Pulmonary mechanics and gas exchange characteristics in uncommon etiologies of acute respiratory distress syndrome
Introduction
Acute respiratory distress syndrome (ARDS) can be triggered by a multitude of direct and indirect insults to the lungs. The most common triggers are pneumonia, aspiration, non-pulmonary sepsis and trauma (1). Although these etiologies are well described in the literature, relatively less attention has focused on uncommon causes of ARDS such as pancreatitis, hepatic failure, transfusion-associated acute lung injury (TRALI), reperfusion lung injury, and inhalational injury. These diverse causes of acute lung injury account for 12–23% of cases (2-4), thus making it difficult to accumulate enough information to conduct a meaningful description of the pathophysiology.
Recently, we reported less common etiologies were distinguished from trauma-associated ARDS by having higher physiologic dead-space fraction (VD/VT) and oxygenation index (OI), along with lower respiratory system compliance (CRS) and arterial oxygen tension-to-inspired oxygen fraction (PaO2/FiO2) (4). Less common etiologies also were distinguished by having lower VD/VT than those with aspiration-associated ARDS and higher OI than those with non-pulmonary sepsis (4).
Because we have recorded a relatively large number of subjects with less-common ARDS etiologies, we inquired whether similar patterns in pulmonary mechanics and gas exchange might be apparent within this catchall category. We hypothesized that, consistent with the main findings of our previous study, direct causes of lung injury would likely produce higher VD/VT compared to indirect causes. We did not speculate whether CRS or oxygenation indices might differ between direct vs. indirect injury, nor between various etiologic subgroupings.
Methods
Briefly, as previously described (4), data were obtained from our hospital’s quality assurance ARDS management database used to monitor our adoption of the National Institutes of Health’s ARDS Clinical Trial’s Network (ARDSNet) lung-protective ventilation protocol (5). Acute lung injury criteria based on the American-European Consensus Conference definition was used prospectively for entering subjects into the database (6). Because of the subsequent development of the Berlin definition all subjects were re-evaluated and redefined according to the newer ARDS definition (7). Use of our quality assurance data for research purposes was approved by the University of California, San Francisco’s institutional review board for human subject protection with waiver of consent granted (IRB#16-19189).
Routine VD/VT measurements began in 2010 as part of implementing the ARDSNet protocol. Measurements were made within 12 h of protocol initiation with 94% of all measurements in the current study done on the day of ARDS onset, 4% within 24 h after ARDS onset and 2% within 96h. VD/VT was measured using the Enghoff-Bohr equation [VD/VT = (PaCO2 − PeCO2) ÷ PaCO2] whereby PaCO2 and PeCO2 are respectively the arterial and mixed expired carbon dioxide tensions (8). An arterial blood gas was drawn simultaneously with mixed expired CO2 measured by volumetric capnography (NICO monitor, Philips/Respironics, Wallingford, CT) as previously described (9).
Dead-space measurements coincided with a ventilator-systems check including expired tidal volume (VT) both in mL and relative to predicted body weight according to the ARDSNet protocol (5), end-inspiratory plateau pressure (Pplat) and positive end-expiratory pressure (PEEP). Consistent with our previous studies (4,9) assessments were made only when patients were observed to be calm and synchronous with the ventilator, in the absence of nursing care activities, and unless contraindicated, studied in the semi-recumbent position.
CRS was calculated as tidal volume (VT) ÷ (Pplat−PEEP) (10). Measures of oxygenation function included the PaO2/FiO2 ratio and OI which was calculated as the product of mean airway pressure and the percent of inspired oxygen divided by PaO2 (11). In addition, Lung Injury Score (LIS) (12), Simplified Acute Physiology Score II (SAPS II) (13) and Acute Physiology and Chronic Health Evaluation II (APACHE II) score (14) were calculated on the day of ARDS onset.
