terms of PFS and OS, suggesting their highly protective role in lung cancer control.
An active antitumor immunity promotes in vivo tumor immunoediting and escape
Pillar studies in murine models have proved the existence of protective immunological mechanisms intercepting cancer cells on the basis of antigenic features and eliminating them mainly through the involvement of adaptive immunity. Similar to what occurs with viral infections, a mechanism of immune surveillance relying on the activation of antigen-specific T cells is believed to survey each tissue for minimizing the survival of aberrant cells (40). The increased incidence of solid cancers in chronically immunodepressed patients (41) and the positive prognostic impact that immune infiltrate plays in most solid cancers, support the evidence that immunosurveillance does exist in human setting as well.
Preclinical studies, supported by recent data from cancer patients treated with immunotherapy, show that immunosurveillance is indeed the leading force promoting an evolutionary process that can sculpt tumor phenotype and functions. The process, known as “immunoediting”, causes substantially the selection of tumor cell clones able to survive immune recognition, through the loss of antigenic determinants (HLA or antigens) expressed by tumor cells or the upregulation of immune suppressive pathways aimed at switching-off T cell function (42).
Immune checkpoints are a hallmark of infiltrated/hot tumors
Detailed studies on cellular and molecular characterization of NSCLC TME have recently unraveled that tumor lesions can be divided in “hot” or “cold” tumors on the basis of a pre-existing T cell infiltrate (43). For reasons likely related to the level of the antigenic repertoire expressed by cancer cells, tumors might appear as heavily (hot) or poorly infiltrated (cold) by T cells (Figure 2) (44). Interestingly, the more infiltrate is present the higher is the expression both in immune and tumor cells of pathways involved in controlling immune homeostasis, i.e., the receptors of the immune checkpoint family. PD-1 as well as CTLA-4, LAG3 and TIM3 are highly upregulated in the T cells present in hot tumors, to indicate that the triggering of a full-fledged immune response is unavoidably associated with the upregulation of intrinsic pathways of immune exhaustion (39). Concomitantly, and possibly because of the IFN-γ release mediated by activated T cells, tumors upregulate the corresponding ligands (starting from PD-L1) thus contributing to the functional switch-off of antitumor immunity (45). This evidence explains why high PD-L1 expression is often associated with better clinical efficacy of PD-1 blockade, being indeed the result of a more T cell infiltrated TME and stronger pre-existing immune response. An interesting emerging issue is the role that the tumor genotype may play in influencing this immune contexture. Indeed, through the release of diverse cytokine and chemokine networks, a tumor can finely tune the composition of its own immunological milieu and, as a consequence, profoundly influence immunotherapy outcome (45). For instance, EGFR and KRAS mutations are featured by limited T cell infiltration and a cold un-inflamed TME, fully justifying the poor clinical benefit of immunotherapy in such a patient setting (46).
Local and systemic strategies of immune escape in NSCLC
A pillar study performed in melanoma provided a rather comprehensive picture about the microenvironmental features of non-responding tumors (47), a scenario that is emerging as possibly being a constant of cold cancers. Along with the paucity of infiltrating T cells, solid lesions share an enrichment of myeloid cells in diverse differentiation stages [immature myeloid cells, myeloid-derived suppressor cells (MDSC), macrophages and neutrophils] and related immunosuppressive mediators like TGF-β, IL-10 and IL-6; angiogenic factors (VEGFA, VEGFC) together with molecules promoting the chemotaxis and the activation of protumor myeloid cells (CCL2, CCL7, CCL8, CCL13) and macrophages (CSF1); fibroblasts and extracellular matrix, and gene signatures associated with wound-healing; and pathways involved in epithelial-to-mesenchymal transition (EMT), a process allowing to gain invasive properties and spread locally and systemically (48).
Cold tumors: the role of myeloid cells and EMT
The TME depicted by Hugo and collaborators as featuring immune resistant lesions is a concentration of most of the known immunosuppressive mechanisms that create a hostile environment to T cells reminiscent of the immunotolerant background required by tissue repair.
The link between EMT and cold tumors in NSCLC is supported by the evidence that the expression of a panel of EMT genes (CDH2, FOXC2, SNAI1, SNAI2, TWIST1, GSC, FN1, ITBG6, MMP3 and MMP9) is associated with exclusion of both CD4+ and CD8+ T cells, and the secretion of multiple immunosuppressive cytokines, including IL-10 and TGF-β (49).
