Evaluating the response of neoadjuvant chemotherapy for treatment of breast cancer: are tumor biomarkers and dynamic contrast enhanced MR images useful predictive tools?
Introduction
In recent years, a number of clinical research results have indicated that neoadjuvant chemotherapy (NAC) has failed to increase overall survival (OS) in patients with early stage breast cancer (1). However, OS of patients with local advanced breast cancer has increased after NAC if they achieved complete pathological response (pCR) (2,3). It would be beneficial to clinical practice if an evaluation system for NAC therapeutic effect could be identified.
Compared with clinical examination, ultrasonography and mammography, dynamic contrast enhanced magnetic resonance imaging (DCE-MRI) is considered the most accurate method for evaluating the extent of residual breast tumor after NAC (4,5). Moreover, the tumor biomarkers Ki-67 (a tumor proliferation marker) and P-glycoprotein (P-gp) are also widely recognized as important histopathologic NAC evaluation tools (6,7).
In order to improve prognostic predictions of patients with breast cancer, we evaluated MRI and tumor biomarkers as indicators of therapeutic response to NAC. We also investigated the correlation of the two methods.
Materials and methods
Patients
Between December 2009 and January 2013, 114 breast cancer patients treated at Huashan Hospital (Fudan University) were enrolled in this study. We used the AJCC (American Joint Committee on Cancer) Breast Cancer Staging System (7th edition, 2009) (8), to classify tumors by TNM staging. All patients were diagnosed with breast cancer based on the tumor tissues obtained by core needle biopsy before NAC. Patient demographics, clinical and imagining examinations, and tumor characteristics were collected.
The inclusion criteria of this study were as follows:
- Operable female patients;
- AJCC stage II (exclude T0N1M0 and T1N1M0) and AJCC stage III;
- No adjuvant treatments (chemotherapy, endocrine therapy, radiology therapy, targeting therapy) before NAC.
The exclusion criteria of this study were as follows:
- Pregnancy;
- Patients who had a previous history of breast cancer;
- AJCC stage IV patients.
Chemotherapy
All patients receiving NAC were given four courses of CET regimen chemotherapy at three week intervals [cyclophosphamide (500 mg/m2), epirubicin (75 mg/m2) and paclitaxel (175 mg/m2)]. All were given by intravenous bolus injection (9).
Pathological examination
The breast cancer tissue was collected by core needle biopsy (Mammotome System, 8 Gauge, Ethicon Inc., USA) before NAC treatment. After four courses of NAC, the surgically removed breast tissue was fixed in neutral buffered formalin and then embedded in paraffin. The specimens were cut into thin sections and stained with hematoxylin and eosin. Determination of P-gp and Ki-67 expression was made immunohistochemically. Standard immunohistochemistry (IHC) techniques were used. P-gp grading was as follows: 0, negative for cell membrane or fewer than 10% of cancer cells positive; 1, slightly positive in more than 10% of cancer cells; 2, moderately positive in more than 10% of cancer cells; 3, markedly positive in more than 10% of cancer cells. Ki-67 positive staining was defined as Ki-67 staining of 14% or more of cancer cell nuclei; Ki-67 negative staining was defined as Ki-67 staining of fewer than 14% of cancer cell nuclei (10).
Histopathologic criteria for assessment of NAC therapeutic response
The histopathologic therapeutic effects were determined based on the histopathologic criteria for assessment of therapeutic response in breast cancer described in the 2007 version of the Japanese Breast Cancer Society (JBCS) (11). In this study, we grouped the histopathologic therapeutic effects into three categories: (I) no pathological response (pNR); (II) partial pathological response (pPR, grade 1 and grade 2); and (III) pCR (grade 3).
MRI acquisition and assessment
To be eligible for the study, all patients were required to receive MRI scans before and after NAC treatment. Two experienced radiologists calculated the MR tumor size measurements using Functool SER software with a AW43 imaging processing workstation (GE Inc., USA). The radiologists were blinded to patient information, including breast cancer subtype, NAC regimen and final pathological outcome.
