Diagnostic and prognostic significance of lysophosphatidic acid in malignant pleural effusions
Introduction
Malignant pleural effusion (MPE), the accumulation of pleural fluid due to metastasis of cancer to the pleural space, is a common clinical manifestations with an annual incidence rate of approximately 500 patients/million in the United States (1,2). Malignancies account for approximately 40% of all pleural effusions; lung cancer is the most common metastatic tumor associated with MPE (3,4). In fact, at the time of diagnosis, 14% of patients with non-small-cell lung cancer have a pleural effusion, because this cancer disseminates to the pleura, approximately 50% will eventually develop a pleural effusion (5). Whereas, the most common causes of benign pleural effusion (BPE) are tuberculosis and pneumonia. Therapeutic management differs for these two types of effusions; therefore, it is clinically important to differentiate between them rapidly and accurately. Finding malignant cells, in either the pleural fluid by cytological examination or in a pleural biopsy, has traditionally been the primary diagnostic method for MPE (6). However, it is often difficult to find malignant cells in MPE, and because pleural biopsy is an invasive procedure it is not readily accepted by some patients. Although several tumor markers, such as carcinoembryonic antigen (CEA), cancer antigen 125 (CA-125), and cytokeratin fragment 19 (CYFRA 21-1), can assist the diagnosis of MPE, their specificity and sensitivity are limited (7). Therefore, new indices that are more accurate are needed. Several factors that participate in the formation of pleural effusion been identified; however, our current understanding of the basic mechanisms by which effusion accumulates within the pleural space is poor. Metastasis of tumor cells into the pleural space may lead to production of large amounts of PE. In addition, angiogenic factors released by the infiltrated tumor cells or stromal cells play an important role in the development of PE (8).
Lysophosphatidic acid (LPA) is a bioactive phospholipid signaling mediator that is present in almost all mammalian cells and tissues (9). Recent research indicates that LPA promotes the proliferation and invasion of cancer cells (10) and regulates tumor angiogenesis (9). In vitro experiments found that A549 lung carcinoma cells express endogenous LPA receptors and that LPA can activate this receptor, enhance the degradation of the p53 tumor suppressor and thereby allow cancer cell proliferation and motility (11){Murph, 2007 #6516}. Therefore, LPA also plays an important role in lung cancer.
Previous studies reported that malignant effusions contained LPA-like activity, but they primarily evaluated ascites of ovarian cancer patients (12), and the method for detecting LPA, by the neurite retraction assay, was limited. Most importantly, whether LPA is actually present in MPE and whether there is a significant difference in LPA levels between MPE and BPE remained unknown.
The aims of our study were to explore the diagnostic value of LPA in differentiating between MPE and BPE and to evaluate the prognostic value of LPA in MPE caused by lung cancer.
Patients and methods
Patients
This retrospective cohort study included 123 patients with PE who were hospitalized in the Respiratory Department, Nanjing General Hospital of Nanjing Military Command in China, from September 2009 through September 2013. Written consent was obtained from all the patients who participated in this study. Twenty-nine patients (20 males and nine females; mean age, 61.21 years) had BPEs, and 94 had MPEs caused by lung cancer (55 males and 39 females; mean age, 63.29 years). Only patients diagnosed with primary malignancies were included; otherwise they were excluded.
Diagnostic criteria for pleural effusions
In our study, BPE mainly included tuberculosis PE (TPE) and parapneumonic PE. The diagnostic criteria for TPE were as follows: identification of M. tuberculosis, pleural biopsy revealing granulomatous tissue and positive response to anti-tuberculosis treatment. Pleural effusions were considered pneumonic if they had the following these characteristics: an acute febrile condition along with cough, yellow sputum, chest CT with pulmonary infiltration, leukocytosis with neutrophilic predominance in the PE and response to antibiotic treatment. The diagnostic criteria for MPE were malignant cells present either in the cytology of the pleural fluid or seen on histopathologic examination of a biopsy specimen of the pleura.
