Obstructive sleep apnea in patients with chronic thromboembolic pulmonary hypertension
Introduction
Sleep apnea syndrome (SAS), a highly prevalent public health problem, is characterized by a recurrent apnea and hypopnea during sleep. Obstructive sleep apnea (OSA) is the most frequent subtype of SAS, which is caused by a complete or partial obstruction of the upper airway during nocturnal sleep (1). Except for leading to a decline in sleep quality decline and daytime sleepiness, OSA is considered to be a risk factor for many cardiovascular diseases like, coronary artery disease, hypertension, heart failure, arrhythmia and pulmonary hypertension (PH). The underlying mechanism that causes this may be relevant to an exaggerated negative intrathoracic pressure, sympathetic activation, oxidative stress, systemic inflammation and endothelial dysfunction (2).
OSA most often occurs in men, the obese, and those who are aged more than 65 years (3). Recently, several studies have revealed that OSA is closely linked to a thromboembolic phenomenon, due to the increased platelet activation and hypercoagulability (4). Deflandre et al. (5) found that OSA was an independent risk factor for venous thromboembolism (VTE) events. Arzt et al. (6) also demonstrated that OSA occurred more frequently in patients with deep vein thrombosis (DVT) and/or acute pulmonary embolism (PE) than in the control group. Chronic thromboembolic pulmonary hypertension (CTEPH) is characterized by a chronic obstruction of the major pulmonary arteries and microvascular disease, and is regarded as a long-term complication of PE event. CTEPH is classified as a group 4 PH by the World Health Organization (WHO). It has been reported that the incidence of CTEPH within the first 2 years after suffering from a PE event is about 0.1–9.1% (7,8). Furthermore, specific risk factors for VTE have been identified as risk factors for CTEPH (9). This is in line with the high prevalence of OSA in CTEPH patients. Dumitrascu et al. (10) found that approximately 20% of the CTEPH patients were accompanied by OSA. However, the data about the relationship between CTEPH and OSA is still lacking. To investigate whether OSA has an effect on CTEPH patients, we performed a single-center, retrospective study in CTEPH patients whose diagnosis had been established by a right heart catheterization (RHC). In addition, factors associated with OSA in CTEPH were also investigated.
Methods
This single center study was conducted in Fuwai Hospital, National Center for Cardiovascular Diseases in Beijing, China. The study was performed with the approval of the Fuwai Hospital Ethics Committee. Written informed consent of all participants was obtained.
Study sample
We retrospectively reviewed the data of the hospitalized patients who had been newly diagnosed with CTEPH between August 2015 and December 2017. Before diagnosis, each patient received at least three months of effective anticoagulation therapy and underwent routine screening of CTEPH which included echocardiography, ventilation/perfusion scan and computed tomography (CT) angiography. Finally, patients whose RHC results showed a mean pulmonary arterial pressure (mPAP) of ≥25 mmHg with a pulmonary artery wedge pressure (PAWP) of ≤15 mmHg, together with mismatched perfusion defects by image inspecting, were confirmed with CTEPH (11). Hospitalized patients in our center who had snoring at night, daytime sleepiness, neck circumference enlargement or micrognathia were advised to receive overnight polysomnography (PSG). Patients who agreed to receive the PSG, and had finally been diagnosed with CTEPH were enrolled. All enrolled CTEPH patients were in stable clinical status. PSG was performed before or after RHC in hospital; the time interval was no more than 7 days. According to the results of the PSG, all participants were then divided into two patient categories: CTEPH patients with and without OSA. Patients having one of the following conditions were excluded: (I) other forms of PH; (II) age less than eighteen years old; (III) chronic heart failure with ejection fraction <30%; (IV) chronic liver or kidney dysfunction, defined as having three times higher liver enzymes than normal and a creatinine clearance rate of less than 30 milliliter/minute respectively; (V) life-threatening cardiac arrhythmias; (VI) a sleep period of less than two hours.
