Pediatric heart transplantation—indications and outcomes in the current era
Introduction
The field of pediatric heart transplantation (HTx) has progressed significantly since Dr. Adrian Kantrowitz transplanted the heart of a brain dead infant into another infant in 1967 (1). Based on the most recent data, there are now approximately 100 centers performing over 500 pediatric heart transplants yearly worldwide (2). Orthotopic HTx has become an acceptable treatment strategy and the standard of care for end-stage heart disease in children, whether secondary to underlying congenital heart disease (CHD) or cardiomyopathy.
The United Network for Organ Sharing (UNOS) and the International Society for Heart and Lung Transplantation (ISHLT) maintain multicenter databases and collaboratives which have helped forge medical and surgical progress. In addition, the Pediatric Heart Transplant Study (PHTS) was founded in 1991 and is dedicated to the advancement of the science and treatment of children during listing for and following HTx. The purposes of the PHTS are to establish and maintain an international, prospective, event driven database for HTx, to use the database to encourage and stimulate basic and clinical research in the field of pediatric HTx and to promote new therapeutic strategies. The PHTS is unique in that its data entry is event-driven both pre- and post-transplantation, so events such as annual follow-up, development of cardiac allograft vasculopathy (CAV), and rejection are captured in addition to transplantation and death. Through the data provided by these organizations and single-center studies, the field of pediatric heart transplant has and will continue to advance.
Since the initial transplantation performed by Dr. Kantrowitz, advances in surgical technique, understanding of rejection and immunology, immunosuppressive medications, and treatment for rejection have led to improved outcomes. In addition, improved palliation for complex CHD has helped define those patients who should be considered for pediatric HTx. This article reviews the indications for orthotopic HTx and outcomes in the pediatric population.
Indications
Past guidelines for pediatric HTx have been broadly defined (3,4). Since these guidelines, there have been improvements in surgical palliation for hypoplastic left heart syndrome (HLHS) (5,6), improved understanding of certain diseases such as restrictive cardiomyopathy (RCM) (7,8), extrapolation of heart failure management from adult literature to the pediatric population (9-12), and increasing retransplantation (2)—all of which have led to the need for guideline revision. In addition, adult heart failure has been defined into four stages (13): (I) stage A (at risk); (II) stage B (pre-clinical, asymptomatic); (III) stage C (past/present history of heart failure with symptoms); and (IV) stage D (end-stage heart failure). This staging system has been incorporated into published guidelines for the treatment of pediatric heart failure (14). The American Heart Association commissioned a working group to reassess the indications for pediatric HTx, and the recommendations were published in 2007 (15). These indications are largely based on level C evidence indicating expert clinical opinion (Table 1). Repeat transplantation occurs rarely in pediatric populations and, as expected, is associated with a worse outcome as compared to primary transplantation. Indications for repeat transplantation are outlined in Table 2 and all recommendations are based on level B evidence (data derived from nonrandomized studies).
The improved outcomes in surgical correction and palliation in children with CHD have led to an increasing population of adults with CHD who may develop complications and indications for HTx. This population often warrants increased evaluation of organ systems, including pulmonary function, liver function/cirrhosis, and renal function, given the long-standing effects of palliated CHD on the various organ systems. The published guidelines previously mentioned specifically address the indications and contraindications in this population (15).
The guidelines also outline recommendations where the risk outweighs the benefit and would be considered contraindications to transplantation and retransplantation (15). For example, the efficacy of transplant has not been established in those patients with a history of (I) infection with hepatitis B or C, or human immunodeficiency virus; (II) recent illicit drug or tobacco, or alcohol abuse; and (III) poor psychosocial support and medical non-compliance. Similarly, multisystem organ failure or a progressive and irreversible multisystem disease process precludes HTx. Finally, primary transplant for CHD in which palliative surgery is feasible is not recommended. As for repeat transplantation, there are two main concerns emphasized: (I) retransplantation should not be performed during an ongoing acute allograft rejection episode even in the presence of graft vasculopathy; and (II) retransplantation is not efficacious when performed during the first 6 months after the primary transplant.
There are several notable revisions in these guidelines. For example, RCM is an indication for HTx when associated with reactive pulmonary hypertension. Additionally, due to a limited pediatric donor pool, primary HTx for CHD is not recommended unless there are additional confounding variables such as ventricular dysfunction, significant valvar insufficiency, or severe coronary anomalies. These recommendations also acknowledge the mortality and morbidity associated with pulmonary hypertension, severe valvar insufficiency not amenable to surgery, and protein losing enteropathy in previously repaired or palliated CHD thus translating into indications for HTx.