Of the 94 subjects identified with uncommon ARDS etiologies, 8 were excluded from the analysis because sepsis was a co-diagnosis. The 86 remaining subjects had 18 distinct etiologies, wherein only a minority of whom could be classified as direct (9%), versus indirect injury (79%) while 11% had idiopathic causes (Table 1). When distinct etiologies shared what we believed were similar injury mechanisms we subsumed these into a larger group to analyze their mechanical and gas exchange characteristics. For example, near-drowning and inhalation injury were grouped together; as were those with apparent secondary lung injury from a hyperimmune response (e.g., tumor lysis syndrome, Steven-Johnson Syndrome) In six of the 10 groups with eight or more subjects (inhalation injury, drug overdose, pancreatitis, hemorrhagic shock-reperfusion injury, neurologic injury and idiopathic) we also compared CRS, VD/VT, PaO2/FiO2 and OI between survivors and non-survivors. Only the two cases of cutaneous burns and the single case of malaria could not be placed into a larger grouping.
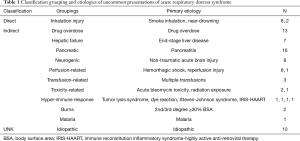
Full table
Statistical analysis was done using PRISM (Graphpad Software, La Jolla CA). Given the small sample size and non-normal distribution, data are reported as median and interquartile range. Comparisons across groups were made using Kruskal-Wallis and Dunn’s post-test. Comparisons between groups (i.e., non-survivors vs. survivors; indirect vs. direct injury) were made using Mann-Whitney tests. Categorical variables were assessed by Fisher Exact tests. Alpha was set at 0.05.
Results
Because of the small sample size and wide dispersion of data our results were not statistically significant, the only exceptions were higher APACHE II score in subjects with ARDS associated with drug overdose versus pancreatitis (Table 2). When classified as either direct versus indirect lung injury no differences were found in CRS [33 (23 to 34) vs. 29 (21 to 38) mL/cmH2O respectively, P=0.24], VD/VT [0.66 (0.57 to 0.73) vs. 0.63 (0.53 to 0.70) respectively, P=0.39], PaO2/FiO2 [115 (83 to 123) vs. 113 (91 to 158) mmHg respectively, P=0.96], OI [14.8 (11.5 to 18.2) vs. 14.1 (9.3 to 21.4) respectively, P=0.51], pH [7.29 (7.25 to 7.33) vs. 7.34 (7.27 to 7.41) respectively, P=0.14] or Base Deficit [−6.0 (−7.9 to −5.4) vs. −3.7 (−8.2 to −0.2) mEq/dL respectively, P=0.33].
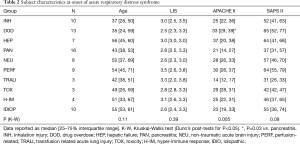
Full table
Analysis of subgroups based on similar injury mechanisms only produced apparent trends in terms of pulmonary mechanics and dead-space ventilation. The median CRS of our subjects ranged from 18 to 40 mL/cmH2O (Table 3). Using the reported average CRS for ARDS (30–34 cmH2O) (10), six groups fell below this range whereas 3 groups were above. The lowest CRS was observed in toxicity, hyper-immune response and hepatic failure whereas the highest CRS was found in neurologic injury, drug overdose and idiopathic subgroups (Figure 1).
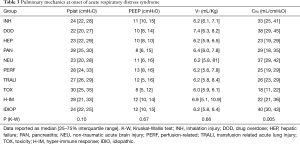
Full table
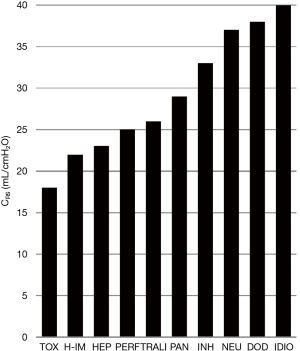
VD/VT, was extraordinarily high in ARDS associated with hepatic failure and lowest in pancreatitis (Table 4, Figure 2). All subgroups met moderate ARDS criteria based on median PaO2/FiO2 wherein eight of 10 groups had a median PaO2/FiO2 of 103 to 120 mmHg. The hyper-immune response and TRALI groups had median values of 154 and 163 mmHg respectively. Acidosis was most pronounced in those with hepatic failure and hyper-immune response.