Patients assessment
The baseline clinical characteristics of each participant was collected, including the age, sex, body mass index (BMI), smoking history, six-minute walking distance (6-MWD), WHO functional class (WHO FC), comorbidity, drug therapy and PE, or VTE history. In addition to this, hemoglobin (Hb), hematocrit (Ht), mean corpuscular volume (MCV), red blood cell width (RDW), N-terminal pro-brain natriuretic peptide (NT-proBNP), uric acid, creatinine and high sensitive C reactive protein (hs-CRP) were measured using fasting venous blood samples on the first day of admission. Partial pressure of oxygen (PaO2), partial pressure of carbon dioxide (PaCO2) and oxygen saturation (SPO2) were measured by arterial blood gas. Transthoracic echocardiography and pulmonary function testing were performed on each patient before RHC. Diastolic left ventricle diameter was measured in the left ventricular long axis view and diastolic right ventricle diameter was measured in the apical four chamber view. SPAP is equal to right ventricular systolic pressure (RVSP) in the absence of a gradient of across the pulmonic valve or right ventricular outflow tract. RVSP can be reliably estimated from a peak tricuspid regurgitation jet velocity, using the simplified Bernoulli equation, combined with the right atrium pressure (RAP) level: RVSP=4 (peak tricuspid regurgitation jet velocity)2 + RAP (12). In addition, the Simpson biplane method was used to assess EF. To confirm the diagnosis of CTEPH, RHC was conducted. The baseline hemodynamic parameters, i.e., mean RAP (mRAP), mPAP, cardiac index (CI) and pulmonary vascular resistance (PVR) were obtained in all patients.
Sleep study
Each enrolled CTEPH patients undertook an overnight PSG test in the Sleep Center of Fuwai Hospital using the Embletta (Medcare Flaga, Reykjavik, Iceland). The device recorded nasal airflow, finger pulse oximetry, thoracic and abdominal movement, body position, and snoring. Based on the American Academy of Sleep Medicine (AASM) manual for the scoring of sleep and associated events (13), the definition of a sleep apnea event must satisfy a decrease of nasal airflow by more than 90%, lasting at least 10 seconds. If the airflow absence is accompanied by a persistent or enhanced inspiratory effort, the apnea event is considered as obstructive. The definition of a hypopnea event must meet a decrease of nasal airflow by more than 30%, lasting at least 10 seconds along with a drop of more than 4% oxygen desaturation. If there is snoring or increased inspiratory flattening of the nasal pressure or associated thoracoabdominal paradox, the hypopnea event is considered as obstructive. The total number of apnea and hypopnea events is divided by the number of sleeping hours to calculate the apnea-hypopnea index (AHI). AHI ≥5/h and the obstructive events ≥50% are diagnostic criteria of SAS.
Statistical analysis
Continuous variables are presented as a mean ± SD or median (interquartile range). Categorical variables are given as counts or percentages. In order to compare the baseline parameters between two groups, an unpaired t-test was used for normally distributed, continuous variables and a nonparametric Kruskal-Wallis test was used for the non-normally distributed continuous variables. Categorical variables were compared using an χ2 test. To explore possible factors that were associated with OSA in CTEPH, logistic regression analysis was also used. P value <0.05 was considered as statistically significant. Data analysis was performed using SPSS version 22.0 (SPSS Inc., Chicago, IL, USA).
Results
Patients characteristics
A total of 78 patients were newly confirmed with CTEPH and in a stable clinical status. Among them, 62 patients received an overnight PSG monitoring before or after RHC. Five CTEPH patients without available sleep data were excluded. Finally, 57 CTEPH patients who received PSG were enrolled. Baseline clinical characteristics of CTEPH patients with and without OSA were compared, and are displayed in Table 1. CTEPH patients with OSA showed an older age (57±9 versus 50±12 years, P=0.020), a higher BMI (24.98±3.06 versus 23.32±2.95 kg·m−2, P=0.044) and a worse WHO FC (P=0.033). Other characteristics did not make a difference between the two groups.
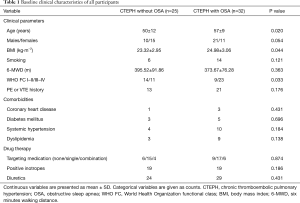
Full table
Laboratory, hemodynamic and echocardiographic tests
Table 2 compared laboratory, hemodynamic and echocardiographic parameters between the two groups. CTEPH patients with OSA have a higher hemoglobin level (163.47±15.81 versus 155.24±14.10 g/L, P=0.046) than those without. There was no difference in other parameters.