In addition to the guidelines, certain diagnoses account for the majority of pediatric HTx, including cardiomyopathies and CHD, most notably HLHS and pulmonary atresia with intact ventricular septum. However, other indications for transplant may include refractory arrhythmias and malignancies.
Special considerations/populations
Allosensitization
Allosensitization or highly-sensitized patients are usually defined as having an elevated panel reactive antibody >10%. While human leukocyte antigen (HLA) sensitization is uncommon in patients with cardiomyopathy, it can frequently be seen in patients with CHD who have had prior surgeries. It is accepted that the use of cryopreserved allograft material induces an immune response with the development of both class I and II anti-HLA antibodies and elevated panel reactive antibodies (16,17). In addition to allograft exposure, blood transfusions, mechanical circulatory support, pregnancy, and prior HTx have also been shown to be risk factors for developing anti-HLA antibodies. Studies have shown that transplantation in the setting of allosensitization carries increased risk and mortality (18-21). Given this increased risk, some centers may choose not to offer HTx to patients with elevated panel reactive antibody or may result in increased waitlist times.
Alternatively, desensitization (decreasing the circulating anti-HLA antibodies) or prospective/virtual crossmatching may be alternatives to improve outcomes in the setting of allosensitization. Many studies have reported methods to desensitize patients, including administration of IVIG, plasmapheresis, and use of cyclophosphamide or mycophenolate mofetil (22-25). In addition, newer medications, including rituximab (a monoclonal antibody to CD20) and bortezomib (a proteasome inhibitor directed against plasma cells) have been shown to reduce circulating antibodies (26-29). As opposed to desensitization, prospective crossmatching aims to avoid the potential reaction between the donor and recipient. Unfortunately, prospective crossmatching can be time consuming and requires the presence of both recipient serum and donor cells to perform a direct assessment of the donor-recipient crossmatch. This can be limited by geographical proximity. Alternatively, many advocate for the use of a virtual crossmatch in which the recipient anti-HLA antibody profile is compared to the donor HLA typing to predict a possible crossmatch alleviating the geographic restrictions placed by the direct, prospective crossmatch (30-32).
ABO-incompatible transplantation
Infants currently have the longest waiting time for HTx (33). As such, ABO-incompatible HTx has become increasingly more frequent as a means to decrease potential waiting time. Currently, UNOS guidelines permit ABO-incompatible HTx in children <1 year of age with any isohemagglutinin titer and for infants between 1 and 2 years of age with isohemagglutinin titers ≤1:4. ABO-incompatible eligible infant listing has increased from 0% prior to 2002 to 53% is 2007 (34). Unfortunately, when Almond et al. compared ABO-incompatible listed infants to those listed exclusively for ABO-compatible transplantation, there was no difference in waitlist mortality (34). Infants with blood type O were more likely to undergo transplantation by 30 days from listing when listed for ABO-incompatible heart transplant, but this did not hold true for infants listed with either A or B blood types (34). When comparing ABO-incompatible listed infants to those listed for ABO-compatible transplantation, studies have demonstrated they are more likely to require extracorporeal membrane oxygenation (ECMO), mechanical ventilation, and have renal failure, suggesting this listing strategy is still employed in a more ill population which may account for the similar waitlist mortality between the two groups (34,35).
Regardless of the listing strategy, ABO-incompatible heart transplant recipients have similar outcomes to those undergoing ABO-compatible transplantation. Review of the PHTS data demonstrated similar 1-year survival between ABO-incompatible and ABO-compatible infant heart transplants, 82% vs. 84%, respectively (35). Comparable results in short-term survival, long-term survival, rejection, and CAV have been borne out by review of the UNOS registry and by Dipchand et al. (36,37). In addition to infants and young children, Urschel et al. demonstrated that ABO-incompatible HTx can also be performed in older children (up to 90 months in their cohort) and with higher isohemagglutinin titers (up to 1:64 in their cohort) (38). Further studies demonstrating safety and equivalent outcomes could open this opportunity to a much larger population of children.