In accordance with the response evaluation criteria in solid tumors (RECIST) (12), MRI tumor size was determined by the longest tumor diameter. For multiple tumors, the maximal tumor diameters of the five largest lesions were measured. NAC treatment responses were based on DCE-MRI examinations. Responses were classified as follows: MRI complete response (mCR: no residual cancer); MRI partial response (mPR: ≥30% size reduction); MRI no response (mNR: <30% size reduction, including stable disease (SD); and progressive disease (PD).
Time-signal intensity curves (TIC, a measure of peak intensity from the time of contrast material injection) in DCE-MRI were designed to differentiate benign from malignant enhancing lesions based on region of interest (ROI) analysis. TICs were classified according to the shapes as type I, steady enhancement with a straight or curved time-signal intensity line; type II, plateau of signal intensity; or type III, washout of signal intensity.
Statistical analysis
Correlations between Ki-67, P-gp and pCR were derived using chi-square tests. The Kruskal-Wallis test was used to analyze the correlation between the histopathologic therapeutic responses (pCR, pPR and pNR), and Ki-67/P-gp expression. The NAC therapeutic effect was evaluated using Spearman’s rank correlation coefficient. The consistency between MRI responses and histopathologic therapeutic responses was tested using the Kappa test. Data was analyzed by SAS (Version 8.01; Statistical Analysis System Inc., North Carolina, USA). A Pvalue of less than 0.05 was considered statistically significant.
Results
Patient cohort
A total of 114 NAC-treated patients were included in the study. Twenty-eight patients (24.6%) reached a pCR after NAC treatment. At the start of the study, Ki-67 and P-gp were not used as routine test biomarkers; thus, 88 and 89 patients were analyzed for Ki-67 and P-gp expression, respectively, before NAC treatment. There were 19 pCR patients who tested positively for Ki-67 and 20 pCR patients who tested positively for P-gp. Three non-pCR patients were excluded due to the failure of the Ki-67 test. Thus, Ki-67 and P-gp tests were run on 66 and 69 non-pCR patients, respectively, following NAC treatment. Fifty five patients underwent complete MRI therapy before NAC treatment. Among them, six patients achieved a mCR. Thus, the MRI therapeutic response was evaluated in 49 patients after NAC treatment (Table 1).
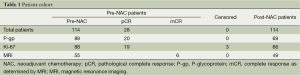
Full table
Patient characteristics
The clinical-pathologic characteristics of the 114 patients are shown in Table 2. The patients’ ages ranged from 29 to 74 years (mean 51.9 years). In terms of menopausal status, 68 patients (59.7%) were postmenopausal, and 46 patients (40.4%) were premenopausal. With regard to the pathologic category, invasive ductal carcinoma (76.3%) was the most commonly observed. With a cut-off for Ki-67 positivity of ≥14% positively stained tumor cells, most of the tumors (65.9%) were classified as having a high level of Ki-67. Fifty-two patients (58.4%) were P-gp positive before NAC. Twenty-eight patients (24.5%) achieved a pCR; 66 patients (57.9%) achieved a pPR, and 20 patients (17.5%) achieved a pPR.
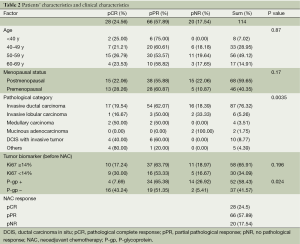
Full table
Correlations between patient characteristics and NAC histopathologic therapeutic effects
Table 2 shows the results of the univariate analyses for the factors associated with NAC histopathologic therapeutic effects. The association between NAC response versus age (P=0.87) and menopausal status (P=0.17) were not statistically significant. Compared with postmenopausal patients, premenopausal patients achieved slightly higher pCR and pPR rates (pCR: 28.3% vs. 22.1%; pPR: 60.9% vs. 55.9%), but a lower pNR rate (10.9% vs. 22.1%).
