Neurokinin 1 receptor promotes rat airway smooth muscle cell migration in asthmatic airway remodelling by enhancing tubulin expression
Introduction
In recent years, the prevalence and mortality of bronchial asthma have increased. Bronchial asthma is a chronic respiratory disease that seriously affects human health, characterized by airway inflammation, hyper-responsiveness, reversible obstruction, and remodelling. Despite the use of standard anti-asthma agents, approximately 55% of asthma patients still have poorly controlled conditions, reduced quality of life, and increased asthma-related hospitalization and mortality rates, resulting in heavy healthcare and socio-economic burdens. Some patients with poorly controlled conditions are diagnosed incorrectly or have other diseases along with asthma, requiring more detailed examination and confirmation. Additionally, other patients experience airway remodelling, which induces airway hyper-responsiveness and chronic asthma (1-4).
Airway remodelling refers to changes in the airway wall structure of asthma patients resulting from airway inflammation, tissue damage, and subsequent abnormal repair. As airway remodelling is the main cause of airway hyper-responsiveness and asthma chronicity, substantial research efforts have focused on elucidating the mechanism of airway remodelling in asthma to identify potential targets for treatment (5). Many imaging techniques, including chest computed tomography, bronchial ultrasound, and transbronchial needle aspiration (6-9), have been used to evaluate bronchial wall layers to study airway remodelling. The main pathological changes include subepithelial fibrosis, airway smooth muscle cell (ASMC) proliferation and migration, mucus hyperplasia, and angiogenesis (10,11). However, changes in ASMCs appear to be the primary event that contributes to airway remodelling (12) along with constriction of smooth muscle cell microtubules (13,14). ASMC migration occurs when smooth muscle precursor cells migrate from the non-smooth muscle area into airway smooth muscle bundles and differentiate into smooth muscle cells. One of the main factors contributing to hyperplasia of the smooth muscle may be the differentiation of some fibroblasts into ASMCs in the subepithelial. These fibroblasts migrate in response to certain chemotactic factors in the circulation. Therefore, it is thought that the migration of ASMCs is an important component of long-term asthma-induced pathological changes, such as tracheal smooth muscle hyperplasia and remodelling (15). However, the specific mechanisms and factors that contribute to this process remain unclear.
We previously showed a potential role for neurokinin 1 receptor (NK1R) in airway remodelling, since treatment of airway remodelling-induced ASMCs with NK1R antagonists promoted cell migration. NK1R is a G protein-coupled receptor with high affinity for Substance P, a major neuropeptide, which is a key mediator of active communication between the nervous and immune systems. Moreover, the neuropeptide is involved in neurogenic inflammation and exerts pro-inflammatory effects on various inflammatory cells (16,17). Substance P induces increased mRNA and protein expression levels of the chemokines MIP-1alpha/CCL3 and MIP-2/CXCL2 in neutrophils, as well as triggering IκBα degradation and changes in the binding activity and nuclear translocation of NF-κB p65 in neutrophils (18). Moreover, substance P induces increased mRNA expression levels and secretion of the chemokine CXCL8 and HDC in human umbilical cord blood mast cells, further supporting a role in promoting neurogenic inflammation (19).
In the respiratory tract, NK1R can be found in the airway smooth muscle, submucosal gland, and vascular endothelium. Some inflammatory cells also express NK1R, where it plays a role in the regulation of pain transmission, endocrine and paracrine effects, vasodilatation, and cell proliferation (20-25). In addition, recent studies have shown that activation of NK1 receptors can block HHK1-induced breast cancer cell migration (26). However, the effect of NK-1R on the migration of ASMCs has received less attention. Although our previous work points to such a role, the mechanism underlying these effects has not yet been adequately explored (27).
To examine this mechanism, we investigated the effects of a NK1R antagonist (WIN62577) on the migration of ASMCs and the expression of NK1R and alpha-tubulin in airway remodelling using young rats with asthma.