Mutually exclusive with CD8+ T cell infiltration in NSCLC is also the accrual of cells of myeloid origin including neutrophils and macrophages. Indeed, the microenvironment of lung cancer is highly enriched in granulocytes and mononuclear phagocytes and their presence is inversely correlated with the level of T cell infiltrate, indicating an immunosuppressive pressure resulting in a more aggressive disease, as depicted by the anti-correlation between myeloid cell tumor signature and OS (50,51).
The large accrual of neutrophils, macrophages and MDSCs in NSCLC appears to be mediated at least in part by EGFR activation and the ability of this oncogenic pathway to drive a broad series of immune escape processes. EGFR activation leads to the autonomous immune-independent upregulation of PD-L1 and the consequent block of T cell activation and function triggered by PD-1 engagement (52). Concomitantly, EGFR activated cells release significant levels of immunosuppressive cytokines (IL-6, TGF-β), proangiogenic factors (VEGF), and mediators of myeloid cell accrual and activation (CCL2, GM-CSF), rendering TME a cold milieu in terms of adaptive immunity and T cell infiltrate (52).
Circulating myeloid cells: a biomarker to monitor systemic immune suppression in NSCLC
Accumulation of myeloid cells at tumor site means having potent and persistent tumor allies sustaining the tropism of transformed cells and promoting local and systemic cancer spread and progression (Figure 3). This large family of cellular subsets, key components of chronic inflammation, is essential in favoring neoangiogenesis (through the release of VEGF and the differentiation of monocytic precursors into endothelial cells), remodeling extracellular matrix (via the secretion of TGF-β), inducing EMT and suppressing adaptive T cell-mediated immune responses through the release of TGF-β, IL-6, ROS, iNOS, IDO, arginase, and the expression of PD-L1 or CD39/CD37 adenosine pathway (53). It is important to underline that these effects are also exerted systemically in peripheral immune organs such as lymph nodes, spleen and bone marrow, where they contribute in setting up the pre-metastatic niche and favor other metastatic sites such as osteolytic lesions (54) (Figure 4).
At difference with lymphoid cells, produced mostly in the lymph nodes, myeloid cells present in a tissue during chronic stimulation arise from residential cells or derive from the bone marrow. A proportion of tumor-associated macrophages (TAM) that in the TME acquire a protumor immunosuppressive phenotype, generally defined as M2 (55), derive from the polarization of resident macrophages. This is a local subset present from birth as “patrolling monocytes” typically in organs in direct contact with external environment such as lung and kidney (56), which is rapidly engaged by the tumor to sustain its own growth through chronic inflammation.
Most of tumor-related myeloid cells come instead from the bone marrow as the result of altered myelopoiesis that usually parallels cancer development. Indeed, the release by TME of factors that can enter the blood stream, ranging from circulating tumor cells, to soluble cytokines and chemokines, miRNAs and exosomes, molds myelogenesis in the bone marrow and drives the mobilization of altered (in terms of numbers and differentiation stage) myeloid cells including neutrophils, monocytes and MDSC (53). Besides homing to tumor site for promoting local invasion and systemic progression, these cells contribute to create a state of systemic immunosuppression profoundly influencing disease course and response to therapy (Figure 3).
Quantitatively and qualitatively aberrant myeloid cells accumulate in peripheral blood of patients with NSCLC as well as in most solid tumors as a hallmark of aggressive disease. This process involves monocytes and neutrophils in different activation/differentiation states (57), together with a heterogeneous subset of immature myeloid elements globally known as MDSC. Extensive studies in preclinical models have shown that early during cancer development bone marrow is involved in a process of reactive responses including the activation of myelogenesis and the release of immature elements. As a reaction to the presence of tumor cells and the consequent persistent immune stimulation, MDSC circulate in body fluids and colonize tumor site well as peripheral lymphoid organs, substantially to cool-off cancer-induced inflammation but unfortunately antagonizing antitumor immunity.