The predominant tumor type was invasive ductal carcinoma (76.3%). Patients having medullary carcinoma, or ductal carcinoma in situ (DCIS) with invasive tumors, achieved promising response rates (50% pCR, 50% pPR, and 40% pCR, 60% pPR, respectively). Two mucinous adenocarcinoma patients did not respond. These data indicated that pathologic type of tumor had a significant correlation with the NAC response (P=0.0035).
The pre-NAC Ki-67 expression was not a good predictor of NAC treatment response (P=0.196). P-gp negative patients achieved higher pCR rates than P-gp positive patients (43.2% vs. 7.7%, P=0.024).
Change in P-gp and Ki-67 status pre- and post-NAC
Figure 1A indicates that P-gp was up-regulated in 32 patients and down-regulated in 10 patients after NAC treatment. This change was statistically significant (P=0.001). Among the 16 pNR patients, P-gp was up-regulated in 13 patients, down-regulated in 1 patient and remained unchanged in 2 patients. Of the 53 pPR patients, P-gp was upregulated in 19, down-regulated in 9 and unchanged in 25. The up-regulation of P-gp was associated with a negative NAC therapeutic effect (P=0.024) (Figure 1B).
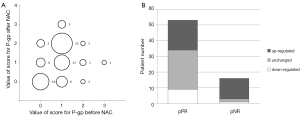
Figure 2A indicates that Ki-67 was down-regulated in 31 patients, and up-regulated in 2 patients after NAC treatment. The down-regulation of Ki-67 after NAC treatment was statistically significant (P<0.001), but it had no correlation with NAC therapeutic effect (P=0.9645) (Figure 2B).
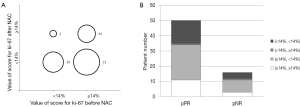
Correlations between MRI TIC type and NAC MRI/histopathologic therapeutic effect
Table 3 indicates that the pre-NAC TIC categories were not predictive for NAC therapeutic effects, by either MRI analysis (P=0.637) or by histopathologic analysis (P=0.503). Figure 3 shows the change of TIC categories pre-and-post NAC treatment: 21 patients (42.9%) remained unchanged; 19 patients (38.8%) moved into a higher TIC category; and 9 (18.4%) patients moved into a lower TIC category. However, the change of TIC category pre-and-post NAC treatment was not statistically significant (P=0.0872). It had no significant correlation with therapeutic effect, by either MRI analysis (P=0.948) or by histopathologic analysis (P=0.792).
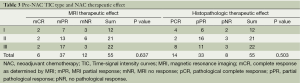
Full table
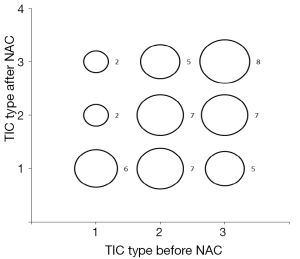
The equivalence of therapeutic effects as analyzed by MRI or histopathology
Table 4 indicates overlap of 4 mCR with 14 pCR patients (28.6%), and 2 mNR with 8 pNR patients (25.0%).Thus, MRI and the pathology response grades did not match well. In patients achieving a partial response, there was a better overlap of 23 mPR with 33 pNR patients (69.7%). However, although the data approached significance, it failed to reach statistical significance (P=0.056).
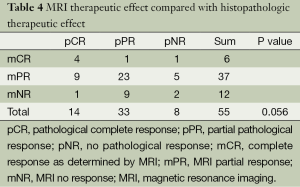
Full table
In Figure 4, we classified NAC treatment responses as pCR and non-pCR. Within the pCR group, we found 4 mCR in 14 pCR patients. This specificity was 28.6%. Within the group of 41 non-pCR patients, there were only 2 mCR patients. This sensitivity was 95.1%. Overall, the positive predictive value was 79.6% and the negative predictive value was 66.7%. However, the correlation between mCR and pCR was not statistically significant (P=0.29).