Methods
Establishment of a childhood asthma airway remodelling rat model
Specific pathogen-free-grade young female Sprague-Dawley rats from China Medical University Experimental Animal Centre weighing 60–80 g were used. The study was conducted with approval from the Ethics Committee at Shengjing Hospital of China Medical University. Each group included 8 SD rats.
The airway remodelling and control groups both consisted of 8 SD rats. On the first and eighth days, rats in the asthma airway remodelling group were intraperitoneally injected with 1 mL of an antigen-sensitive mixture (100 mg ovalbumin, 100 mg aluminium hydroxide, and 5×109 inactivated Bordetella pertussis cells). In the control group, rats were injected with an equal volume of normal saline. As of the 15th day, rats were individually placed in a closed container (20 cm × 20 cm × 20 cm) and the model rats were treated with 1% ovalbumin ultrasonic inhalation for 30 min three times per week for 8 weeks. In the control group, saline (instead of ovalbumin) was administered for 8 weeks.
Primary culture of rat ASMCs
After routine disinfection and anaesthesia, rats were sacrificed by drawing blood from the left ventricle. The rat trachea was isolated aseptically, the outer membrane was peeled carefully, and the intima was removed. The tracheal smooth muscle was cut off, and the tissue was then cut into pieces and mixed with 0.1% trypsin. Treated tissue was shaken in a water bath at 37 °C for 10 min and centrifuged at 1,000 rpm and 800 ´g for 5 min. The supernatant was discarded, and 0.1% collagenase type IV (GIBCO Reagents Inc., USA) was added. The tissue was shaken again in the water bath at 37 °C for 30 min, centrifuged at 1,000 rpm and 800 ´g for 5 min, and the supernatant was then discarded. The reaction was stopped by adding Dulbecco’s modified Eagle medium (DMEM) supplemented with 10% foetal bovine serum (FBS) (HyClone Laboratories, Inc., Logan, UT, USA), gently swirled, and filtered through a 100-mm mesh cell sieve. Cells were inoculated into a 50-mL culture flask and incubated at 37 °C for 1 h. ASMCs were purified based on the rapid adherence characteristics of fibroblasts. After the cells covered the bottom of the bottle, they were passaged at a ratio of 1:2 and the third generation of cells was collected for experiments.
Experimental cells were divided into the following three groups: (I) control group; (II) asthma airway remodelling group; and (III) NK1R-specific inhibitor WIN62577 intervention group. In the NK1R blocking group, before the migration experiments, cells in the upper chamber in the asthmatic airway remodelling group were pre-treated with various concentrations of WIN62577 (Sigma, St. Louis, MO, USA; 10−11, 10−10, 10−9, 10−8 and 10−7 mol/L) for 30 min. To test the mRNA and protein expression levels of α-tubulin, ASMCs from the asthmatic airway remodelling group were pretreated with 10−8 mol/L WIN62577 for 24 h.
Transwell chamber detection of ASMC migration
For the migration assay, ASMCs were collected at passage 3–5. When ASMCs grew to 80% confluence, cells were digested with 0.25% trypsin and counted. The cell density was adjusted to 1×105 cells/mL with DMEM containing 10% FBS, and 100 mL of each cell suspension was added to the upper chamber and 600 mL DMEM with 10% FBS was added to the lower chamber of the transwell (Corning Co., Corning, NY, USA). The chamber was incubated at 37 °C for 24 h, after which the filter was collected and non-permeable cells were gently wiped on the upward side of the microporous membrane with a cotton swab. ASMCs below the membrane were fixed with 4% paraformaldehyde for 20 min, stained with haematoxylin for 10 min, incubated with xylene for 1 min, and mounted with neutral gum. The number of cells in five visual fields was counted randomly under an inverted microscope (400×) and averaged.