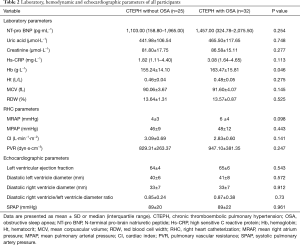
Full table
Pulmonary function, arterial blood gas and sleep study
Data about the pulmonary function, arterial blood gas and sleep study of the two groups are shown in Table 3. CTEPH patients with and without OSA had a similar pulmonary function. However, OSA patients had a lower PaO2 (60.13%±13.48% versus 68.22%±13.27%, P=0.008) and SPO2 (90.32%±4.16% versus 93.26%±2.25%, P=0.004). In terms of the sleep data, the OSA group had a higher AHI (16.74±12.21 versus 2.43±1.69, P<0.001) and oxygen desaturation index [(13.35 (8.40, 31.45) versus 3.50 (1.75, 6.05), P<0.001] but a lower minimum SPO2 (77.25%±7.82% versus 80.28%±8.75%, P=0.041).
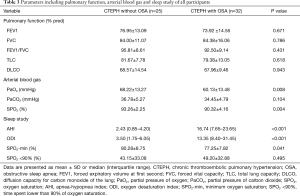
Full table
Possible factors associated with OSA in CTEPH
Logistic regression analysis which was adjusted for age, sex and BMI demonstrated that hemoglobin [odd ratio (OR) =1.057, 95% confidence interval (CI): 1.001–1.117, P=0.046], SPO2 (OR =0.718, 95% CI: 0.554–0.929, P=0.012), NT-proBNP (OR =1.001, 95% CI: 1.000–1.002, P=0.016) and WHO FC III–IV (OR =18.550, 95% CI: 2.363–144.128, P=0.005) were associated with OSA in CTEPH. In addition, hemodynamic parameters including mRAP (OR =1.284, 95% CI: 1.030–1.600, P=0.026), mPAP (OR =1.087, 95% CI: 1.001–1.180, P=0.048), CI (OR =0.058, 95% CI: 0.008–0.433, P=0.037), PVR (OR =1.004, 95% CI: 1.001–1.007, P=0.014) were also associated with OSA in CTEPH. These details are shown in Table 4. To further identify which factor was more important in indicating the coexistence of OSA and CTEPH, a multivariate logistic regression analysis including SPO2, NT-proBNP, WHO FC, mRAP, mPAP, CI in addition to age, sex and BMI was performed, which is detailed in Table 5.
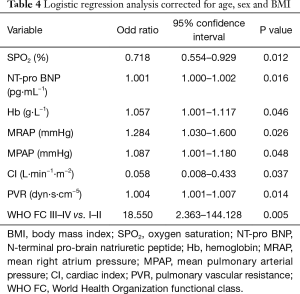
Full table
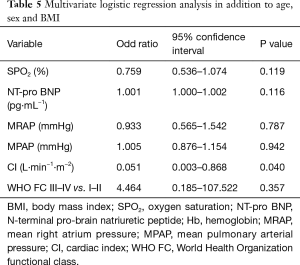
Full table
Discussion
Since the early 1970s, there has been an increasing amount of studies demonstrating how OSA can increase the mortality rate of various cardiovascular diseases (14). Intermittent hypoxia and an inflammation condition which had resulted from OSA may contribute to pulmonary vasoconstriction and pulmonary vascular remodeling, leading to a temporary or persistent PH (15). However, few studies have investigated the relationship between OSA and CTEPH. In this study, we demonstrated that the clinical status of CTEPH patients with OSA might be exacerbated when compared to those patients without OSA, which was supported by a higher Hb, lower SPO2 and worse WHO FC. Adjusted for age, sex and BMI, a worse hemodynamics, oxygenation state and cardiac function were associated with OSA in CTEPH. Multivariate regression analysis has further demonstrated CI was independently associated with OSA in CTEPH, among multiple other factors in addition to age, sex and BMI. However, we did not observe a linear relationship between CI and AHI as Orr et al. Established on a previous study that demonstrated the relationship between OSA and cardiovascular diseases (2), the influence of OSA on CTEPH was mainly discussed.
OSA itself can be a cause of PH which is categorized as WHO group 3 PH. Through hypoxic pulmonary vasoconstriction and left heart damage, OSA can lead to pre-capillary and post-capillary PH (11). Sajkov et al. (16) separately investigated the prevalence of PH in 27 and 32 OSA patients without cardiopulmonary diseases in 1994 and 1999. About 41% and 34% of these OSA patients were screened as PH, respectively using echocardiography. OSA often occurs in male, the obese and older individuals (17-19). This was proved by our results, which showed that CTEPH patients with OSA had an older age, a higher BMI and a larger proportion of male sex. In addition, OSA is prevalent in the population with cardiovascular diseases, particularly left heart failure. There is also a high prevalence of OSA in PH patients. Minic et al. (20) demonstrated that 56% of the 72 patients with WHO group 1 PH had OSA. In our study, we found that 32 of the 57 CTEPH patients had OSA. This high prevalence of OSA in CTEPH accorded with the findings of Orr et al. (21), whose study revealed that sleep disordered breathing was found in 57% of the 49 CTEPH patients.