Sample collection and biochemical analyses
Pleural effusions were collected by routine thoracentesis performed after patients gave written the consent. Samples were centrifuged at 1,500 ×g for 10 min at –4 °C. The supernatants were dispensed into 1.5 mL Eppendorf tubes and were stored at –80 °C. LPA was determined using a commercial human LPA ELISA Kit (CUSABIO, China; Catalog Number CSB-EQ 028005HU). The assay was performed following the manufacturer instructions. The technicians were blinded to the clinical data.
Statistical methods
Statistical analyses were performed with SPSS Software 17.0. Patient demographics and disease characteristics were summarized using descriptive statistics. The student’s t-test was used to assess the difference of the LPA level between MPE and BPE. One-way ANOVA was used to compare the difference of LPA level in subgroups of MPE (adenocarcinoma, squamous cell carcinoma and small cell lung carcinoma) patients. Receiver operator characteristic curve (ROC) analysis was used to select the threshold value of LPA that best differentiated MPE from BPE, specifically the value that maximized the sum of specificity and sensitivity. OS was defined as the time interval from the date of diagnosis to the date of death from any cause or to the date on which the patient was last known to be alive. PFS was defined as the interval from the date of diagnosis to the date that progression was detected or to the date when the patient was last known to be disease free. Kaplan-Meier survival curves were used to examine the associations between the LPA concentration and the OS and PFS; significance of these associations was analyzed using the log-rank or Breslow test. A multivariate Cox proportional hazards model was used to assess whether LPA was an independent predictor of survival. For the above comparisons, P<0.05 was considered statistically significant.
Results
Our study was carried out with 123 patients (75 males and 48 females; age range, 17-83 years) with MPE (94 MPE) caused by lung cancer or BPE (29 BPE) caused by tuberculous pleuritis or pneumonia. The patient characteristics are shown in Table 1. There were no significant differences in gender, age, and smoking history between the MPE and BPE groups (P>0.05). Of the MPE cases, 74 (78.7%) had adenocarcinoma, 12 (12.8%) squamous carcinoma and eight (8.5%) small cell lung cancer. The MPE cytology results were 28 (29.8%) positive and 66 (70.2%) negative. There were 19 patients with TBE and ten with pneumonic PE in BPE group.
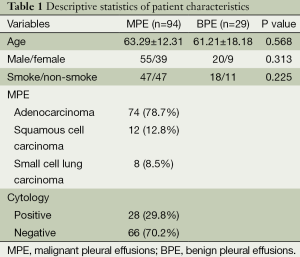
Full table
LPA levels in PE
As shown in Figure 1A, the level of LPA was significantly higher in patients with MPE (22.08±8.72 µg/L) than in those with BPE (14.61±5.12 µg/L; P=0.000). Subgroup analysis (Figure 1B) found that the levels of LPA in MPEs caused by adenocarcinoma, squamous cell carcinoma and small cell lung carcinoma, 22.01±9.28, 20.76±4.65, and 24.75±8.72 µg/L, respectively, were not significantly different (P=0.603).
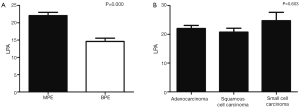
Relationship between LPA concentration and clinicopathologic factors in lung cancer patients with MPE
Because the mean LPA concentration was significantly higher in MPEs than in BPEs, we evaluated the relationship between the levels of LPA and gender, age, smoking history, histologic type of tumor, and a positive cytology result. However, as shown in Table 2, no significant associations between LPA concentration and these clinicopathologic factors were found.
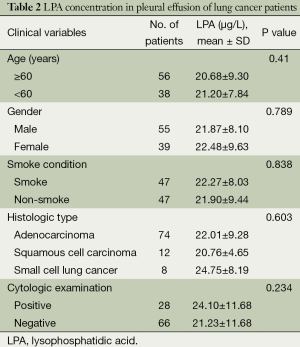
Full table
Discriminative power of LPA in MPE
ROC curve was created to find sensitivity and specificity of LPA in MPE versus BPE group. The area under the ROC curve was 0.769±0.045 (SE) (P=0.000, 95% CI, 0.68-0.857). Using a threshold value of 18.93 µg/L, LPA had a sensitivity of 60%, a specificity of 83%. The ROC curve is shown in Figure 2.
