Fluorescent image-based evaluation of gastric conduit perfusion in a preclinical ischemia model
Introduction
Esophageal reconstruction after esophagectomy is associated with a high rate of complications and mortality (1,2). Anastomotic leaks are among the most significant complications following surgery, and their incidence is reportedly between 6.2% and 27% (3,4). The anastomosis area often experiences poor perfusion because the gastroepiploic arterial supply rarely reaches the end of the gastric conduit; this may lead to various complications such as tissue necrosis, edema, and subsequent anastomotic leakage (5-9). Therefore, the perfusion status of the gastric conduit is essential for the success of the procedure and intraoperative real-time evaluation of it would be the best way to improve the success rate of the surgery (6,10,11).
Several new technologies have been currently used to evaluate the perfusion of the gastric conduit. These include single-photon emission computed tomography (SPECT), computed tomography (CT) angiography, and visible light spectroscopy (12). However, SPECT and CT angiography cannot be performed intraoperatively, and visible light spectroscopy has a limited viewing area (13). Alternatively, laser doppler flowmetry has been applied because of its ease of use, efficiency, and reproducibility (6,14). However, it is limited to the microvasculature, and the size of the region of interest (ROI) is small, similar to that of visible light microscopy (15). Transmucosal oxygen saturation also might be used to assess perfusion with tissue fluorescein studies following postoperative monitoring; however, this also has a small ROI (16).
In recent years, near-infrared (NIR) fluorescence imaging has been demonstrated to offer exquisite sensitivity, enabling real-time detection of sentinel lymph nodes (SLN) (17). Since the first study of indocyanine green (ICG) NIR fluorescent imaging for evaluation of gastric conduit perfusion was conducted in 2011 (15), surgeons have tried to bring this technology into their operations (10,13,15,17-26). However, there were still lacks of data on the real-time observation performance of NIR fluorescence imaging technology on gastric conduit perfusion during esophageal surgery especially based on research using animal model.
In our previous studies, we developed an intraoperative color and fluorescence imaging system (ICFIS) that can visualize merged NIR fluorescence and white-light images in real time. It was shown that ICFIS visualized the NIR fluorescence from SLN in the lung and esophagus using various ICG based fluorescent tracers (27-29). The ICFIS also identified specific segments during pulmonary segmentectomy in rabbit and porcine models (30). Despite the excellent performance of the ICFIS, it has never been evaluated for immediate visualization of perfusion at an anastomotic area.
To more accurately evaluate the NIR fluorescence imaging system for detection of gastric conduit perfusion, an ischemic animal model of gastric conduit of which the overall perfusion should be verifiable in real time was required. Various animal models have been used for assessing the changes that supervene to the gastric remnant and subsequent esophagogastric anastomosis after partial gastric devascularization by ligation of the short gastric (SG) vessels or the left and short gastric (LG/SG) vessels (31-33). These ischemic conditioning models evaluate whether preoperative redistribution of the gastric blood supply can ameliorate lack of tissue oxygenation at the site of the esophagogastric anastomosis. However, an animal model for real-time feasibility assessment of gastric conduit perfusion using NIR fluorescence imaging system has not been established.
In this study, we created a large-animal model of gastric conduit ischemia through ligation and release of the right gastroepiploic artery (RGEA), which is the main source of blood to the reconstructed stomach. The ligation and release of the RGEA were expected to show a clear demarcation of the perfused and non-perfused areas of the gastric conduit in real time; this is a good way to objectively evaluate the superiority of the NIR fluorescent imaging system. We also compared the time to NIR fluorescent signal stabilization using peripheral and central line injections of ICG to determine whether the ICFIS could distinguish slight differences according to the injection method. Our study is expected to provide criteria for evaluation of the applicability of the fluorescent imaging technology in esophagectomy.
Methods
ICFIS
Our custom-manufactured ICFIS was previously described in detail (27-30). For the current study, the optics were modified to accommodate the entire surgical field near the gastric conduit (Figure 1).