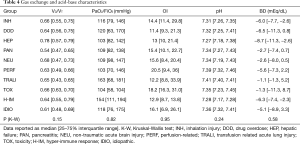
Full table
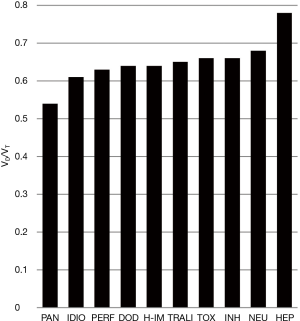
For the entire cohort, only VD/VT was statistically different between non-survivors and survivors: 0.66 (0.57 to 0.78) vs. 0.59 (0.51 to 0.68), P=0.012. Moreover, in the six subgroups with an n≥8 subjects no consistent pattern emerged regarding differences between survivors and non-survivors for CRS, PaO2/FiO2, OI, pH or BD (data not shown) Only VD/VT demonstrated a consistent pattern of higher values among non-survivors (Figure 3).
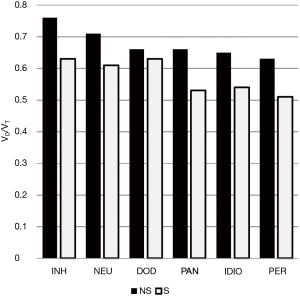
For the three other cases, ARDS associated with cutaneous burns (without inhalation injury) had the lowest VD/VT (0.39) and highest PaO2/FiO2 (242 mmHg) along with a mid-range CRS (34 mL/cmH2O) compared to the entire cohort. In contrast, the malaria case was characterized by both severely impaired gas exchange and chest mechanics (VD/VT: 0.68, PaO2/FiO2: 73 mmHg, CRS: 25 mL/cmH2O measured on PEEP of 14 mL/cmH2O and FiO2 of 1).
Discussion
Our main finding was that subjects with uncommon ARDS etiologies are characterized primarily by indirect injury mechanisms; however, differences between these categories were not significant in regards to either chest mechanics or gas exchange. Yet, when the entire cohort was analyzed VD/VT was the only pulmonary-specific variable associated with mortality.
Our main hypothesis that uncommon etiologies of ARDS from direct causes would have higher VD/VT was not borne out by the data which demonstrated substantial diversity in the severity of carbon dioxide excretion dysfunction. In part this may have resulted from an imbalance between the two groups. However, our results suggest that even within the classification of indirect lung injury there appears to be marked variability greater than would be anticipated by its underlying mechanism (i.e., primary endothelial injury). For example, hepatic failure was associated with inordinately high VD/VT while pancreatitis had the lowest VD/VT similar to that in trauma-associated ARDS (4). This variability likely represents complex contributing mechanisms, as well as ambiguity in clinical presentation that are discussed below.
The tendency towards inordinately high VD/VT in hepatic failure (compared to our subjects with direct lung injury) probably reflects the contribution of three factors: first, pulmonary arteriovenous shunting from the vasodilatory effects of elevated ammonia (15); second, the contribution of “shunt dead-space” (from ascites-induced compression of dorsocaudal lung regions) (16); third, endothelial injury from disseminated intravascular coagulation documented in three cases (17). These subjects also had particularly low CRS in part from ascites, while the extraordinarily high mortality (86%) is consistent with the established literature (15,18). Although it is particularly difficult to exclude sepsis in those with hepatic failure, we noted that VD/VT in our subjects (0.78) was substantially higher than that reported in non-pulmonary sepsis (0.61) (4).
Likewise, acute pulmonary toxicity was characterized by higher VD/VT similar to that reported in direct lung injury from inhalation injuries as well as pneumonia and aspiration (4), albeit from what appears to be indirect mechanisms. Bleomycin toxicity is an established model used in preclinical studies (19) that initially results in both microvascular endothelial injury and diffuse interstitial edema (that eventually injures the alveolar epithelium) (19,20). The progressive cellular damage and resulting fibrosis from bleomycin toxicity is considered dissimilar from other forms of acute lung injury. Radiation-associated ARDS is similar to hyperoxic acute lung injury as it results in the generation of radical oxygen species that overwhelms the body’s antioxidant defense mechanisms (21)
Our naming a hyper-immune associated ARDS subgroup was based upon other’s hypotheses that this form of lung injury is caused by a proinflammatory mediator burst both during tumor lysis syndrome (22) and immune reconstitution inflammatory syndrome (23), whereas hypersensitivity or cell-mediated immune response may play a role in toxic epidermal necrolysis (24). Yet, in these presentations of ARDS, other occult mechanisms may be present. For example, toxic epidermal necrolysis often involves upper airway and bronchial mucosa injury increasing the possibility of aspiration or pneumonia.