Immunofluorescence staining for NK1R in ASMCs
The logarithmic growth-phase cells were digested to prepare a single-cell suspension, and the density was appropriately adjusted before inoculation in a six-well plate cover glass. Culturing was carried out overnight at 37 °C in a CO2 incubator. After cells adhered to the wall, they were fixed with 4% paraformaldehyde. Cells were treated with 3 M H2O2 at room temperature (21–25 °C) for 10 min, followed by 3 g/L triton 100 at 37 °C for 10 min, and blocked with 3% sheep serum at room temperature for 15 min. Cells were then incubated with rabbit anti-rat NK1R or α-tubulin antibody (1:100; Santa Cruz Technology, Santa Cruz, CA, USA) and placed in a wet box at 4 °C overnight. Fluorescein isothiocyanate (FITC)-labelled goat anti-rabbit IgG was added and incubated for 30 min at 37 °C. Subsequently, cells were incubated with 5 µg/mL 6-acetyl-2-phenylindole (DAPI; Sigma, St. Louis, MO, USA) at room temperature for 5 min. Cells were sealed with 50% glycerol and observed under a fluorescence microscope. Each sample was washed with phosphate-buffered saline (PBS) between steps. The negative control was set up using PBS instead of a primary antibody.
Real-time qPCR detection ofNK1R and α-tubulin mRNA expression
Primers for qPCR were synthesized by Invitrogen: NK1R upstream 5'-ACC AAC ACC TCT GAG TCT AA-3', downstream 5'-TGG TCA CTG TCC TCA TTC T-3' (amplified fragment length, 154 bp); α-tubulin upstream 5'-CCACTCATTCCCTCCTTGAA-3', downstream 5'-ATGGCTCCATCAAACCTCAG-3' (amplified fragment length, 200 bp); and GAPDH upstream 5'-GCA CCG TCA AGG CTG AGA AC-3', downstream 5'-ATG GTG GTG AAG ACG CCA GT-3' (amplified fragment length, 142 bp). RNA was extracted with RNAisoTM Plus and qPCR was conducted using a kit from TaKaRa Co. (Mountain View, CA, USA). SYBR Green I fluorescent dye chimerism was used to prepare a standard curve of target genes (NK1R, α-tubulin) and a housekeeping gene (GAPDH) for quantification. The relative expression levels of the test gene were calculated as follows: (test gene copy number)/(GAPDH gene copy number).
Western blot detection of NK1R and α-tubulin protein expression
Whole proteins were extracted using a kit from KGI Biological Technology Development Co. (Nanjing, China), and the bicinchoninic acid assay was used for protein quantification. Proteins were separated with 10% sodium dodecyl sulphate-polyacrylamide gel electrophoresis at a stacking gel voltage of 80 V and separation gel voltage of 120 V; next, the gel was transferred with a constant current of 280 mA for 50 min. Rabbit anti-rat primary antibody (1:400) and alkaline phosphatase-labelled goat anti-rabbit secondary antibody (1:2,000) were used for western blotting, and an enzymatic method was used to develop the blots. The SeeBlueTM protein marker was purchased from Invitrogen (Grand Island, NY, USA). The grey value of each protein electrophoresis band was recorded and analysed quantitatively with FlourChem V 2.0 gel imaging analysis software (USA) as follows: protein content = (sample protein grey value)/(β-actin grey value of the same sample).
Statistical analysis
All data were expressed as means ± SD and analysed with the Statistical Package for the Social Sciences (SPSS) software (version 13.0). Analysis of variance (ANOVA) was used to compare differences between continuous variables. The multiple-comparison post-hoc test [Student-Newman-Keul (SNK)] and least significant difference (LSD) test were used for experiments in which more than 2 groups were compared. A value of P<0.05 was considered to indicate a statistically significant difference.
Results
Expression of NK1R in ASMCs
By fluorescence microscopy, NK1R was observed to be mainly distributed in the cytoplasm and cell membrane of ASMCs. The overall green fluorescent intensity of cells from the airway remodelling group was higher than that of the control group (Figure 1). Moreover, the expression levels of NK1R mRNA and protein in the asthmatic airway remodelling group were significantly higher than those of the control group (P<0.05), but were significantly decreased after treatment with the NK1R antagonist (P<0.01) (Table 1).