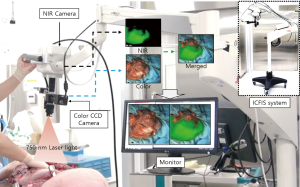
The excitation light source was a 793-nm laser diode with 1.0-W optical power. The beam was delivered by a liquid light guide (LLG0538-8, Thorlabs, Newton, NJ, USA) and expanded by a concave lens (LC1254-B, Thorlabs) that was attached next to the imaging optics. The optical power of the excitation beam was large enough to illuminate the entire surgical area uniformly, but was not above 0.5 mW/cm2, following the American National Standards Institute recommendation for fluence rate on tissues at a working distance of 350 mm. Both the fluorescence and anatomical color images were delivered through the same imaging optics until they were split by a dichroic mirror (750 DCXXR, Chroma, Bellows Falls, VT, USA). Images were recorded at 25 frames/s by an NIR electron-multiplying charge-coupled device (EMCCD) camera (Evolve 512, Photometrics, AZ, USA) and a color CCD camera (IMC-4018FT, IMI-tech, Anyang, Korea), respectively. All optical parts in the imaging path were anti reflective-coated over a wide visible-NIR range. Representative color, NIR fluorescence, and merged images are shown in Figure 2.
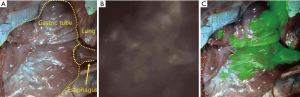
The ICFIS can visualize blood perfusion in tissues and blood flow within feeding vessels. Collected images were enhanced, aligned, merged using imaging software (Streampix6, Norpix, Canada), and displayed on the surgical monitor. Adjustments of NIR image threshold, contrast, and opacity were made using a custom-designed graphical user interface. The entire experiment was performed under bright surgical astral lamps, which caused no disturbance to the surgical view. The system was mounted on a surgical articulating arm that the practitioner could easily maneuver to a specific surgical ROI. We selected 3 ROI sites on each porcine esophagus and stomach and measured the pre-injection background and post-injection signal at each site.
Animals and animal care
The animal study procedures (including care and handling) were approved by the Institutional Animal Care and Use Committee of Korea University. Ten female Yorkshire pigs (Optipharm, Seoul, Korea) with a mean weight of 33.1±2.75 kg were used in this study. To help the pigs adapt to their environment, they were housed in individual cages with freely available food and water for 1 week, in accordance with our humane animal care protocol.
Real-time assessment of perfusion in a large animal model with gastric conduit ischemia using ICFIS
The pigs were injected with 15 mg/kg of Zoletil 50 (Virbac, Carros, France) and Rumpun (Bayer, Seoul, Korea). A mechanical ventilator was connected to the tracheostomy site and maintained with 2% isoflurane. Vital signs were monitored throughout surgery by a preclinical anesthetist. We performed a left thoracoabdominal incision to expose the middle to lower esophagus and stomach. Once the isolated lower esophagus was resected, a gastric conduit was prepared, and the LG/SG arteries were tied off, with only the RGEA and branches of the left GEA providing blood supply. Esophageal reconstruction was performed by advancing the greater curvature gastric conduit to the thorax for an esophagogastric anastomosis. The RGEA was ligated just before ICG (0.6 mg/kg; Sankyo, Seoul, Korea) injection, and it was released 2 minutes after ICG injection. We monitored the ICG fluorescence signals in the esophagus and gastric conduit during the entire procedure of ligation and releasing of the RGEA using the ICFIS (Figure S1). The ICG fluorescence signals in the esophagus and gastric conduit were measured at 30, 60, 90, 120, 300, and 360 s after ICG injection and were quantified by the signal-to-background ratio (SBR). We quantitatively evaluated the perfusion of the gastric conduits by comparing their SBR with that of the esophagus = gastric conduit SBR/esophageal SBR.
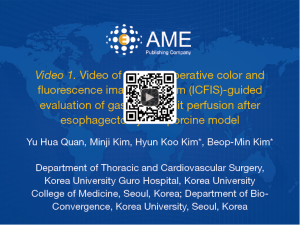
Comparison of gastric conduit perfusion between peripheral and central venous injection of ICG under ICFIS
To confirm whether there were differences in fluorescence signals obtained by two different injection routes, 0.6 mg/kg of ICG was injected into the ear vein (peripheral, n=6) or inferior vena cava (central, n=4) of the gastric conduit ischemic pig model. The blood supply with ICG fluorescence in the esophagus and gastric conduit was monitored in real time. We set the release of the ligation of RGEA as the starting point. We recorded the time point for the first observation of the ICG fluorescence signal in the esophagus and the time point of the ICG fluorescence being stably observed in the esophagus. After completion of the experiments, pigs were euthanized by direct injection of 20 mL air into the heart under anesthesia.