Fetal listing
Fetal listing for HTx has been proposed as a means to increase the potential window for transplantation. While there are no specific indications for fetal listing, it has historically been utilized when considering primary HTx for left-sided obstructive lesions, such as HLHS. Current UNOS guidelines allow for fetal listing between 32 and 36 weeks gestation after thorough fetal evaluation for viability has been completed, and if the fetus does not undergo transplantation prior to delivery, the waitlist time restarts after delivery as to not disadvantage those listed after birth (39). Fetal listing is currently a rare entity with PHTS registry data indicating showing fetal listing in 46 of the 4,365 (1%) patients between 1993 and 2009 (40). However, there is clearly institutional variation as Pollock-BarZiv et al. reported 26 fetal listings of 269 total listings between 1990 and 2006 (this institution is a participating center in the PHTS and is included in the previously mentioned PHTS data) (41). The recent PHTS data demonstrated similar overall waiting times between the fetal and neonatal listing group, but it is worth noting that the patients listed prenatally had a shorter postnatal waiting time (40). Interestingly, in the cohort reported by Pollock-BarZiv et al., two of the fetal listing patients were delivered via cesarean section when a donor became available, and seven of the 26 fetal listings were delisted after delivery (41). The latter statistic poses an intriguing question of whether physicians can truly predict who will require HTx as a fetus, and whether the option of fetal listing by UNOS should persist, as it is currently being considered for elimination.
Outcomes
General outcomes
With collective experience over each era, pediatric HTx outcomes continue to improve. The most recent data from the ISHLT, including patients from 1982 through June 2011, demonstrates the median survival is 19.7 years for infants, 16.8 years for children ages 1-5 years, 14.5 years for children ages 6-10 years, and 12.4 years for children 11-17 years of age at the time of transplantation (Figure 1A) (2). The highest mortality rate remains the during the first year post-transplant, and when accounting for conditional survival during the first year, the median survivals increase to 20.6 years for children ages 1-5 years, 16.7 for children ages 6-10 years, and 16.1 years for children 11-17 years of age (Figure 1B) (2). The decreasing median survival in older age groups is likely multifactorial and related to several factors including, the relative immature immune system in the infants and lack of preformed antibodies, sensitization in the older children due to surgical repair and palliation for CHD, and risk-taking behaviors such as medication non-compliance in older children. Additional factors are discussed below. Recent analysis of the PHTS registry demonstrated an overall survival of 83% at 5 years after transplantation in the most recent era [2005-2009] (42). While these outcome data are limited to 5 years of follow-up, a significant increase in survival was noted at 5 years post-transplant between the most recent era and those transplanted between 2000 and 2004, 83% vs. 76%, respectively (42). This study also assessed many variables that affect outcomes, many of which are outlined in this review.
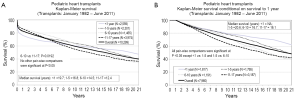
Donor variables have also been shown to affect pediatric heart transplant outcomes. Factors previously thought to negatively impact post-transplant survival, such as donor cause of death, need for inotropic support, and cardiopulmonary resuscitation, have been recently shown to have no significant impact on outcomes (43). Gender mismatch between the donor and recipient has also been shown not to affect the post-transplant survival (44). However, recent analysis of the PHTS registry did demonstrate that longer ischemic times (>300 min) adversely impacted survival at 1 year but not overall survival, and the effect of ischemic time was a greater factor for patients >10 years of age (43). While the donor ischemic time is dependent upon donor variables, e.g., proximity to the recipient, it can also be dependent upon recipient variables, including complex CHD and the number of prior sternotomies.
Despite improved post-transplantation outcomes and advances in cardiovascular support for those awaiting transplantation, a relative shortage of organs persists, and waitlist mortality remains an important topic. Analysis of the United States Scientific Registry of Transplant Recipients (SRTR) has shown a waitlist mortality of 17% for pediatric HTx (45), and others have shown that waitlist mortality is as high as 23% by 6 months after listing in the highest risk group—infants (33). In addition, several factors have been found to be associated with increased waitlist mortality, including the need for ECMO or mechanical ventilation, status 1A listing, diagnosis of CHD (with or without prior surgery), the need for dialysis, weight <3 kilograms, and non-white race (33,45,46). In 2006, changes were made to the organ allocation system resulting in broader regional sharing, and this has been shown to decrease the risk of waitlist mortality or becoming too ill to transplant by 17% in the adult population but has not been studied in pediatric HTx (47). Despite multiple studies demonstrating risk factors for waitlist mortality in pediatric patients, the current allocation algorithm for pediatric HTx remains imperfect and does not distinguish between a single high-dose inotrope and more aggressive means of support, such as mechanical circulatory support or mechanical ventilation. However, there is ongoing debate regarding the current allocation system with potential changes to more closely mirror the adult allocation algorithm looming in the future. Ideally, revising the allocation system to reflect the risk factors above would result in improved waitlist mortality for children awaiting HTx, but follow-up and review would be necessary if these changes manifest.