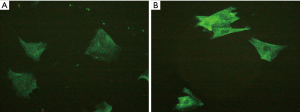
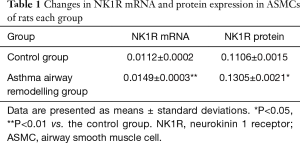
Full table
Changes in the numbers of migrating ASMCs
The number of ASMCs migrating in the control group was approximately half that detected in asthmatic airway remodelling group (15.86±2.62 vs. 32.14±3.74; P<0.01). WIN62577 inhibited the migration of ASMCs in the asthmatic airway remodelling group in a dose-dependent manner, with peak inhibition detected at 10−8 mol/L (Figures 2 and 3).
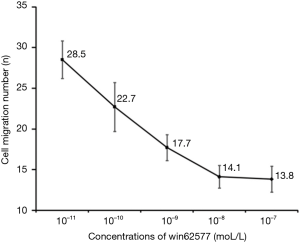
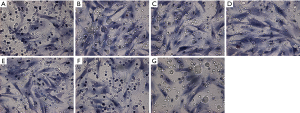
Distribution and morphology of α-tubulin in rat ASMCs
Immunofluorescence staining for α-tubulin showed a high-density microtubule structure in the cytoplasm, demonstrating a silk-like and radial-like distribution from the nucleus centre to the periphery. The fluorescence distribution was uneven, and the fluorescent signal intensity and density of microtubules in the asthmatic airway remodelling group were significantly greater than those of the control group. However, the fluorescence signal of microtubules in the 10−8 mol/L WIN62577 intervention group was obviously lower, the network structure was significantly reduced, and the number of fragments increased (Figure 4).
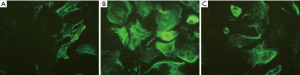
mRNA and protein expression levels of α-tubulin in rat ASMCs
The expression levels of α-tubulin mRNA and protein in the airway remodelling group were significantly higher than those of the control group (P<0.01). However, the expression levels of α-tubulin in ASMCs of the airway remodelling group pretreated with 10−8 mol/L WIN62577 significantly decreased (P<0.05) (Table 2).
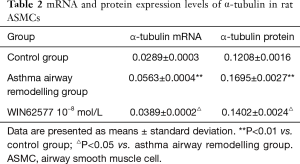
Full table
Discussion
We previously demonstrated the importance of ASMC migration in airway remodelling, which could stimulate with external factors in vitro to induce an airway remodelling status. In this present study, we confirmed these findings in primary cultured ASMCs from an asthma airway remodelling rat model, which more closely mimics the condition of airway remodelling in asthma patients. Thus, ASMC migration may be an important factor that can promote or aggravate asthma airway remodelling.
However, our current understanding of the underlying migration mechanism remains incomplete. The general view is that the original smooth muscle cells in the airways participate in asthma airway remodelling by undergoing a series of changes. The general cell migration mechanism is closely related to G protein-coupled receptors, receptor tyrosine kinases, and mitogen-activated protein kinase signalling pathways. These signal modules control the remodelling of multiple key effector proteins, including actin, myosin, and tubulin, and promote cell motility through chemical cues, matrix adhesion, or matrix stiffness gradients. The upstream factors that promote these changes are transforming growth factor-β, vascular endothelial growth factor, interleukin (IL)-1β, IL-17, and many others (28-32). Gaining a detailed understanding of the mechanism of the different mediators that play roles in ASMC migration, and the types of self-alterations in smooth muscle cells that lead to migration will help to inform new strategies for the individual treatment of asthmatic airway remodelling. The purpose of our study was to investigate whether the upstream molecule NK1R is involved in the migration of ASMCs and whether the tracheal smooth muscle undergoes remodelling of microtubule cytoskeletal proteins during airway remodelling.