Statistical analysis
Signaling with central and peripheral vein injection was analyzed with a paired Mann-Whitney U test. ImageJ software was used for imaging analysis. SPSS Statistics for Windows Version 17.0 (SPSS Inc., Chicago, IL, USA) was used for statistical analysis. The null hypotheses of no difference were rejected if the P values were <0.05.
Results
The ICFIS can visualize blood perfusion in the esophagus and gastric conduit as well as blood flow within feeding vessels. The ICFIS also successfully visualized the ischemic region via NIR fluorescence detection. The surgical region was illuminated by the NIR excitation light source and bright surgical astral lamps (Figure 1). The NIR fluorescence from ICG was detected by a high-sensitivity NIR camera, and an enhanced NIR fluorescence image was merged with a color image, resulting in a real-time anatomical view. Consequently, NIR fluorescence appeared only on the esophagus and the parts of the gastric conduit with good blood perfusion. Each time point along with the fluorescent signal intensity of the fluorescence images were recorded throughout the procedure. Representative color, NIR fluorescence, and merged images as seen by the surgeon are shown in Figure 2.
Real-time NIR fluorescence diagnosis of the ischemic region and perfusion recovery
The ICG with a 0.6 mg/kg dose was intravenously administered in this experiment; this was regarded as optimal in our previous study (30). The NIR fluorescence signals from the gastric conduit and esophagus were detected every 30 s after ICG injection. With ligation of the REGA, SBR in the esophagus was significantly higher than in the gastric conduit (2.8±0.54 vs. 1.7±0.37, P=0.02, Figure 3). After releasing the ligation of the REGA, the NIR fluorescence signal in the gastric conduit rapidly increased; the ratio of gastric to esophageal SBR was 0.72±0.072 at 120 s and 0.97±0.024 at 300 s. In 8 of 10 pigs, the gastric conduit perfusion was completely recovered by releasing the RGEA ligation at 360 s (Figure 3).
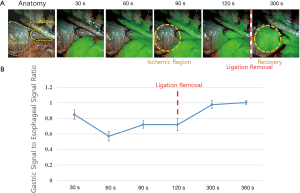
Time for noticeable fluorescence and stable fluorescence
To assess the ICFIS sensitivity for diagnosing ischemia, we chose central and peripheral veins as two different ICG injection routes and compared the resulting esophageal NIR signals using the ICFIS. The in vivo fluorescence signals in the esophagus were monitored, and noticeable fluorescence and stable fluorescence times were measured. The NIR fluorescence signal was first detected at 68.0±42.9 s with the central vein injection and at 138.0±82.1 s with the peripheral vein injection (P=0.157). The time to a stable NIR fluorescence signal, at which the signal was stable for at least 60 s, was 119.0 ± 65.1 s for the central route vs. 295.0±130.4 s for the peripheral route (P=0.04, Figure 4).
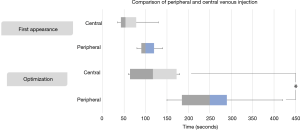
Possible clinical applications
Interestingly, in 2 pigs the region of the gastric conduit did not recover after releasing the ligation of the RGEA until after 360 s. As shown in Figure 5, the region with a low fluorescence signal in the fundus area of the gastric conduit could be diagnosed as the ischemic region intraoperatively. In these cases, we could make the decision to selectively resect the proximal ischemic region for eradication of the risk of gastric conduit ischemia and subsequent anastomotic complications.
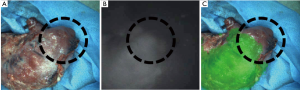
Discussion
In this study, we used an ischemic animal model that concretely evaluated the feasibility of the ICFIS for diagnosis of gastric conduit ischemia during reconstruction after esophagectomy. The ICFIS successfully provided a real-time NIR fluorescent image of esophageal and gastric conduit perfusion at an anastomotic area (Figures 1,2 and S1).