The occurrence of this process in NSCLC patients is witnessed by the increased frequency of monocytic (CD14+HLA-DRneg) and granulocytic (CD15+HLA-DRneg) MDSC that is reported in direct association with disease stage and poor prognosis (58). Interestingly, it is also clearly emerging that a sign of this process can be intercepted by standard blood tests as increased neutrophils, monocytes and their relative ratio with lymphocytes that has been reproducibly depicted as a negative prognostic factor and a biomarker of poor response to therapy including ICI (59,60). Hence, systemic immunosuppression deriving from the active cross-talk between tumor site and the bone marrow, may heavily condition tumor-bearing host and create a hostile environment to the protective activity of our inner immune surveillance.
Therapeutic implications
The clinical implications of tumor immunity have drastically changed the scenario of therapeutic strategies in NSCLC patients. Immunotherapy based on the removal of immune escape mechanisms through the administration of antagonizing antibodies targeting immune checkpoints such as PD-1, PD-L1 or CTLA-4, has recently become standard treatment for patients displaying no oncogenic mutations targetable by specific tyrosine kinase inhibitors (TKI) (61). Nevertheless, still open is the search for biomarkers to identify resistant patients and to improve the therapeutic benefit by combinatorial approaches. In this view, the possibility to impact the systemic cancer-related immunosuppression and its negative effects host on defenses appears a promising path. Preliminary although indirect confirmation of this hypothesis is the evidence that ICI improve their therapeutic efficacy when combined with chemotherapy, as depicted by recent clinical experience in early disease (62). Indeed, mostly through the myelotoxic activity that chemotherapy often mediates as side effect, a significant down-sizing of the myeloid cell population and the MDSC subsets can be obtained by the administration of cisplatin-based chemotherapy (63) or anti-angiogenics (64). This should result in a more prone environment to the activation of T cell-mediated adaptive immunity induced by ICI and likely a more effective immune-mediated tumor control. Of note, the immunomodulating properties of standard cancer therapy are rapidly emerging and supporting clinical trial testing combination strategies (65).
Multiple are the tools that oncologists have in their hands to potentially contrast myeloid cell accrual in NSCLC patients, and prospective studies testing the hypothesis should be encouraged. Indeed, as depicted in Table 1, several drugs have been reported to interfere with the myeloid cell differentiation and survival as off-target effect. For instance, in addition to chemotherapeutics, anti-angiogenic drugs are endowed with the marked ability to block MDSC accrual by antagonizing VEGF pathway and its stimulatory effect on myeloid cells (66). Similarly, ibrutinib, an irreversible inhibitor of Bruton’s tyrosine kinase (BTK) used in B cell malignancies, can antagonize MDSC activity and function in diverse cancer settings (67). Interestingly, markers expressed on myeloid cell components and exploited for targeted therapies in hematological cancers, like CD38 and CD30, have been also reported to persistently reduce MDSC frequency and function (68). Similarly, the anti-inflammatory effect of specific radiotherapy regimens, including ablative radiotherapy, can also change tumor immune microenvironment and contribute to induce durable complete remissions (69). The acknowledged key role of myeloid cells in disease progression and the evidence that their removal overcomes resistance to immunotherapy in animal models have promoted the development of specific drugs able to block myeloid cell survival and immunosuppressive pathways in vivo. Among these molecules, some have already reached advanced phases of clinical testing such as the anti-CSF1R mAb (70), blocking the activation of tumor associated macrophages and myeloid cells by CSF-1 and IL34. Drugs targeting mediators of MDSC-immunosuppressive activity, such as anti-TGF-β (71) and anti-ROS or iNOS (72) are currently being developed and hold promise of increasing efficacy of immunotherapies by imparing cancer-related immunosuppression.
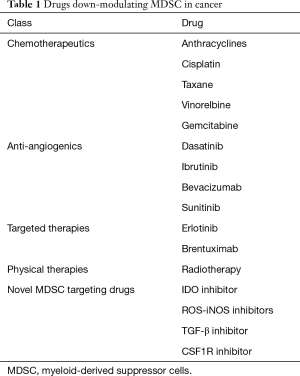
Full table
Milestones and future directions
Thanks to the amazing boost that preclinical and clinical research in lung cancer immunology has experienced in the last few years, we gained solid and unquestionable evidence about the immunogenic features of NSCLC. Specifically, we need to keep in mind that:
- CD8+ T cell infiltrate, when significantly present, can control disease progression as shown by its role as independent positive prognostic factor; it could also represent the basis for PD-1 blockers to work in vivo and as such contribute in predicting response to ICI;
- Infiltrating T cells recognize a broad array of antigens (cancer/testis, epithelial, neoantigens); this broad antigenic repertoire could be exploited to potentiate inner immunity by cancer vaccine strategies;
- Protective TIL are clonal, express the memory marker CD103 and high levels of immune checkpoints; defining the specific mechanisms underlying CD103+ T cell activation could lead to more effective strategies to induce full-fledged anti-cancer immune response;
- TIL lacking tumors are instead featured by myeloid cell infiltrate, EMT and driver oncogenic mutations. This innate immunity prevailing milieu, still needing to be fully understood in most cancers including NSCLC, holds promise for novel and complementary approaches of immunotherapy. In this view, standard cancer treatments or novel drugs impacting on myeloid components represent a valid option.