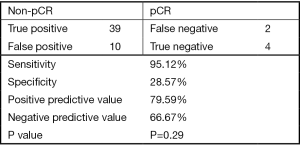
The average extent of the longest diameter in residual tumor by MRI analysis was 20.83±4.14 mm, and that of the surgically removed specimen, 18.89±3.71 mm. In 18 patients, the residual tumor extent was smaller by MRI analysis than the actual surgically excised specimen (max: –31.0 mm; mean: -10.67 mm). However, in 22 patients, the residual tumor extent by MRI analysis was larger than the actual surgically excised specimen (max: +43.0 mm; mean: +12.77 mm) (Figure 5).
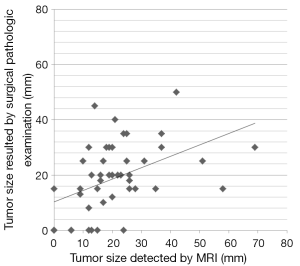
Discussion
Earlier studies of the prognostic assessment of breast cancer following NAC have focused on one of two aspects: (I) the biological and pathological analysis of surgical specimens pre/post treatment of NAC (13) or; (II) magnetic resonance imaging (MRI) to evaluate residual tumor extent after NAC (14). Traditional prognostic tumor biomarkers for breast cancer have been well studied. Imaging assessment of NAC treatment response, such as MRI, can provide valuable information about the residual tumor extent and enable better surgical plans to achieve a tumor-free margin. Complete response determined by MRI overlaps with complete response determined by pathological methods, for evaluating NAC response and predicting prognosis. In this current study, we combined the predictive use of pathological biomarkers with imaging findings, to evaluate their consistency and to identify discrepancies. The factors that influence accuracy were elucidated, to better guide clinical practice in the treatment of breast cancer.
Our study investigated DCE-MRI and the expression of tumor biomarkers, in an effort to explore their combined assessment value in NAC-treated breast cancer patients. IHC results indicated that lower pre-NAC P-gp expression was associated with better pCR rates. This suggests that P-gp is a good predictive tool for the efficacy of NAC. In addition, P-gp was significantly up-regulated after NAC treatment, the tendency of which was even more obvious in pNR patients. In other studies, it has been shown that P-gp expression is induced by NAC and its expression confers some degree of drug resistance (15). In this current study, Ki-67 expression was lower after NAC treatment, suggesting a decrease of tumor proliferation; however, its prognostic value for pCR is limited. The accuracy of MRI for evaluating NAC outcome and its consistency with pathological findings are inconclusive. Here, complete response as determined by MRI (mCR) was compared to complete response as determined by IHC (pCR). MRI analysis for pCR prediction was observed with high sensitivity, but with low specificity.
In recent years, proliferative tumor markers, including Ki-67, have been widely investigated as predictive and prognostic factors following NAC treatment. It has been shown that, in the neoadjuvant setting, a high level of Ki-67 expression predicts response to chemotherapy regimens (16), which may explain the high sensitivity of proliferating tumor cells to NAC compounds. Many studies have studied the correlation of Ki-67 to overall response rates (17-19), but with varying and conflicting results. de Azambuja et al. (20) analyzed the data from 29 studies, using patients with node-negative and node-positive breast cancer, and demonstrated that Ki-67 expression is associated with poor OS and disease-free survival. While other studies found that patients with high Ki-67 expression were more likely to achieve pCR (21), or Ki-67 shows no significant trend in relation to OS (22), Klintman et al. (23) have concluded that the value of Ki-67, as a prognostic tool, is restricted to ER-positive patients and to those with histological grade 2 tumors. There are some plausible reasons for these discordant results, including varying chemotherapy protocols, heterogeneous patient subtypes, and different Ki-67 standards and scoring systems.
Our study investigated the value of Ki-67 as a predictive factor and found that patients with low Ki-67 status pre-NAC were somewhat more likely to achieve pCR, although these results were not statistically significant. Interestingly, in this current study, most patients demonstrated a decrease of Ki-67 from high pre-treatment levels to low post-treatment levels. Our findings are consistent with previous studies that show Ki-67 low level expression (<3%) in healthy breast tissue (23), and a progressive increase of Ki-67 expression from benign breast disease, to DCIS, to invasive breast cancer (24). Our finding of Ki-67 reduction change suggests that NAC treatment induces a gradual downgrading from invasive carcinoma to DCIS, and may even promote eventual tumor disappearance.