In our animal model, we performed both SG and LG artery ligation to mimic the actual surgical procedure in an esophageal patient (34-37). The RGEA, a dominant artery supplying blood to the gastric conduit, was intentionally ligated to induce gastric conduit ischemia. When ICG was administered, the NIR fluorescent signal mainly appeared on the esophagus and not on the gastric conduit side (Figure 3). At that point in time, the fluorescent SBR of the gastric conduit was lower than that of the esophagus. We additionally verified that gastric conduit perfusion recovered within 180 s after release of the ligation of the RGEA in the pig model. Although this ischemic model differs from a clinical scenario, in which the RGEA should not be ligated during esophageal reconstruction, we successfully developed the reversible gastric conduit ischemia model using ligation and release of the RGEA for evaluating the performance of ICFIS in ischemic area detection. We thought that the performance of the NIR fluorescent imaging system should be confirmed for accurate evaluation of gastric conduit perfusion in real time at the specific animal models.
We also evaluated whether the ICFIS could distinguish a slight difference based on the injection route of ICG by comparing the NIR fluorescent signal stabilization time. Although there was no statistical difference in fluorescence signal intensity, the central route diagnosed the ischemic region more rapidly than the peripheral route (Figure 4). Because the majority of patients undergoing esophagectomy will have their central line secured, use of central injection will allow the diagnosis of ischemia 180 s faster than with peripheral injection.
As shown in Figure 5, in 20% of cases (2 of 10 pigs) the ischemic area in the gastric conduit could be detected by the ICFIS after release of the ligation of the RGEA. Zehetner et al. reported that delayed or non-perfused areas of the gastric conduit were detected in 66% of cases in a clinical study (38). Therefore, in each clinical case, if ischemic regions could be detected using NIR fluorescent imaging during anastomosis, complications induced by non-perfusion were able to be prevented.
The ICFIS can be a competitive solution because it allows visualization over a wide ROI and provides intuitive perfusion information. Recently the SPY system, which can provide real-time intraoperative assessment of both gross and microperfusion, was used to assess gastric conduit perfusion in 150 patients undergoing esophagectomy (38). In our study, we used an ischemic animal model in an evaluation of the sensitivity and utility of ICFIS for real-time diagnosis of ischemic areas of the gastric conduit. Since the ICFIS is similar to the SPY system, its use may lead to altered surgical plans during esophagectomy using laser-assisted fluorescent-dye ICG angiography. However, a prospective study is needed to determine the incidence of anastomotic complications in which two groups of pigs are compared, groups with and without resection of an identified ischemic gastric region.
Conclusions
Using the ICFIS, we have shown that a simple ICG injection can help surgeons easily identify ischemic areas induced by ligation of the RGEA in large animals. Therefore, surgeons using the ICFIS can evaluate blood perfusion and perform a properly modified surgical procedure during an esophagectomy. Thus, the ICFIS-guided reconstruction after esophagectomy may be an optimal choice for surgery in patients with esophageal cancer.
Acknowledgements
Funding: This work was supported by a Korea University Grant and a grant from the Korean Health Technology R&D Project, Ministry of Health & Welfare, Republic of Korea (No. HI17C0654 and HI13C1501).
Footnote
Conflicts of Interest: The authors have no conflicts of interest to declare.
Ethical Statement: This study was approved by Institutional Animal Care and Use Committee of Korea University (No. KOREA-2017-0030).