Acknowledgements
Funding: This article was supported by AIRC 5x1000 (number 12162).
Footnote
Conflicts of Interest: The authors have no conflicts of interest to declare.
References
- Clemente CG, Mihm MC Jr, Bufalino R, et al. Prognostic value of tumor infiltrating lymphocytes in the vertical growth phase of primary cutaneous melanoma. Cancer 1996;77:1303-10. [Crossref] [PubMed]
- Johnson SK, Kerr KM, Chapman AD, et al. Immune cell infiltrates and prognosis in primary carcinoma of the lung. Lung Cancer 2000;27:27-35. [Crossref] [PubMed]
- Georgiannos SN, Renaut A, Goode AW, et al. The immunophenotype and activation status of the lymphocytic infiltrate in human breast cancers, the role of the major histocompatibility complex in cell-mediated immune mechanisms, and their association with prognostic indicators. Surgery 2003;134:827-34. [Crossref] [PubMed]
- Vgenopoulou S, Lazaris AC, Markopoulos C, et al. Immunohistochemical evaluation of immune response in invasive ductal breast cancer of not-otherwise-specified type. Breast 2003;12:172-8. [Crossref] [PubMed]
- Schmidt M, Bohm D, Von TC, et al. The humoral immune system has a key prognostic impact in node-negative breast cancer. Cancer Res 2008;68:5405-13. [Crossref] [PubMed]
- Erdag G, Schaefer JT, Smolkin ME, et al. Immunotype and immunohistologic characteristics of tumor-infiltrating immune cells are associated with clinical outcome in metastatic melanoma. Cancer Res 2012;72:1070-80. [Crossref] [PubMed]
- Nielsen JS, Sahota RA, Milne K, et al. CD20+ tumor-infiltrating lymphocytes have an atypical. Clin Cancer Res 2012;18:3281-92. [Crossref] [PubMed]
- Giraldo NA, Becht E, Vano Y, et al. Tumor-Infiltrating and Peripheral Blood T-cell Immunophenotypes Predict Early Relapse in Localized Clear Cell Renal Cell Carcinoma. Clin Cancer Res 2017;23:4416-28. [Crossref] [PubMed]
- Chung YR, Kim HJ, Jang MH, et al. Prognostic value of tumor infiltrating lymphocyte subsets in breast cancer depends on hormone receptor status. Breast Cancer Res Treat 2017;161:409-20. [Crossref] [PubMed]
- Barnes TA, Amir E. HYPE or HOPE: the prognostic value of infiltrating immune cells in cancer. Br J Cancer 2017;117:451-60. [Crossref] [PubMed]
- Gentles AJ, Newman AM, Liu CL, et al. The prognostic landscape of genes and infiltrating immune cells across human cancers. Nat Med 2015;21:938-45. [Crossref] [PubMed]
- Kern DE, Gillis S, Okada M, et al. The role of interleukin-2 (IL-2) in the differentiatin of cytotoxic T cells: the effect of monoclonal anti-IL-2 antibody and absorption with IL-2 dependent T cell lines. J Immunol 1981;127:1323-8. [PubMed]
- Kim PS, Ahmed R. Features of responding T cells in cancer and chronic infection. Curr Opin Immunol 2010;22:223-30. [Crossref] [PubMed]
- Rosenberg SA, Packard BS, Aebersold PM, et al. Use of tumor-infiltrating lymphocytes and interleukin-2 in the immunotherapy of patients with metastatic melanoma. A preliminary report. N Engl J Med 1988;319:1676-80. [Crossref] [PubMed]
- Rosenberg SA, Yannelli JR, Yang JC, et al. Treatment of patients with metastatic melanoma with autologous tumor-infiltrating lymphocytes and interleukin 2. J Natl Cancer Inst 1994;86:1159-66. [PubMed]
- Newick K, O'Brien S, Moon E, et al. CAR T Cell Therapy for Solid Tumors. Annu Rev Med 2017;68:139-52. [Crossref] [PubMed]