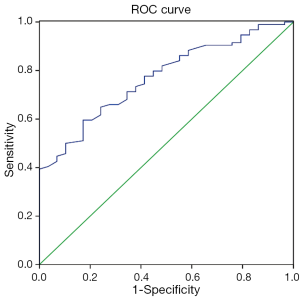
Prognostic value of LPA among MPE patients
For this analysis, the patients with MPE were divided into two groups based on the LPA level in their PE, either above or below the median LPA concentration, 20.57 µg/L. Figure 3A,B showed no significant differences in the OS rate [P=0.580, Log-rank (Mantel-Cox)] and the PFS rate [P=0.186, Log-rank (Mantel-Cox)] between the high LPA and low LPA concentration groups.
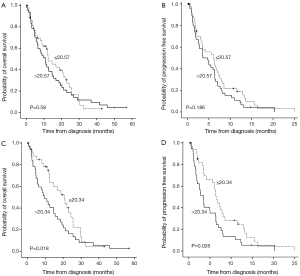
We also carried out a subgroup analysis based on the three types of lung cancer. The number of patients with MPE caused by squamous cell carcinoma and small cell lung carcinoma was too small to provide meaningful statistical results, so we only further analyzed the relationship between the LPA concentration and patient’s OS and PFS in patients with adenocarcinoma of the lung in the MPE group. The median LPA level, 20.34 µg/L, was used to separate these patients into high and low LPA concentration groups. The median OS intervals were 20.67 and 9.7 months, respectively, for the patients in the low and high LPA concentration groups [P=0.018, Breslow (generalized Wilcoxon); P=0.076, Log-rank (Mantel-Cox)] as depicted in Figure 3C. The median PFS intervals were 6.53 and 3.47 months, respectively, for the patients with low and high LPA concentrations [P=0.026, Log Rank (Mantel-Cox); P=0.009, Breslow (generalized Wilcoxon)] as depicted in Figure 3D.
Multivariate cox proportional hazards analysis
LPA concentration and other factors, including gender, age, smoking history, p-T status, lymph node metastasis and extrapulmonary distant metastasis, were analyzed by Cox’s proportional hazards regression models in the 74 patients with MPE caused by adenocarcinoma of the lung. We found that LPA concentration was an independent prognostic factor for PFS (P=0.034) but not for OS (P=0.112). Only LPA level was retained as a significant variable in the forward regression model, when controlling for other variables, based on the likelihood ratio test (Table 3).
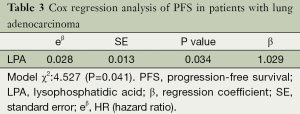
Full table
Discussion
This is the first study to investigate the potential of LPA, measured by ELISA, as a possible marker to differentiate MPE due to lung cancer from BPE. We not only showed that the level of LPA was higher in MPE than in BPE, but also that LPA concentration was an independent prognostic factor for PFS in patients with MPE who had adenocarcinoma of the lung.
LPA is a naturally occurring phospholipid produced by activated platelets, mesothelial cells, fibroblasts, adipocytes and some cancer cells (10,13) and now is recognized as an extracellular lipid mediator that evokes growth-factor-like responses in almost every cell type (10). LPA was first detected in serum and ascites from ovarian cancer patients. In 1998, Westermann et al. (12) found that malignant effusions contained LPA-like activity using a bioassay of neurite retraction in differentiated NIE-115 neuroblastoma cells described by Jalink et al. (14). Their study included malignant effusions caused by various types of cancers, but not benign effusion as a control group. Furthermore, malignant effusions caused by each type of cancer had a limited number of samples and only seven samples were from non-small lung cancer. That study’s primary finding was that the LPA-like activity in the effusions of ovarian cancer patients was significantly higher than in the effusions caused by other cancers.