We found that NK1R was expressed in the membrane and cytoplasm of rat ASMCs, and increased in ASMCs of asthmatic rats with airway remodelling. Moreover, administration of the NK1R antagonist WIN62577 blocked NK1R expression and inhibited the migration of ASMCs in the asthmatic airway remodelling rats in a dose-dependent manner.
The cytoskeleton is a fibrillar matrix of eukaryotic cells, and fibrous structures in the cells can be found in reticular, bundle, band, or other forms. The cytoskeleton consists of microfilaments, microtubules, and medium fibres, with distributions that are highly coordinated. They are connected to the nucleus, plasma membrane, and organelles, forming a cytoskeleton and motor coordination system to maintain cell morphology and exercise motor functions, with important roles in signal transmission (33-36).
We also found that the mRNA and protein expression levels of microtubule α-tubulin increased in the asthma airway remodelling rats, indicating a change of microtubule structure in the ASMCs during airway remodelling, which would affect ASMC migration. Microtubules are hollow cylindrical tubular fibres with a diameter of ~25 nm, and are mainly composed of α and β tubulin, with dimers composed of two subunits α and β. They are also a type of guanosine triphosphate-binding protein with significant amino acid sequence homology to G proteins in signal transduction systems and have similar functional properties. Microtubules are involved in cellular motion, cytoplasmic transport, chromosomal motion, and mitotic process (37-41).
Moreover, the expression of α-tubulin in the ASMCs of the asthmatic airway remodelling group significantly decreased after pretreatment with 10−8 mol/L WIN62577, indicating that the inhibition of cytoskeletal remodelling induced by the NK1R antagonist may reduce cell migration and, thus, reduce the occurrence and development of airway remodelling.
In this study, only the expression of the α-tubulin was measured, but the cytoskeleton consists of many components. Therefore, further studies are needed to explore the relationship between other cytoskeleton components and the migration of ASMCs.
In summary, our findings indicate that NK1R may be a novel target for the prevention and control of airway remodelling in asthma. Our previous studies have indicated that montelukast can reduce NK1R expression in asthmatic rats with airway remodelling (42). Thus, it will be important to compare the efficacy of NK1R antagonists with classic drugs, such as montelukast, in airway remodelling in future studies.
Acknowledgements
None.
Footnote
Conflicts of Interest: The authors have no conflicts of interest to declare.
Ethical Statement: The study was approved by the Ethics Committee at Shengjing Hospital of China Medical University.
References
- Zhu D, Zhang C, Shen H, et al. Breaking through restricting bottleneck for better asthma control. J Transl Int Med 2017;5:192-93. [Crossref] [PubMed]
- Wildhaber J, Carroll WD, Brand PL. Global impact of asthma on children and adolescents' daily lives: the room to breathe survey. Pediatr Pulmonol 2012;47:346-57. [Crossref] [PubMed]
- Yang X, Wang Y, Zhao S, et al. Long-term exposure to low-dose Haemophilus influenzae during allergic airway disease drives a steroid-resistant neutrophilic inflammation and promotes airway remodeling. Oncotarget 2018;9:24898-913. [Crossref] [PubMed]
- Louis R, Demarche S, Schleich F. A historical perspective: Are inhaled corticoids sufficient to control asthma? J Transl Int Med 2015;3:113-5. [Crossref] [PubMed]