References
- Bailey SH, Bull DA, Harpole DH, et al. Outcomes after esophagectomy: a ten-year prospective cohort. Ann Thorac Surg 2003;75:217-22; discussion 222. [Crossref] [PubMed]
- Ferguson MK, Martin TR, Reeder LB, et al. Mortality after esophagectomy: risk factor analysis. World J Surg 1997;21:599-603; discussion 603-4. [Crossref] [PubMed]
- Sarela AI, Tolan DJ, Harris K, et al. Anastomotic leakage after esophagectomy for cancer: a mortality-free experience. J Am Coll Surg 2008;206:516-23. [Crossref] [PubMed]
- Udagawa H, Akiyama H. Surgical treatment of esophageal cancer: Tokyo experience of the three-field technique. Dis Esophagus 2001;14:110-4. [Crossref] [PubMed]
- Urschel JD. Esophagogastrostomy anastomotic leaks complicating esophagectomy: a review. Am J Surg 1995;169:634-40. [Crossref] [PubMed]
- Boyle NH, Pearce A, Hunter D, et al. Intraoperative scanning laser Doppler flowmetry in the assessment of gastric tube perfusion during esophageal resection. J Am Coll Surg 1999;188:498-502. [Crossref] [PubMed]
- Dewar L, Gelfand G, Finley RJ, et al. Factors affecting cervical anastomotic leak and stricture formation following esophagogastrectomy and gastric tube interposition. Am J Surg 1992;163:484-9. [Crossref] [PubMed]
- Liebermann-Meffert DM, Meier R, Siewert JR. Vascular anatomy of the gastric tube used for esophageal reconstruction. Ann Thorac Surg 1992;54:1110-5. [Crossref] [PubMed]
- Meyerson SL, Mehta CK. Managing complications II: conduit failure and conduit airway fistulas. J Thorac Dis 2014;6 Suppl 3:S364-71. [PubMed]
- Karliczek A, Benaron DA, Baas PC, et al. Intraoperative assessment of microperfusion with visible light spectroscopy for prediction of anastomotic leakage in colorectal anastomoses. Colorectal Dis 2010;12:1018-25. [Crossref] [PubMed]
- Karliczek A, Harlaar NJ, Zeebregts CJ, et al. Surgeons lack predictive accuracy for anastomotic leakage in gastrointestinal surgery. Int J Colorectal Dis 2009;24:569-76. [Crossref] [PubMed]
- Hochwald SN, Ben-David K. Minimally invasive esophagectomy with cervical esophagogastric anastomosis. J Gastrointest Surg 2012;16:1775-81. [Crossref] [PubMed]
- Pacheco PE, Hill SM, Henriques SM, et al. The novel use of intraoperative laser-induced fluorescence of indocyanine green tissue angiography for evaluation of the gastric conduit in esophageal reconstructive surgery. Am J Surg 2013;205:349-52; discussion 352-3. [Crossref] [PubMed]
- Pierie JP, De Graaf PW, Poen H, et al. Impaired healing of cervical oesophagogastrostomies can be predicted by estimation of gastric serosal blood perfusion by laser Doppler flowmetry. Eur J Surg 1994;160:599-603. [PubMed]
- Shimada Y, Okumura T, Nagata T, et al. Usefulness of blood supply visualization by indocyanine green fluorescence for reconstruction during esophagectomy. Esophagus 2011;8:259-66. [Crossref] [PubMed]
- Jacobi CA, Zieren HU, Zieren J, et al. Is tissue oxygen tension during esophagectomy a predictor of esophagogastric anastomotic healing? J Surg Res 1998;74:161-4. [Crossref] [PubMed]
- Murawa D, Hunerbein M, Spychala A, et al. Indocyanine green angiography for evaluation of gastric conduit perfusion during esophagectomy--first experience. Acta Chir Belg 2012;112:275-80. [PubMed]
- Rino Y, Yukawa N, Sato T, et al. Visualization of blood supply route to the reconstructed stomach by indocyanine green fluorescence imaging during esophagectomy. BMC Med Imaging 2014;14:18. [Crossref] [PubMed]
- Ishiguro T, Kumagai Y, Ono T, et al. Usefulness of indocyanine green angiography for evaluation of blood supply in a reconstructed gastric tube during esophagectomy. Int Surg 2012;97:340-4. [Crossref] [PubMed]
- Sarkaria IS, Bains MS, Finley DJ, et al. Intraoperative near-infrared fluorescence imaging as an adjunct to robotic-assisted minimally invasive esophagectomy. Innovations (Phila) 2014;9:391-3. [Crossref] [PubMed]