The drug resistance of breast cancer is widely observed during chemotherapy, and various mechanisms have been characterized. Several xenobiotic transporter-related genes have been most frequently implicated as modifiers of NAC in breast cancer (25), and P-gp (encoded by MDR-1) is one of the key factors. P-gp is one member of the transmembrane efflux pump group, which is normally involved in the excretion of toxins from cells. P-gp has a broad range of substrates and exports a number of chemotherapeutics, inducing drug resistance (26). Some studies have investigated the relationship between P-gp expression levels and its prognostic value in breast cancer. Although a number of different conclusions have been drawn, the broad consensus is that P-gp expression can be induced by NAC (27,28). In our study, we found that 46.4% of patients showed P-gp up-regulation following chemotherapy for disease progression, a finding consistent with other studies (28,29). The reasons for this P-gp up-regulation include at least two possibilities. The first is our chemotherapy regimen of anthracycline and taxanes, which are both known substrates for P-gp (26). Here, P-gp negative cells are more sensitive and are killed by NAC treatment, while the primary high P-gp expressing tumor cells survive. The second possibility is acquisition of drug resistance after exposure to NAC treatment. Seven P-gp negative patients became P-gp positive, an indication of drug resistance. Regardless of whether P-gp expression is primary or acquired, high P-gp expression in either the pre- or post-treatment setting predicts poor patient outcome.
Some studies have reported that the decrease of tumor volume and enhancement on DCE-MRI is correlated with histopathologic response (30). Results from the I-SPY 1 trial (31) revealed that MRI findings of tumor reduction are better indicators of pathology than clinical examinations. In contrast, one study by Ko et al. (14) reported that ER positive breast cancer of a low nuclear grade might be associated with a poor response following NAC, and MRI might be less accurate in predicting the residual lesion extent of this kind of tumor.
In our study, the residual tumor extent on MRI and the surgically removed specimen size were analyzed. Based on the longest diameter of residue tumor, Pearson’s correlation coefficient between the two methods was 0.513 (P=0.0003), indicating a moderate correlation between the two measurements. However, the measurements by MRI included ten false-positive diagnoses. There are several possible reasons for this overestimation. (I) The residual tumor extent on MRI is in vivo. The tumor may shrink when fixed in formalin after surgical removal; (II) DCIS is often manifested as enhanced lesions on MRI images, which are similar to invasive tumors and difficult to differentiate from invasive tumors. Small residual lesions after NAC may often be misinterpreted as pCR, and residual DCIS tissue may lead to a false-positive diagnosis. Two of the 10 false positive lesions in our study were revealed to be DCIS, and were included in the pCR category. Although the impact of a DCIS diagnosis might be negligible in terms of prognosis, it may be an important risk factor for local recurrence after breast conserving surgery (32). Therefore, with DCIS tumors, overestimation on MRI is inevitable; (III) In another study, Ko et al. reported that a taxane-induced chemotherapy regimen may increase the vascular permeability, and capillary protein leakage could lead to augmented gadolinium uptake by the tumor, producing an over-or underestimation (14).
In conclusion, by studying the change of P-gp and Ki-67 expression from pre- to post-NAC, our investigation demonstrated that NAC treatment may arrest tumor proliferation, but may also potentially increase drug resistance. The pre-NAC P-gp status provided independent prognostic information for NAC treatment, while the pre-NAC Ki-67 status had little prognostic value. With rapidly evolving MRI technology, imaging assessment has been more widely applied to the evaluation of therapeutic effectiveness; it provides valuable information about the residual tumor. However, since the accuracy of MRI might be affected by many factors—the NAC-induced reactive change in tumor, the molecular character of the tumor, and the influence of chemotherapeutic agents—the discrepancies between imaging results and pathologic findings remain to be settled. In this retrospective study, we did not compare our chemotherapy regimen with more recently developed NAC chemotherapeutics. Therefore, our findings may not be applicable to newer therapeutics and will require further investigation.