Likewise, VD/VT in non-traumatic neurologic injury was similar to that recently reported in aspiration (0.68) compared to subjects with isolated traumatic brain injury (0.55) (4). Therefore, the fact that aspiration was not witnessed in these subjects (nor suggested by inspection of tracheal aspirates following intubation) does not exclude the possibility that it might have occurred.
ARDS associated with acute pancreatitis was noteworthy for its similarity with trauma-associated ARDS, namely a tendency towards lower VD/VT and mortality (0.54 and 19% respectively in our subjects). Acute lung injury in pancreatitis is believed to be caused by a two-hit mechanism: an initial pronounced systemic inflammatory response, followed by secondary injury from the systemic release of digestive enzymes that causes cell necrosis and damages pulmonary surfactant (25,26).
Perfusion associated acute lung injury from hemorrhagic shock is complicated as all cases required initiation of our mass transfusion protocol These subjects likely suffered ischemia-reperfusion injury as well. Early studies of ARDS following hemorrhagic shock implicated coagulopathy as the primary mechanism (27) with fibrinogen degradation products such as fragment D directly implicated in the pathogenesis (28,29). Additional pulmonary insults are associated with the sequelae of shock: most notably systemic inflammatory response due to macrophage activation from bacterial translocation occurring in the gut (30).
In addition, ischemia-reperfusion produces radical oxygen and nitrogen species causing injury both in directly affected organs as well as distant organs (31). The primary mechanism in TRALI appears to be neutrophil-mediated, along with a postulated “two-hit model” (i.e., a baseline proinflammatory state that activates the pulmonary endothelium followed by blood products that induce neutrophil activation (32). Interestingly, when comparing our subjects with hemorrhagic shock/reperfusion injury to the three cases of isolated TRALI both median CRS (25 vs. 26 mL/cmH2O respectively) and VD/VT (0.63 and 0.65) were similar.
Despite considerable differences in pathophysiology between smoke inhalation and near drowning, there was very little difference in CRS (34 vs. 32 mL/cmH2O respectively) and VD/VT (0.65 vs. 0.63 respectively) between injury mechanisms. Smoke inhalation involves both thermal injury to the bronchial mucosa and toxic gas-induced injury to the alveolar capillary membrane, in addition to peripheral airway obstruction from fibrin clots. Pronounced increases in bronchial blood flow (approximately 8-fold) increases anatomic shunting and vascular engorgement (through bronchopulmonary anastomoses), whereas loss of barrier integrity causes hyperpermeability and surfactant deactivation (33). Our two cases of near-drowning involved both seawater and fresh water aspiration. In the former, hyperosmolarity, low temperature and high pathogen content of the aspirated fluid results in both an osmotic gradient across a damaged alveolar-capillary membrane and surfactant inactivation (34,35). Freshwater aspiration is distinguished by less severe inflammatory mediator release and milder lung injury (36).
The obvious limitation of this study is that diverse, less common ARDS etiologies restricts the number of cases available for analysis. In consequence the ability to construct statistically meaningful, descriptions that advance our knowledge is stymied. In addition, from a pragmatic standpoint the infrequent occurrences make studying these etiologies impractical. Thus, our understanding of uncommon ARDS etiologies will remain speculative. This study was an initial attempt to explore this catch-all ARDS category and begin describing the apparent multiplicity of pulmonary mechanics and gas exchange derangements.
A further limitation is inter-observer variability and physiologic confounders that can affect measurement consistency when using data generated during routine clinical practice. For example, CRS is affected by the level of PEEP, VT size and fluid balance, none of which are controlled in a retrospective observational study such as ours. However, we found no correlation between VT and CRS (r=−0.07; P=0.56) and only a weak correlation between PEEP and CRS (r=0.17; P=0.12). Moreover, previous studies have shown a remarkable consistently in repeated measurements of VD/VT and CRS over the first 3 days of ARDS with mean variability of approximately 0.02 and 1–2 mL/cmH2O respectively (37-39).
Conclusions
In summary, this diverse sample of 86 subjects with less common presentations of ARDS exhibited marked variability in the distribution of CRS and VD/VT between different etiologies. The relative rarity of these cases limited our findings to describing apparent differences between individual etiologies. In many instances this was made more ambiguous given the possibility of other mechanisms contributing to derangements in both pulmonary mechanics and gas exchange. Despite these limitations, elevated VD/VT was statistically higher in non-survivors compared to survivors.