In our study, we focused on MPE caused by lung cancer and we compared the levels of LPA between MPE and BPE using an ELISA to measure LPA, a more accurate detection method in a larger study population than that reported by Westermann et al. ROC curves for MPE versus BPE were analyzed and the cutoff value of 18.93 µg/L had a sensitivity of 60% and a specificity of 83%. Other tumor markers, such as CEA, CA-125, and CYFRA 21-1 have been widely used to distinguish between MPE and BPE. Huan-Zhong Shi et al. performed a meta-analysis of forty-five studies and found that the sensitivity and specificity of CEA for the diagnosis of MPE were 54% and 94%, respectively (15). Another meta-analysis reported that the sensitivity/specificity of these tumor markers for the diagnosis of MPE were as follows: CA 125, 48%/85%; and CYFRA 21-1, 55%/91%, respectively (16). In contrast, the specificity of LPA for the diagnoses of MPE was slightly lower than that of these tumor markers. However, much less research has been conducted on the diagnostic value of LPA in MPE than on these tumor markers, and the 123 PE samples in our study were too few to support definitive recommendations. Therefore, our results only suggest that LPA could be a new informative tumor marker for the diagnosis of MPE caused by lung cancer. More high quality studies with larger samples need to be performed.
LPA, an inducer of cell proliferation, migration and survival, has actions that are concordant with many of the ‘hallmarks of cancer’ (17) and suggestive of a role for LPA in the initiation or progression of malignant disease (10). In our study, we evaluated the prognostic value of LPA in MPE caused by three pathological types of lung cancer and in MPE caused only by adenocarcinoma of the lung. We found that LPA concentration was not significantly associated with the OS or PFS of the three types of lung cancer. These results agreed with those reported by Westermann et al. (12). However, when we only considered the patients with MPE caused by lung adenocarcinoma we found that those patients with a lower LPA concentration in their MPE survived markedly longer (20.67 months, median OS) than patients with a higher LPA concentration in their MPE (9.7 months, median OS) based on univariate analysis of OS and PFS. Multivariate Cox proportional hazards analysis found that LPA concentration was significantly and independently associated with PFS but not with OS. These findings mean that patients with lower LPA concentrations in their MPE have a longer PFS than those with higher LPA concentrations. Westermann et al. (12) reported that disease free and overall survival were not in any way linked to LPA-equivalent levels in malignant effusions. The reason for these different results may be that they evaluated MPE due to various cancers; whereas, we specifically analyzed MPE caused by lung adenocarcinoma. Accordingly, we propose that LPA may contribute to the progression of MPE caused by lung adenocarcinoma.
Several potential biologic mechanisms might explain our findings and are worthy of further study. One possible explanation is that LPA stimulates lung adenocarcinoma cells more than it stimulates squamous cell or small cell growth at concentrations just as LPA present in ascitic fluid markedly stimulates ovarian tumor growth (18). Therefore, those patients with both lung adenocarcinoma and a higher LPA level in their MPE had a shorter OS and PFS time. Furthermore, LPA has been found to stimulate hypoxia-inducible factor-1-independent VEGF expression that promotes angiogenesis and vascular permeability and leads to ascites formation (18-20). Xiaoyu Xu found that LPA antagonist BrP-LPA inhibited tumor growth and angiogenesis in an engineered three-dimensional lung cancer xenograft model (20). According to our clinical results and these previous studies, we propose that LPA facilitates MPE caused by lung adenocarcinoma by promoting angiogenesis and that LPA may be a potential target for MPE therapy. However, these are only our hypotheses. Additional studies with larger number of samples are necessary to determine whether or how LPA affects the pathogenesis of pleural effusions in lung cancer.
Conclusions
Our findings indicate that the level of LPA in PE can assist to distinguish MPE from BPE and the level of LPA in MPE is inversely related to the length of OS and PFS in lung adenocarcinoma. LPA may contribute to the progression of MPE caused by lung adenocarcinoma. We believe that LPA may be a therapeutic target to reduce or inhibit MPE (12). Additional studies that are more extensive are needed to understand the role and mechanism of LPA in the formation of MPE.
Acknowledgements
This work was supported by grants from the Natural Science Foundation of Jiangsu province (No. BK2011658), Clinical Science and Technology Project of Jiangsu Province (NO. BL2013026) and National Natural Science Foundation of China (NO. 81302032). We greatly thank the editors for their valuable comments and suggestions. We also thank native English-speaking experts of BioMed Proofreading for polishing the English translation of our paper. The authors declare that they have no conflict of interest relating to the publication of this manuscript.
Disclosure: The authors declare no conflict of interest.