- Tung HY, Li E, Landers C, et al. Advances and Evolving Concepts in Allergic Asthma. Semin Respir Crit Care Med 2018;39:64-81. [Crossref] [PubMed]
- Dietrich CF, Jenssen C, Herth FJ. Endobronchial ultrasound elastography. Endosc Ultrasound 2016;5:233-8. [Crossref] [PubMed]
- Aswanetmanee P, Limsuwat C, Kabach M, et al. The role of sedation in endobronchial ultrasound-guided transbronchial needle aspiration: Systematic review. Endosc Ultrasound 2016;5:300-6. [Crossref] [PubMed]
- Stellari F, Sala A, Ruscitti F, et al. Monitoring inflammation and airway remodeling by fluorescence molecular tomography in a chronic asthma model. J Transl Med 2015;13:336. [Crossref] [PubMed]
- Dietrich CF. Contrast-enhanced endobronchial ultrasound: Potential value of a new method. Endosc Ultrasound 2017;6:43-8. [Crossref] [PubMed]
- Ye WJ, Xu WG, Guo XJ, et al. Differences in airway remodeling and airway inflammation among moderate-severe asthma clinical phenotypes. J Thorac Dis 2017;9:2904-14. [Crossref] [PubMed]
- van den Berg MP, Meurs H, Gosens R. Targeting arginase and nitric oxide metabolism in chronic airway diseases and their co-morbidities. Curr Opin Pharmacol 2018;40:126-33. [Crossref] [PubMed]
- Salter B, Pray C, Radford K, et al. Regulation of human airway smooth muscle cell migration and relevance to asthma. Respir Res 2017;18:156. [Crossref] [PubMed]
- Wei B, Shang YX, Li M, et al. Cytoskeleton changes of airway smooth muscle cells in juvenile rats with airway remodeling in asthma and the RhoA/ROCK signaling pathway mechanism. Genet Mol Res 2014;13:559-69. [Crossref] [PubMed]
- Tang DD, Gerlach BD. The roles and regulation of the actin cytoskeleton, intermediate filaments and microtubules in smooth muscle cell migration. Respir Res 2017;18:54. [Crossref] [PubMed]
- Lee CC, Wang CN, Lee YL, et al. High mobility group box 1 induced human lung myofibroblasts differentiation and enhanced migration by activation of MMP-9. PLoS One 2015;10. [Crossref] [PubMed]
- McCormack RJ, Hart RP, Ganea D. Expression of NK-1 receptor mRNA in murine T lymphocytes. Neuroimmunomodulation 1996;3:35-46. [Crossref] [PubMed]
- O'Connor TM, O'Connell J, O'Brien DI, et al. The role of substance P in inflammatory disease. J Cell Physiol 2004;201:167-80. [Crossref] [PubMed]
- Sun J, Ramnath RD, Bhatia M. Neuropeptide substance P upregulates chemokine and chemokine receptor expression in primary mouse neutrophils. Am J Physiol Cell Physiol 2007;293:C696-704. [Crossref] [PubMed]
- Castellani ML, Ciampoli C, Felaco M, et al. Neuropeptide substance P induces mRNA expression and secretion of CXCL8 chemokine, and HDC in human umbilical cord blood mast cells. Clin Invest Med 2008;31:E362-72. [Crossref] [PubMed]
- Mantyh PW. Neurobiology of substance P and the NK1 receptor. J Clin Psychiatry 2002;63:6-10. [PubMed]
- Bright FM, Byard RW, Vink R, et al. Normative distribution of substance P and its tachykinin neurokinin-1 receptor in the medullary serotonergic network of the human infant during postnatal development. Brain Res Bull 2018;137:319-28. [Crossref] [PubMed]
- Leonard AV, Manavis J, Blumbergs PC, et al. Changes in substance P and NK1 receptor immunohistochemistry following human spinal cord injury. Spinal Cord 2014;52:17-23. [Crossref] [PubMed]
- Gozal D, Kim J, Bhattacharjee R, et al. Substance P and neurokinin 1 receptors as potential therapeutic targets in children with OSA. Chest 2014;145:1039-45. [Crossref] [PubMed]