- Yukaya T, Saeki H, Kasagi Y, et al. Indocyanine Green Fluorescence Angiography for Quantitative Evaluation of Gastric Tube Perfusion in Patients Undergoing Esophagectomy. J Am Coll Surg 2015;221:e37-42. [Crossref] [PubMed]
- Nakano T, Sakurai T, Maruyama S, et al. Indocyanine green fluorescence and three-dimensional imaging of right gastroepiploic artery in gastric tube cancer. World J Gastroenterol 2015;21:369-72. [Crossref] [PubMed]
- Kumagai Y, Ishiguro T, Haga N, et al. Hemodynamics of the reconstructed gastric tube during esophagectomy: assessment of outcomes with indocyanine green fluorescence. World J Surg 2014;38:138-43. [Crossref] [PubMed]
- Koyanagi K, Ozawa S, Oguma J, et al. Blood flow speed of the gastric conduit assessed by indocyanine green fluorescence: New predictive evaluation of anastomotic leakage after esophagectomy. Medicine (Baltimore) 2016;95. [Crossref] [PubMed]
- Nakashima Y, Saeki H, Yukaya T, et al. Blood Flow Assessment with Indocyanine Green Fluorescence Angiography for Pedicled Omental Flap on Cervical Esophagogastric Anastomosis after Esophagectomy. J Am Coll Surg 2016;222:e67-9. [Crossref] [PubMed]
- Kubota K, Yoshida M, Kuroda J, et al. Application of the HyperEye Medical System for esophageal cancer surgery: a preliminary report. Surg Today 2013;43:215-20. [Crossref] [PubMed]
- Kim HK, Quan YH, Oh Y, et al. Macrophage-Targeted Indocyanine Green-Neomannosyl Human Serum Albumin for Intraoperative Sentinel Lymph Node Mapping in Porcine Esophagus. Ann Thorac Surg 2016;102:1149-55. [Crossref] [PubMed]
- Oh Y, Lee YS, Quan YH, et al. Thoracoscopic color and fluorescence imaging system for sentinel lymph node mapping in porcine lung using indocyanine green-neomannosyl human serum albumin: intraoperative image-guided sentinel nodes navigation. Ann Surg Oncol 2014;21:1182-8. [Crossref] [PubMed]
- Oh Y, Quan YH, Choi Y, et al. Intraoperative combined color and fluorescent images-based sentinel node mapping in the porcine lung: comparison of indocyanine green with or without albumin premixing. J Thorac Cardiovasc Surg 2013;146:1509-15. [Crossref] [PubMed]
- Oh Y, Quan YH, Kim M, et al. Intraoperative fluorescence image-guided pulmonary segmentectomy. J Surg Res 2015;199:287-93. [Crossref] [PubMed]
- Kechagias A, van Rossum PS, Ruurda JP, et al. Ischemic Conditioning of the Stomach in the Prevention of Esophagogastric Anastomotic Leakage After Esophagectomy. Ann Thorac Surg 2016;101:1614-23. [Crossref] [PubMed]
- Beck SM, Malay MB, Gagne DJ, et al. Experimental model of laparoscopic gastric ischemic preconditioning prior to transhiatal esophagectomy. Surg Endosc 2011;25:2470-7. [Crossref] [PubMed]
- Reavis KM, Chang EY, Hunter JG, et al. Utilization of the delay phenomenon improves blood flow and reduces collagen deposition in esophagogastric anastomoses. Ann Surg 2005;241:736-45; discussion 745-7. [Crossref] [PubMed]
- Chasseray VM, Kiroff GK, Buard JL, et al. Cervical or thoracic anastomosis for esophagectomy for carcinoma. Surg Gynecol Obstet 1989;169:55-62. [PubMed]
- Muller JM, Erasmi H, Stelzner M, et al. Surgical therapy of oesophageal carcinoma. Br J Surg 1990;77:845-857. [Crossref] [PubMed]
- Ando N, Ozawa S, Kitagawa Y, et al. Improvement in the results of surgical treatment of advanced squamous esophageal carcinoma during 15 consecutive years. Ann Surg 2000;232:225-32. [Crossref] [PubMed]
- Wormuth JK, Heitmiller RF. Esophageal conduit necrosis. Thorac Surg Clin 2006;16:11-22. [Crossref] [PubMed]
- Zehetner J, DeMeester SR, Alicuben ET, et al. Intraoperative Assessment of Perfusion of the Gastric Graft and Correlation With Anastomotic Leaks After Esophagectomy. Ann Surg 2015;262:74-8. [Crossref] [PubMed]
- Quan YH, Kim M, Kim HK, et al. Video of the intraoperative color and fluorescence imaging system (ICFIS)-guided evaluation of gastric conduit perfusion after esophagectomy in a porcine model. Asvide 2018;5:782. Available online: http://www.asvide.com/article/view/27400