Acknowledgements
Funding: This study was supported by funding (grant number 114119B2500) from the Shanghai Committee of Science and Technology, China.
Disclosure: The authors declare no conflict of interest.
References
- Alvarado-Cabrero I, Alderete-Vazquez G, Quintal-Ramirez M, et al. Incidence of pathologic complete response in women treated with preoperative chemotherapy for locally advanced breast cancer: correlation of histology, hormone receptor status, Her2/Neu, and gross pathologic findings. Ann Diagn Pathol 2009;13:151-7. [PubMed]
- Gonzalez-Angulo AM, McGuire SE, Buchholz TA, et al. Factors predictive of distant metastases in patients with breast cancer who have a pathologic complete response after neoadjuvant chemotherapy. J Clin Oncol 2005;23:7098-104. [PubMed]
- Charfare H, Limongelli S, Purushotham AD. Neoadjuvant chemotherapy in breast cancer. Br J Surg 2005;92:14-23. [PubMed]
- Londero V, Bazzocchi M, Del Frate C, et al. Locally advanced breast cancer: comparison of mammography, sonography and MR imaging in evaluation of residual disease in women receiving neoadjuvant chemotherapy. Eur Radiol 2004;14:1371-9. [PubMed]
- Akazawa K, Tamaki Y, Taguchi T, et al. Preoperative evaluation of residual tumor extent by three-dimensional magnetic resonance imaging in breast cancer patients treated with neoadjuvant chemotherapy. Breast J 2006;12:130-7. [PubMed]
- Tsukamoto F, Shiba E, Taguchi T, et al. Immunohistochemical Detection of P-glycoprotein in Breast Cancer and Its Significance as a Prognostic Factor. Breast Cancer 1997;4:259-63. [PubMed]
- Dowsett M, Nielsen TO, A’Hern R, et al. Assessment of Ki67 in breast cancer: recommendations from the International Ki67 in Breast Cancer working group. J Natl Cancer Inst 2011;103:1656-64. [PubMed]
- Edge SB, Compton CC. The American Joint Committee on Cancer: the 7th edition of the AJCC cancer staging manual and the future of TNM. Ann Surg Oncol 2010;17:1471-4.
- Yao X, Hosenpud J, Chitambar CR, et al. A phase II study of concurrent docetaxel, epirubicin and cyclophosphamide as a neoadjuvant chemotherapy regimen in patients with locally advanced breast cancer. J Cancer 2012;3:145-51. [PubMed]
- Cheang MC, Chia SK, Voduc D, et al. Ki67 index, HER2 status, and prognosis of patients with luminal B breast cancer. J Natl Cancer Inst 2009;101:736-50. [PubMed]
- Kurosumi M, Akashi-Tanaka S, Akiyama F, et al. Histopathological criteria for assessment of therapeutic response in breast cancer (2007 version). Breast Cancer 2008;15:5-7. [PubMed]
- Watanabe H, Okada M, Kaji Y, et al. New response evaluation criteria in solid tumours-revised RECIST guideline (version 1.1). Gan To Kagaku Ryoho 2009;36:2495-501. [PubMed]
- Railo M, Lundin J, Haglund C, et al. Ki-67, p53, ER receptors, ploidy and S phase as long-term prognostic factors in T1 node-negative breast cancer. Tumour Biol 2007;28:45-51. [PubMed]
- Ko ES, Han BK, Kim RB, et al. Analysis of factors that influence the accuracy of magnetic resonance imaging for predicting response after neoadjuvant chemotherapy in locally advanced breast cancer. Ann Surg Oncol 2013;20:2562-8. [PubMed]
- Rudas M, Filipits M, Taucher S, et al. Expression of MRP1, LRP and Pgp in breast carcinoma patients treated with preoperative chemotherapy. Breast Cancer Res Treat 2003;81:149-57. [PubMed]