Intermittent hypoxia and apnea bring about an enhanced inspiratory effort, as well as a large negative intrathoracic pressure swing. Increased venous return leads to a right ventricular enlargement, and thus impedes the left ventricular filling (16). Additionally, increasing amounts of studies have revealed that OSA possibly has negative effects on the right ventricular function and structure. Guidry et al. (22) revealed that the right ventricular wall measured by echocardiography was much thicker in severe OSA patients, when compared with mild OSA patients. Other studies also revealed a decrease of the right ventricular contraction in patients with severe OSA (23,24). The detrimental influences of OSA on the left and right ventricles were consistent with the worse WHO FC in CTEPH patients with OSA in our study. This theory was also confirmed by our multivariate logistic regression results, which demonstrated that CI was independently associated with OSA in CTEPH in addition to age, sex and BMI. However, there was not a linear relationship between CI and AHI (data not shown). We speculated that a lower CI was associated with the coexistence of OSA, rather than associated with the severity of OSA in CTEPH. The chronic effects of OSA on pulmonary circulation and right ventricle also lead to a deteriorated hemodynamic (25); however, due to the limited sample size, hemodynamic parameters in this study did not reach a statistical difference in CTEPH patients with and without OSA.
Nocturnal hypoxia stimulates pulmonary vasoconstriction, sympathetic activation, inflammation and oxidative stress which are secondary to OSA to promote pulmonary vascular remodeling (26). These pulmonary vascular changes induced by the OSA could aggravate the mismatched ventilation and perfusion of CTEPH patients, which was supported by a lower PaO2 and SPO2 level in arterial blood gas, higher oxygen desaturation index and minimum SPO2 during night sleep in CTEPH patients with OSA. This result was similar to the previous study by Bady et al. (27) which had revealed a lower daytime arterial oxygen tension and a more severe nocturnal hypoxemia in pulmonary arterial hypertension patients with OSA. Our study showed lower SPO2 was a factor associated with OSA in patients with CTEPH in Table 4. This finding was in accordance with the research by Zhong et al. (28) that revealed SPO2 in waking condition might play a role as a predictor for the evaluation and the diagnosis in the patients with OSA. Besides, SPO2 and AHI had a negative linear correlation in our study (data not shown). Moreover, higher Hb was observed in CTEPH patients with OSA in this study. Carlson et al. (29) and Moore-Gillon et al. (30) also observed a portion of patients with unexplained polycythemia who suffered from sleep breathing disorders. Two mechanisms may explain the elevation of Hb in OSA patients: (I) erythropoiesis induced by oxygen desaturation; (II) hemoconcentration resulting from fluid shifts from the intravascular to the extravascular bed. Hb elevation contributes to an increased blood viscosity of OSA patients, which can promote the occurrence of VTE. VTE including DVT and PTE appear to be more prevalent in OSA patients than in normal people, and OSA is even an independent risk factor for recurrent VTE (31). This phenomenon may be ascribed to the hypercoagulability and low fibrinolysis state of OSA. Bokinsky et al. (32) measured blood platelet aggregation and activation of patients with suspected OSA. The results showed an increased platelet aggregation and activation in OSA patients, whose effect was reduced by the therapy of OSA. Although there is no study suggesting OSA is a risk factor for CTEPH, we presume OSA can perhaps exacerbate the preexisting CTEPH, or even originally cause CTEPH in this way. Fluid retention in decompensated CTEPH patients facilitates a peripharyngeal edema during sleep, inducing a more severe narrowing of the upper airway, than found in the general population (33). Thus, the severity of CTEPH may be associated with OSA in turn. However, data in this study could not explain whether CTEPH aggravated OSA.
Clinical implications
From this study, we noticed CTEPH patients with OSA might have a worse clinical condition, than the CTEPH patients without OSA, reflecting the potentially detrimental influence of OSA on CTEPH in some way. We speculate OSA may further increase the mortality of patients with CTEPH who are receiving a long term follow up. Therefore, it is imperative to recognize the existence of OSA in CTEPH patients, and give appropriate therapy for it.