- Coulie PG, Van den Eynde BJ, Van der Bruggen P, et al. Tumour antigens recognized by T lymphocytes: at the core of cancer immunotherapy. Nat Rev Cancer 2014;14:135-46. [Crossref] [PubMed]
- Zeltsman M, Dozier J, McGee E, et al. CAR T-cell therapy for lung cancer and malignant pleural mesothelioma. Transl Res 2017;187:1-10. [Crossref] [PubMed]
- Kawakami Y, Eliyahu S, Sakaguchi K, et al. Identification of the immunodominant peptides of the MART-1 human melanoma antigen recognized by the majority of HLA-A2-restricted tumor infiltrating lymphocytes. J Exp Med 1994;180:347-52. [Crossref] [PubMed]
- Kawakami Y, Rosenberg SA. Immunobiology of human melanoma antigens MART-1 and gp100 and their use for immuno-gene therapy. Int Rev Immunol 1997;14:173-92. [Crossref] [PubMed]
- Kawakami Y, Eliyahu S, Jennings C, et al. Recognition of multiple epitopes in the human melanoma antigen gp100 by tumor-infiltrating T lymphocytes associated with in vivo tumor regression. J Immunol 1995;154:3961-8. [PubMed]
- Baxevanis CN, Perez SA. Cancer vaccines: limited success but the research should remain viable. Expert Rev Vaccines 2016;15:677-80. [Crossref] [PubMed]
- Whiteside TL. Immune suppression in cancer: effects on immune cells, mechanisms and future therapeutic intervention. Semin Cancer Biol 2006;16:3-15. [Crossref] [PubMed]
- Okazaki T, Honjo T. PD-1 and PD-1 ligands: from discovery to clinical application. Int Immunol 2007;19:813-24. [Crossref] [PubMed]
- Li X, Shao C, Shi Y, et al. Lessons learned from the blockade of immune checkpoints in cancer immunotherapy. J Hematol Oncol 2018;11:31. [Crossref] [PubMed]
- Tumeh PC, Harview CL, Yearley JH, et al. PD-1 blockade induces responses by inhibiting adaptive immune resistance. Nature 2014;515:568-71. [Crossref] [PubMed]
- Choueiri TK, Fishman MN, Escudier B, et al. Immunomodulatory Activity of Nivolumab in Metastatic Renal Cell Carcinoma. Clin Cancer Res 2016;22:5461-71. [Crossref] [PubMed]
- Wei SC, Levine JH, Cogdill AP, et al. Distinct Cellular Mechanisms Underlie Anti-CTLA-4 and Anti-PD-1 Checkpoint Blockade. Cell 2017;170:1120-33. [Crossref] [PubMed]
- Kamphorst AO, Pillai RN, Yang S, et al. Proliferation of PD-1+ CD8 T cells in peripheral blood after PD-1-targeted therapy in lung cancer patients. Proc Natl Acad Sci U S A 2017;114:4993-8. [Crossref] [PubMed]
- Janetzki S, Hoos A, Melief CJ, et al. Structured reporting of T cell assay results. Cancer Immun 2013;13:13. [PubMed]
- Galon J, Costes A, Sanchez-Cabo F, et al. Type, density, and location of immune cells within human colorectal tumors predict clinical outcome. Science 2006;313:1960-4. [Crossref] [PubMed]
- Schalper KA, Brown J, Carvajal-Hausdorf D, et al. Objective measurement and clinical significance of TILs in non-small cell lung cancer. J Natl Cancer Inst 2015;107. [Crossref] [PubMed]
- Djenidi F, Adam J, Goubar A, et al. CD8+CD103+ tumor-infiltrating lymphocytes are tumor-specific tissue-resident memory T cells and a prognostic factor for survival in lung cancer patients. J Immunol 2015;194:3475-86. [Crossref] [PubMed]
- Donnem T, Hald SM, Paulsen EE, et al. Stromal CD8+ T-cell Density-A Promising Supplement to TNM Staging in Non-Small Cell Lung Cancer. Clin Cancer Res 2015;21:2635-43. [Crossref] [PubMed]