- Yerushalmi R, Woods R, Ravdin PM, et al. Ki67 in breast cancer: prognostic and predictive potential. Lancet Oncol 2010;11:174-83. [PubMed]
- Caudle AS, Gonzalez-Angulo AM, Hunt KK, et al. Predictors of tumor progression during neoadjuvant chemotherapy in breast cancer. J Clin Oncol 2010;28:1821-8. [PubMed]
- Stuart-Harris R, Caldas C, Pinder SE, et al. Proliferation markers and survival in early breast cancer: a systematic review and meta-analysis of 85 studies in 32,825 patients. Breast 2008;17:323-34. [PubMed]
- Molino A, Pedersini R, Micciolo R, et al. Relationship between the thymidine labeling and Ki-67 proliferative indices in 126 breast cancer patients. Appl Immunohistochem Mol Morphol 2002;10:304-9. [PubMed]
- de Azambuja E, Cardoso F, de Castro G Jr, et al. Ki-67 as prognostic marker in early breast cancer: a meta-analysis of published studies involving 12,155 patients. Br J Cancer 2007;96:1504-13. [PubMed]
- Penault-Llorca F, Abrial C, Raoelfils I, et al. Changes and predictive and prognostic value of the mitotic index, Ki-67, cyclin D1, and cyclo-oxygenase-2 in 710 operable breast cancer patients treated with neoadjuvant chemotherapy. Oncologist 2008;13:1235-45. [PubMed]
- Pinto AE, Andre S, Pereira T, et al. Prognostic comparative study of S-phase fraction and Ki-67 index in breast carcinoma. J Clin Pathol 2001;54:543-9. [PubMed]
- Klintman M, Bendahl PO, Grabau D, et al. The prognostic value of Ki67 is dependent on estrogen receptor status and histological grade in premenopausal patients with node-negative breast cancer. Mod Pathol 2010;23:251-9. [PubMed]
- Allred DC, Mohsin SK, Fuqua SA. Histological and biological evolution of human premalignant breast disease. Endocr Relat Cancer 2001;8:47-61. [PubMed]
- Amiri-Kordestani L, Basseville A, Kurdziel K, et al. Targeting MDR in breast and lung cancer: discriminating its potential importance from the failure of drug resistance reversal studies. Drug Resist Updat 2012;15:50-61. [PubMed]
- Leonard GD, Fojo T, Bates SE. The role of ABC transporters in clinical practice. Oncologist 2003;8:411-24. [PubMed]
- Rivera E. Implications of anthracycline-resistant and taxane-resistant metastatic breast cancer and new therapeutic options. Breast J 2010;16:252-63. [PubMed]
- Kim B, Fatayer H, Hanby AM, et al. Neoadjuvant chemotherapy induces expression levels of breast cancer resistance protein that predict disease-free survival in breast cancer. PLoS One 2013;8:e62766. [PubMed]
- Burger H, Foekens JA, Look MP, et al. RNA expression of breast cancer resistance protein, lung resistance-related protein, multidrug resistance-associated proteins 1 and 2, and multidrug resistance gene 1 in breast cancer: correlation with chemotherapeutic response. Clin Cancer Res 2003;9:827-36. [PubMed]
- Martincich L, Montemurro F, De Rosa G, et al. Monitoring response to primary chemotherapy in breast cancer using dynamic contrast-enhanced magnetic resonance imaging. Breast Cancer Res Treat 2004;83:67-76. [PubMed]
- Hylton NM, Blume JD, Bernreuter WK, et al. Locally advanced breast cancer: MR imaging for prediction of response to neoadjuvant chemotherapy--results from ACRIN 6657/I-SPY TRIAL. Radiology 2012;263:663-72. [PubMed]
- Moon HG, Han W, Lee JW, et al. Age and HER2 expression status affect MRI accuracy in predicting residual tumor extent after neo-adjuvant systemic treatment. Ann Oncol 2009;20:636-41. [PubMed]