Study limitations
The first limitation of our study is small sample size, due to a relative low incidence of CTEPH. Selection bias is unavoidable in a retrospective study. As a pulmonary vascular disease center, it is more likely CTEPH patients with OSA combined will be admitted. Also, the above-mentioned inclusion criteria for PSG may lead to the high detection rate of OSA. Using bed time to calculate AHI, which may underdiagnose OSA, is another limitation. In fact, the actual sleeping time is less than bed time. Moreover, we did not follow up with these patients, so we cannot ensure the effect of OSA on the mortality of CTEPH patients and if OSA contributes to a persistent and recurrent PH after CTEPH patients undergoing pulmonary endarterectomy. Additionally, whether OSA treatment can alleviate CTEPH and whether CTEPH treatment can alleviate OSA is not investigated in our study. Larger, prospective cohort studies are needed to solve these problems.
Conclusions
In summary, OSA may aggravate CTEPH in some way. It may be of great significance to recognize and treat OSA in CTEPH patients. The clinical significance of the relationship between OSA and CTEPH warrants further studies.
Acknowledgements
We thank Chenhong An and Xiuping Ma for their assistance with the PSG monitoring.
Footnote
Conflicts of Interest: The authors have no conflicts of interest to declare.
Ethical Statement: The study was performed with the approval of Fuwai Hospital Ethics Committee (No. 2009215). Written informed consents of all participants were obtained.
References
- Huang Z, Liu Z, Luo Q, et al. Predictors of blood pressure fall with continuous positive airway pressure treatment in hypertension with coronary artery disease and obstructive sleep apnea. Can J Cardiol 2015;31:853-9. [Crossref] [PubMed]
- Kasai T, Floras JS, Bradley TD. Sleep apnea and cardiovascular disease: a bidirectional relationship. Circulation 2012;126:1495-510. [Crossref] [PubMed]
- Punjabi NM. The epidemiology of adult obstructive sleep apnea. Proc Am Thorac Soc 2008;5:136-43. [Crossref] [PubMed]
- Liak C, Fitzpatrick M. Coagulability in obstructive sleep apnea. Can Respir J 2011;18:338-48. [Crossref] [PubMed]
- Deflandre E, Degey S, Opsomer N, et al. Obstructive sleep apnea and smoking as a risk factor for venous thromboembolism events: review of the literature on the common pathophysiological mechanisms. Obes Surg 2016;26:640-8. [Crossref] [PubMed]
- Arzt M, Luigart R, Schum C, et al. Sleep-disordered breathing in deep vein thrombosis and acute pulmonary embolism. Eur Respir J 2012;40:919-24. [Crossref] [PubMed]
- Tapson VF, Humbert M. Incidence and prevalence of chronic thromboembolic pulmonary hypertension: from acute to chronic pulmonary embolism. Proc Am Thorac Soc 2006;3:564-7. [Crossref] [PubMed]
- Martí D, Gomez V, Escobar C, et al. Incidence of symptomatic and asymptomatic chronic thromboembolic pulmonary hypertension. Arch Bronconeumol 2010;46:628-33. [Crossref] [PubMed]
- Bonderman D, Jakowitsch J, Adlbrecht C, et al. Medical conditions increasing the risk of chronic thromboembolic pulmonary hypertension. Thromb Haemost 2005;93:512-6. [PubMed]
- Dumitrascu R, Tiede H, Eckermann J, et al. Sleep apnea in precapillary pulmonary hypertension. Sleep Med 2013;14:247-51. [Crossref] [PubMed]
- Galiè N, Humbert M, Vachiery J, et al. 2015 ESC/ERS Guidelines for the diagnosis and treatment of pulmonary hypertension. Eur Respir J 2015;46:903-975. [Crossref] [PubMed]
- Rudski LG, Lai WW, Afilalo J, et al. Guidelines for the echocardiographic assessment of the right heart in adults: a report from the American Society of Echocardiography endorsed by the European Association of Echocardiography, a registered branch of the European Society of Cardiology, and the Canadian Society of Echocardiography. J Am Soc Echocardiogr 2010;23:685-713. [Crossref] [PubMed]