Acknowledgements
None.
Footnote
Conflicts of Interest: Mr. Kallet and Dr. Matthay have previous received research support from Philips/Respironics for prior studies involving volumetric capnography. The other authors have no conflicts of interest to declare.
Ethical Statement: Use of our quality assurance data for research purposes was approved by the University of California, San Francisco’s institutional review board for human subject protection with waiver of consent granted (IRB#16-19189).
References
- Villar J. What is the acute respiratory distress syndrome? Respir Care 2011;56:1539-45. [Crossref] [PubMed]
- Roupie E, Lepage E, Wysocki M, et al. Prevalence, etiologies and outcome of the acute respiratory distress syndrome among hypoxemic ventilated patients. Intensive Care Med 1999;25:920-9. [Crossref] [PubMed]
- Manzano F, Yuste E, Colmenero M, et al. Incidence of acute respiratory distress syndrome and its relation to age. J Crit Care 2005;20:274-80. [Crossref] [PubMed]
- Kallet RH, Zhuo H, Ho K, et al. Lung injury etiology and other factors influencing the relationship between dead-space fraction and mortality in ARDS. Respir Care 2017;62:1241-8. [Crossref] [PubMed]
- Acute Respiratory Distress Syndrome Network, Brower RG, Matthay MA, et al. A trial of traditional tidal volume versus lower tidal volume ventilation in acute lung injury and acute respiratory distress syndrome. N Engl J Med 2000;342:1301-8. [Crossref] [PubMed]
- Bernard GR, Artigas A, Brigham KL, et al. Report of the American-European consensus conference on acute respiratory distress syndrome: Definitions mechanisms, relevant outcomes, and clinical trials coordination. Am J Respir Crit Care Med 1994;149:818-24. [Crossref] [PubMed]
- ARDS Definition Task Force, Ranieri VM, Rubenfeld GD, et al. Acute Respiratory Distress Syndrome: The Berlin Definition. JAMA 2012;307:2526-33. [PubMed]
- Enghoff H. Volumen inefficax: Bemerkungen zur frage des schädlichen raumes. Upsala Lakareforen Forh 1938;44:191-218.
- Kallet RH, Daniel B, Garcia O, et al. Accuracy of physiologic dead space measurements in patients with acute respiratory distress syndrome using volumetric capnography: Comparison with the metabolic monitor method. Respir Care 2005;50:462-7. [PubMed]
- Kallet RH, Katz JA. Respiratory system mechanics in acute respiratory distress syndrome. Respir Care Clin N Am 2003;9:297-319. [Crossref] [PubMed]
- Ortiz RM, Cilley RE, Bartlett RH. Extracorporeal membrane oxygenation in pediatric respiratory failure. Pediatr Clin North Am 1987;34:39-46. [Crossref] [PubMed]
- Murray JF, Matthay MA, Luce JM, et al. An expanded definition of the adult respiratory distress syndrome. Am Rev Respir Dis 1988;138:720-3. [Crossref] [PubMed]
- Le Gall JR, Lemeshow S, Saulnier F. A new Simplified Acute Physiology Score (SAPS II) based on a European-North American multicenter study. JAMA 1993;270:2957-63. [Crossref] [PubMed]
- Knaus WA, Draper EA, Wagner DP, et al. Apache II. A severity of disease classification system. Crit Care Med 1985;13:818-29. [Crossref] [PubMed]
- Siegel JH, Goldwyn RM, Farrell EJ, et al. Hyperdynamic states and the physiologic determinants of survival in patients with cirrhosis and portal hypertension. Arch Surg 1974;108:282-92. [Crossref] [PubMed]
- Suter PM, Fairley HB, Isenberg MD. Optimum end-expiratory airway pressure in patients with acute pulmonary failure. N Engl J Med 1975;292:284-9. [Crossref] [PubMed]
- Matuschak GM, Shaw BW. Adult respiratory distress syndrome associated with acute liver allograft rejection: resolution following hepatic retransplantation. Crit Care Med 1987;15:878-81. [Crossref] [PubMed]