References
- American Thoracic Society. Management of malignant pleural effusions. Am J Respir Crit Care Med 2000;162:1987-2001. [PubMed]
- Stathopoulos GT, Psallidas I, Moustaki A, et al. A central role for tumor-derived monocyte chemoattractant protein-1 in malignant pleural effusion. J Natl Cancer Inst 2008;100:1464-76. [PubMed]
- Martínez-Moragón E, Aparicio J, Sanchis J, et al. Malignant pleural effusion: prognostic factors for survival and response to chemical pleurodesis in a series of 120 cases. Respiration 1998;65:108-13. [PubMed]
- Yoneda KY, Mathur PN, Gasparini S. The evolving role of interventional pulmonary in the interdisciplinary approach to the staging and management of lung cancer. Part III: diagnosis and management of malignant pleural effusions. Clin Lung Cancer 2007;8:535-47. [PubMed]
- Naito T, Satoh H, Ishikawa H, et al. Pleural effusion as a significant prognostic factor in non-small cell lung cancer. Anticancer Res 1997;17:4743-6. [PubMed]
- Khaleeq G, Musani AI. Emerging paradigms in the management of malignant pleural effusions. Respir Med 2008;102:939-48. [PubMed]
- Tomita M, Shimizu T, Ayabe T, et al. Carcinoembryonic antigen level in serum and pleural lavage fluid in non-small cell lung cancer. Thorac Cardiovasc Surg 2010;58:350-3. [PubMed]
- Sumi M, Kagohashi K, Satoh H, et al. Endostatin levels in exudative pleural effusions. Lung 2003;181:329-34. [PubMed]
- Chen Y, Ramakrishnan DP, Ren B. Regulation of angiogenesis by phospholipid lysophosphatidic acid. Front Biosci (Landmark Ed) 2013;18:852-61. [PubMed]
- Mills GB, Moolenaar WH. The emerging role of lysophosphatidic acid in cancer. Nat Rev Cancer 2003;3:582-91. [PubMed]
- Murph MM, Hurst-Kennedy J, Newton V, et al. Lysophosphatidic acid decreases the nuclear localization and cellular abundance of the p53 tumor suppressor in A549 lung carcinoma cells. Mol Cancer Res 2007;5:1201-11. [PubMed]
- Westermann AM, Havik E, Postma FR, et al. Malignant effusions contain lysophosphatidic acid (LPA)-like activity. Ann Oncol 1998;9:437-42. [PubMed]
- Aoki J. Mechanisms of lysophosphatidic acid production. Semin Cell Dev Biol 2004;15:477-89. [PubMed]
- Jalink K, Eichholtz T, Postma FR, et al. Lysophosphatidic acid induces neuronal shape changes via a novel, receptor-mediated signaling pathway: similarity to thrombin action. Cell Growth Differ 1993;4:247-55. [PubMed]
- Shi HZ, Liang QL, Jiang J, et al. Diagnostic value of carcinoembryonic antigen in malignant pleural effusion: a meta-analysis. Respirology 2008;13:518-27. [PubMed]
- Liang QL, Shi HZ, Qin XJ, et al. Diagnostic accuracy of tumour markers for malignant pleural effusion: a meta-analysis. Thorax 2008;63:35-41. [PubMed]
- Hanahan D, Weinberg RA. The hallmarks of cancer. Cell 2000;100:57-70. [PubMed]
- Hu YL, Tee MK, Goetzl EJ, et al. Lysophosphatidic acid induction of vascular endothelial growth factor expression in human ovarian cancer cells. J Natl Cancer Inst 2001;93:762-8. [PubMed]
- Song Y, Wu J, Oyesanya RA, et al. Sp-1 and c-Myc mediate lysophosphatidic acid-induced expression of vascular endothelial growth factor in ovarian cancer cells via a hypoxia-inducible factor-1-independent mechanism. Clin Cancer Res 2009;15:492-501. [PubMed]
- Xu X, Prestwich GD. Inhibition of tumor growth and angiogenesis by a lysophosphatidic acid antagonist in an engineered three-dimensional lung cancer xenograft model. Cancer 2010;116:1739-50. [PubMed]