- Zhang K, Chen QT, Li JH, et al. The expression of tachykinin receptors in the human lower esophageal sphincter. Eur J Pharmacol 2016;774:144-9. [Crossref] [PubMed]
- Nederpelt I, Bleeker D, Tuijt B, et al. Kinetic binding and activation profiles of endogenous tachykinins targeting the NK1 receptor. Biochem Pharmacol 2016;118:88-95. [Crossref] [PubMed]
- Li J, Zeng Q, Zhang Y, et al. Neurokinin-1 receptor mediated breast cancer cell migration by increased expression of MMP-2 and MMP-14. Eur J Cell Biol 2016;95:368-77. [Crossref] [PubMed]
- Wei B, Liu Y, Yue X, et al. Biological characteristics of tracheal smooth muscle cells regulated by NK-1R in asthmatic rat with airway remodeling. Int J Clin Exp Med 2015;8:14718-26. [PubMed]
- Gerthoffer WT. Migration of airway smooth muscle cells. Proc Am Thorac Soc 2008;5:97-105. [Crossref] [PubMed]
- Al-Alwan LA, Chang Y, Mogas A, et al. Differential roles of CXCL2 and CXCL3 and their receptors in regulating normal and asthmatic airway smooth muscle cell migration. J Immunol 2013;191:2731-41. [Crossref] [PubMed]
- Tang ML, Wilson JW, Stewart AG, et al. Airway remodelling in asthma: current understanding and implications for future therapies. Pharmacol Ther 2006;112:474-88. [Crossref] [PubMed]
- Delmotte P, Zavaletta VA, Thompson MA, et al. TNFα decreases mitochondrial movement in human airway smooth muscle. Am J Physiol Lung Cell Mol Physiol 2017;313:L166-76. [Crossref] [PubMed]
- Tan WJ, Tan QY, Wang T, et al. Calpain 1 regulates TGF-β1-induced epithelial-mesenchymal transition in human lung epithelial cells via PI3K/Akt signaling pathway. Am J Transl Res 2017;9:1402-9. [PubMed]
- Addi C, Bai J, Echard A. Actin, microtubule, septin and ESCRT filament remodeling during late steps of cytokinesis. Curr Opin Cell Biol 2018;50:27-34. [Crossref] [PubMed]
- Szymanski D, Staiger CJ. The Actin Cytoskeleton: Functional Arrays for Cytoplasmic Organization and Cell Shape Control. Plant Physiol 2018;176:106-18. [Crossref] [PubMed]
- Drechsler M, Giavazzi F, Cerbino R, et al. Active diffusion and advection in Drosophila oocytes result from the interplay of actin and microtubules. Nat Commun 2017;8:1520. [Crossref] [PubMed]
- Muñoz-Castañeda R, Díaz D, Peris L, et al. Cytoskeleton stability is essential for the integrity of the cerebellum and its motor- and affective-related behaviors. Sci Rep 2018;8:3072. [Crossref] [PubMed]
- Vemu A, Atherton J, Spector JO, et al. Tubulin isoform composition tunes microtubule dynamics. Mol Biol Cell 2017;28:3564-72. [Crossref] [PubMed]
- Piedra FA, Kim T, Garza ES, et al. GDP-to-GTP exchange on the microtubule end can contribute to the frequency of catastrophe. Mol Biol Cell 2016;27:3515-25. [Crossref] [PubMed]
- Mukherjee R, Majumder P, Chakrabarti O. MGRN1-mediated ubiquitination of α-tubulin regulates microtubule dynamics and intracellular transport. Traffic 2017;18:791-807. [Crossref] [PubMed]
- Belvindrah R, Natarajan K, Shabajee P, et al. Mutation of the α-tubulin Tuba1a leads to straighter microtubules and perturbs neuronal migration. J Cell Biol 2017;216:2443-61. [Crossref] [PubMed]
- Popova JS, Garrison JC, Rhee SG, et al. Tubulin, Gq, and phosphatidylinositol 4,5-bisphosphate interact to regulate phospholipase Cbeta1 signaling . J Biol Chem 1997;272:6760-5. [Crossref] [PubMed]
- Wei B, Shang YX, Li M, et al. Effect of Montelukast on the Expression of neurokinin-1 Receptor in Young Asthmatic Rats With Airway Remodeling. Zhongguo Dang Dai Er Ke Za Zhi 2013;15:298-301. [PubMed]