- Schwartz DB, Bone RC, Balk RA, et al. Hepatic dysfunction in adult respiratory distress syndrome. Chest 1989;95:871-5. [Crossref] [PubMed]
- Matute-Bello G, Frevert CW, Martin TR. Animal models of acute lung injury. Am J Physiol Lung Cell Mol Physiol 2008;295:L379-99. [Crossref] [PubMed]
- Adamson IY, Bowden DH. The pathogenesis of bleomycin-induced pulmonary fibrosis in mice. Am J Pathol 1974;77:185-97. [PubMed]
- Kallet RH, Matthay MA. Hyperoxic acute lung injury. Respir Care 2013;58:123-41. [Crossref] [PubMed]
- Marenco JP, Nervi A, White AC. ARDS associated with tumor lysis syndrome in a patient with Non-Hodgkin’s Lymphoma. Chest 1998;113:550-2. [Crossref] [PubMed]
- Sharma SK, Soneja M. HIV and immune reconstitution inflammatory syndrome. Indian J Med Res 2011;134:866-77. [Crossref] [PubMed]
- Timsit JF, Mion G, Rouyer N, et al. Bronchopulmonary distress associated with toxic epidermal necrolysis. Intensive Care Med 1992;18:42-4. [Crossref] [PubMed]
- Pastor CM, Matthay MA, Frossard JL. Pancreatitis-associated acute lung injury. New insights. Chest 2003;124:2341-51. [Crossref] [PubMed]
- Elder AS, Saccone GT, Dixon DL. Lung injury in acute pancreatitis: mechanisms underlying augmented secondary injury. Pancreatology 2012;12:49-56. [Crossref] [PubMed]
- Blaisdell FW, Stallone RJ. The mechanism of pulmonary damage following traumatic shock. Surg Gynecol Obstet 1970;130:15-22. [PubMed]
- Luterman A, Manwaring D, Curreri PW. The role of fibrinogen degradation products in the pathogenesis of the respiratory distress syndrome. Surgery 1977;82:703-9. [PubMed]
- Manwaring D, Curreri PW. The role of platelet aggregation and release in fragment D-induced pulmonary dysfunction. Ann Surg 1980;192:103-7. [Crossref] [PubMed]
- Angele MK, Schneider CP, Chaudry IH. Bench-to-bedside review: Latest results in hemorrhagic shock. Crit Care 2008;12:218. [Crossref] [PubMed]
- Halladin NL. Oxidative and inflammatory biomarkers of ischemia and reperfusion injuries. Dan Med J 2015;62:B5054. [PubMed]
- Alvarez P, Carrasco R, Romero-Dapueto C, et al. Transfusion-related acute lung injury (TRALI): current concepts. Open Respir Med J 2015;9:92-6. [Crossref] [PubMed]
- Enkhbaatar P, Traber DL. Patholphysiology of acute lung injury in combined burn and smoke inhalation injury. Clin Sci 2004;107:137-43. [Crossref] [PubMed]
- Jin F, Li C. Seawater-drowning-induced acute lung injury: from molecular mechanisms to potential treatments. Exp Ther Med 2017;13:2591-8. [Crossref] [PubMed]
- Folkesson HG, Kheradmand F, Matthay MA. The effect of salt water on alveolar epithelial barrier function. Am J Respir Crit Care Med 1994;150:1555-63. [Crossref] [PubMed]
- Rui M, Duan YY, Zhang XH, et al. Differences between seawater- and freshwater-induced lung injuries. Zhongguo Wei Zhong Bing Ji Jiu Yi Xue 2009;21:416-20. [PubMed]
- Kallet RH, Alonso JA, Pittet JP, et al. Prognostic value of the pulmonary dead-space fraction during the first six days of acute respiratory distress syndrome. Respir Care 2004;49:1008-14. [PubMed]
- Kallet RH, Zhao H, Liu KD, et al. The association between physiologic dead-space fraction and mortality in patients with acute respiratory distress syndrome enrolled into prospective, multi-centered clinical trial. Respir Care 2014;59:1611-8. [Crossref] [PubMed]
- Seeley EJ, McAuley DF, Eisner M, et al. Decreased respiratory system compliance on day 6 of mechanical ventilation is a predictor of death in patients with established acute lung injury. Respir Res 2011;12:52. [Crossref] [PubMed]