- Turajlic S, Litchfield K, Xu H, et al. Insertion-and-deletion-derived tumour-specific neoantigens and the immunogenic phenotype: a pan-cancer analysis. Lancet Oncol 2017;18:1009-21. [Crossref] [PubMed]
- Yarchoan M, Johnson BA III, Lutz ER, et al. Targeting neoantigens to augment antitumour immunity. Nat Rev Cancer 2017;17:209-22. [Crossref] [PubMed]
- Djureinovic D, Hallstrom BM, Horie M, et al. Profiling cancer testis antigens in non-small-cell lung cancer. JCI Insight 2016;1. [Crossref] [PubMed]
- Li B, Li T, Pignon JC, et al. Landscape of tumor-infiltrating T cell repertoire of human cancers. Nat Genet 2016;48:725-32. [Crossref] [PubMed]
- Ganesan AP, Clarke J, Wood O, et al. Tissue-resident memory features are linked to the magnitude of cytotoxic T cell responses in human lung cancer. Nat Immunol 2017;18:940-50. [Crossref] [PubMed]
- Finn OJ. A Believer's Overview of Cancer Immunosurveillance and Immunotherapy. J Immunol 2018;200:385-91. [Crossref] [PubMed]
- Engels EA, Pfeiffer RM, Fraumeni JF Jr, et al. Spectrum of cancer risk among US solid organ transplant recipients. JAMA 2011;306:1891-901. [Crossref] [PubMed]
- Schreiber RD, Old LJ, Smyth MJ. Cancer immunoediting: integrating immunity's roles in cancer suppression and promotion. Science 2011;331:1565-70. [Crossref] [PubMed]
- Chen DS, Mellman I. Elements of cancer immunity and the cancer-immune set point. Nature 2017;541:321-30. [Crossref] [PubMed]
- Lanitis E, Dangaj D, Irving M. Mechanisms regulating T-cell infiltration and activity in solid tumors. Ann Oncol 2017;28:xii18-32. [Crossref] [PubMed]
- Lizotte PH, Ivanova EV, Awad MM, et al. Multiparametric profiling of non-small-cell lung cancers reveals distinct immunophenotypes. JCI Insight 2016;1. [Crossref] [PubMed]
- Dong ZY, Zhang JT, Liu SY, et al. EGFR mutation correlates with uninflamed phenotype and weak immunogenicity, causing impaired response to PD-1 blockade in non-small cell lung cancer. Oncoimmunology 2017;6. [Crossref] [PubMed]
- Hugo W, Zaretsky JM, Sun L, et al. Genomic and Transcriptomic Features of Response to Anti-PD-1 Therapy in Metastatic Melanoma. Cell 2016;165:35-44. Erratum in: Genomic and Transcriptomic Features of Response to Anti-PD-1 Therapy in Metastatic Melanoma. [Cell 2017]. [Crossref] [PubMed]
- Bu X, Mahoney KM, Freeman GJ. Learning from PD-1 Resistance: New Combination Strategies. Trends Mol Med 2016;22:448-51. [Crossref] [PubMed]
- Chae YK, Choi WM, Bae WH, et al. Overexpression of adhesion molecules and barrier molecules is associated with differential infiltration of immune cells in non-small cell lung cancer. Sci Rep 2018;8:1023. [Crossref] [PubMed]
- Lavin Y, Kobayashi S, Leader A, et al. Innate Immune Landscape in Early Lung Adenocarcinoma by Paired Single-Cell Analyses. Cell 2017;169:750-65. [Crossref] [PubMed]
- Kargl J, Busch SE, Yang GH, et al. Neutrophils dominate the immune cell composition in non-small cell lung cancer. Nat Commun 2017;8:14381. [Crossref] [PubMed]
- Akbay EA, Koyama S, Carretero J, et al. Activation of the PD-1 pathway contributes to immune escape in EGFR-driven lung tumors. Cancer Discov 2013;3:1355-63. [Crossref] [PubMed]
- Gabrilovich DI. Myeloid-Derived Suppressor Cells. Cancer Immunol Res 2017;5:3-8. [Crossref] [PubMed]