- Berry RB, Budhiraja R, Gottlieb DJ, et al. Rules for scoring respiratory events in sleep: update of the 2007 AASM Manual for the Scoring of Sleep and Associated Events. Deliberations of the Sleep Apnea Definitions Task Force of the American Academy of Sleep Medicine. J Clin Sleep Med 2012;8:597-619. [PubMed]
- Peppard PE, Young T, Palta M, et al. Prospective study of the association between sleep-disordered breathing and hypertension. N Engl J Med 2000;342:1378-84. [Crossref] [PubMed]
- Dumitrascu R, Heitmann J, Seeger W, et al. Obstructive sleep apnea, oxidative stress and cardiovascular disease: lessons from animal studies. Oxid Med Cell Longev 2013;2013. [Crossref] [PubMed]
- Sajkov D, McEvoy RD. Obstructive sleep apnea and pulmonary hypertension. Prog Cardiovasc Dis 2009;51:363-70. [Crossref] [PubMed]
- Schwartz AR, Patil SP, Squier S, et al. Obesity and upper airway control during sleep. J Appl Physiol (1985) 2010;108:430-5. [Crossref] [PubMed]
- Edwards BA, O'Driscoll DM, Ali A, et al. Aging and sleep: physiology and pathophysiology. Semin Respir Crit Care Med 2010;31:618-33. [Crossref] [PubMed]
- Jordan AS, McSharry DG, Malhotra A. Adult obstructive sleep apnoea. Lancet 2014;383:736-47. [Crossref] [PubMed]
- Minic M, Granton JT, Ryan CM. Sleep disordered breathing in group 1 pulmonary arterial hypertension. J Clin Sleep Med 2014;10:277-83. [PubMed]
- Orr JE, Auger WR, DeYoung PN, et al. Usefulness of low cardiac index to predict sleep-disordered breathing in chronic thromboembolic pulmonary hypertension. Am J Cardiol 2016;117:1001-5. [Crossref] [PubMed]
- Guidry UC, Mendes LA, Evans JC, et al. Echocardiographic features of the right heart in sleep-disordered breathing: the Framingham Heart Study. Am J Respir Crit Care Med 2001;164:933-8. [Crossref] [PubMed]
- Romero-Corral A, Somers VK, Pellikka PA, et al. Decreased right and left ventricular myocardial performance in obstructive sleep apnea. Chest 2007;132:1863-70. [Crossref] [PubMed]
- Dursunoğlu N, Dursunoglu D, Kilic M. Impact of obstructive sleep apnea on right ventricular global function: sleep apnea and myocardial performance index. Respiration 2005;72:278-84. [Crossref] [PubMed]
- Chaouat A, Weitzenblum E, Krieger J, et al. Pulmonary hemodynamics in the obstructive sleep apnea syndrome. Results in 220 consecutive patients. Chest 1996;109:380-6. [Crossref] [PubMed]
- Fagan KA. Selected Contribution: Pulmonary hypertension in mice following intermittent hypoxia. J Appl Physiol (1985) 2001;90:2502-7. [PubMed]
- Bady E, Achkar A, Pascal S, et al. Pulmonary arterial hypertension in patients with sleep apnoea syndrome. Thorax 2000;55:934-9. [Crossref] [PubMed]
- Zhong ZQ, Tao Y, Zhang ZP, et al. Relationship between excessive daytime sleepiness and oxygen saturation in obstructive sleep apnea-hypopnea syndrome. Zhonghua Yi Xue Za Zhi 2011;91:40-3. [PubMed]
- Carlson JT, Hedner J, Fagerberg B, et al. Secondary polycythaemia associated with nocturnal apnoea--a relationship not mediated by erythropoietin? J Intern Med 1992;231:381-7. [Crossref] [PubMed]
- Moore-Gillon JC, Treacher DF, Gaminara EJ, et al. Intermittent hypoxia in patients with unexplained polycythaemia. Br Med J (Clin Res Ed) 1986;293:588-90. [Crossref] [PubMed]
- Alonso-Fernández A, Suquia AG, de la Pena M, et al. OSA is a risk factor for recurrent VTE. Chest 2016;150:1291-301. [Crossref] [PubMed]
- Bokinsky G, Miller M, Ault K, et al. Spontaneous platelet activation and aggregation during obstructive sleep apnea and its response to therapy with nasal continuous positive airway pressure. A preliminary investigation. Chest 1995;108:625-30. [Crossref] [PubMed]
- White LH, Bradley TD. Role of nocturnal rostral fluid shift in the pathogenesis of obstructive and central sleep apnoea. J Physiol 2013;591:1179-93. [Crossref] [PubMed]