- Sawant A, Ponnazhagan S. Myeloid-derived suppressor cells as osteoclast progenitors: a novel target for controlling osteolytic bone metastasis. Cancer Res 2013;73:4606-10. [Crossref] [PubMed]
- Poh AR, Ernst M. Targeting Macrophages in Cancer: From Bench to Bedside. Front Oncol 2018;8:49. [Crossref] [PubMed]
- Hanna RN, Cekic C, Sag D, et al. Patrolling monocytes control tumor metastasis to the lung. Science 2015;350:985-90. [Crossref] [PubMed]
- Veglia F, Perego M, Gabrilovich D. Myeloid-derived suppressor cells coming of age. Nat Immunol 2018;19:108-19. [Crossref] [PubMed]
- Vetsika EK, Koinis F, Gioulbasani M, et al. A circulating subpopulation of monocytic myeloid-derived suppressor cells as an independent prognostic/predictive factor in untreated non-small lung cancer patients. J Immunol Res 2014;2014. [Crossref] [PubMed]
- Mezquita L, Auclin E, Ferrara R, et al. Association of the Lung Immune Prognostic Index With Immune Checkpoint Inhibitor Outcomes in Patients With Advanced Non-Small Cell Lung Cancer. JAMA Oncol 2018;4:351-7. [Crossref] [PubMed]
- Yin Y, Wang J, Wang X, et al. Prognostic value of the neutrophil to lymphocyte ratio in lung cancer: A meta-analysis. Clinics (Sao Paulo) 2015;70:524-30. [Crossref] [PubMed]
- Bender E. Cancer immunotherapy. Nature 2017;552:S61. [Crossref] [PubMed]
- Deslypere G, Gullentops D, Wauters E. Immunotherapy in non-metastatic non-small cell lung cancer: Can the benefits of stage IV therapy be translated into earlier stages? Ther Adv Med Oncol 2018;10. [Crossref] [PubMed]
- De Biasi AR, Villena-Vargas J, Adusumilli PS. Cisplatin-induced antitumor immunomodulation: a review of preclinical and clinical evidence. Clin Cancer Res 2014;20:5384-91. [Crossref] [PubMed]
- Koinis F, Vetsika EK, Aggouraki D, et al. Effect of First-Line Treatment on Myeloid-Derived Suppressor Cells' Subpopulations in the Peripheral Blood of Patients with Non-Small Cell Lung Cancer. J Thorac Oncol 2016;11:1263-72. [Crossref] [PubMed]
- Brown JS, Sundar R, Lopez J. Combining DNA damaging therapeutics with immunotherapy: more haste, less speed. Br J Cancer 2018;118:312-24. [Crossref] [PubMed]
- Verzoni E, De Cecco L, Dugo M, et al. Broad immunomodulating effect of first-line Pazopanib in metastatic renal cell carcinoma patient. Ann Oncol 2017;28:v295-329.
- Stiff A, Trikha P, Wesolowski R, et al. Myeloid-Derived Suppressor Cells express Bruton's tyrosine kinase and can be depleted in tumor-bearing hosts by ibrutinib treatment. Cancer Res 2016;76:2125-36. [Crossref] [PubMed]
- Krejcik J, Casneuf T, Nijhof IS, et al. Daratumumab depletes CD38+ immune regulatory cells, promotes T-cell expansion, and skews T-cell repertoire in multiple myeloma. Blood 2016;128:384-94. [Crossref] [PubMed]
- Vatner RE, Formenti SC. Myeloid-derived cells in tumors: effects of radiation. Semin Radiat Oncol 2015;25:18-27. [Crossref] [PubMed]
- Papadopoulos KP, Gluck L, Martin LP, et al. First-in-Human Study of AMG 820, a Monoclonal Anti-Colony-Stimulating Factor 1 Receptor Antibody, in Patients with Advanced Solid Tumors. Clin Cancer Res 2017;23:5703-10. [Crossref] [PubMed]
- De Gramont A, Faivre S, Raymond E. Novel TGF-beta inhibitors ready for prime time in onco-immunology. Oncoimmunology 2016;6. [Crossref] [PubMed]
- Nicco C, Batteux F. ROS Modulator Molecules with Therapeutic Potential in Cancers Treatments. Molecules 2017;23. [Crossref] [PubMed]