Specific diseases and their outcomes
Cardiomyopathy
Cardiomyopathy is the most common indication for pediatric HTx, ranging from 41% of patients <1 year of age to 65% of patients between 11 and 17 years of age (2), and has become an increasing indication for pediatric HTx over the past three decades (2,48). This group is comprised of dilated cardiomyopathy (DCM), hypertrophic cardiomyopathy (HCM), and RCM. Some patients may also manifest a mixed phenotype with characteristics of both RCM and DCM or HCM.
Dilated cardiomyopathy (DCM)
The incidence of DCM is 0.58 cases per 100,000 children and accounts for over 50% of cardiomyopathies in the U.S (49). Based on the Pediatric Cardiomyopathy Registry (PCMR) data, the majority of pediatric DCM cases (66%) are idiopathic but may be due to myocarditis, neuromuscular disease, or genetic causes (50). Neuromuscular disorders and metabolic disorders may have decreased life expectancies or survival rates lower than their freedom from transplantation which must be factored when assessing for HTx. The freedom from death or transplantation at 1 and 5 years after DCM diagnosis was 69% and 54%, respectively, and risk factors for death or transplantation included age >6 years or congestive heart failure at presentation and lower left ventricular echocardiographic fractional shortening (50). However, myocarditis as an etiology for DCM was associated with decreased risk compared to idiopathic DCM (50).
Analysis of the PHTS registry demonstrated relatively low waitlist mortality (11%) for patients with DCM listed for transplantation, but factors including history of mechanical ventilation and presence of arrhythmias did increase the risk of death while awaiting transplantation (51). In addition, a recent study utilizing both PCMR and PHTS registries found that older age at diagnosis, in addition to ventilator use, was an additional risk factor for death on the waitlist (52). Singh et al. demonstrated excellent short-term post-transplant survival for patients with DCM with 30-day and 1-year survivals of 98% and 94%, respectively (53). Based on the most recent PHTS data, 74% of pediatric patients with DCM listed for HTx ultimately underwent transplant, and their 10-year post-transplant survival was 72% (Figure 2) (51). Risk factors associated with death post-transplantation included black race, older age, mechanical ventilation at transplant, longer ischemic time, and earlier era of transplantation (51). The outcomes for patients with DCM post-transplant were noted to better than those for other forms of pediatric cardiomyopathy (51,54,55).
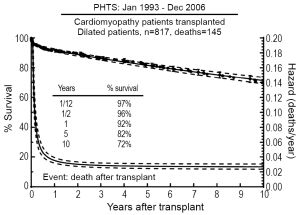
Hypertrophic cardiomyopathy (HCM)
The incidence of HCM is 0.47 cases per 100,000 children and accounts for 42% of pediatric cardiomyopathy (49). HCM may be idiopathic, familial, associated with neuromuscular disorders, or associated with certain syndromes such as Noonan’s-spectrum syndromes (e.g., Noonan’s syndrome, LEOPARD syndrome, Costello syndrome) and Beckwith-Weidemann syndrome. While it is well-accepted that these patients are at risk for arrhythmias and sudden cardiac death, a recent study demonstrated that heart failure deaths were at least as common as sudden cardiac death in pediatric HCM patients (56). HCM is an infrequent etiology for pediatric HTx, accounting for 5-6% of transplantations (54,57). While it makes up a minority of pediatric HTx, several risk factors have been identified those at increased risk for death or transplantation, including age <1 year old, low weight, lower left ventricular fractional shortening, or higher end-diastolic left ventricular posterior wall or septal thickness at the time of diagnosis (58,59). In children with HCM, abnormal blood pressure response to exercise has also shown to be predictive of poor outcomes (56). In a recent large retrospective study from the PCMR, which included 1,085 children with HCM, rates of death or HTx were highest in those populations with inborn errors of metabolism (57% at 2 years from diagnosis) and with mixed phenotypes (45% at 2 years for HCM/DCM and 38% at 2 years for HCM/RCM) (58). This is further supported by a recent study that demonstrated restrictive physiology (defined by echocardiographic parameters) in the presence of HCM conferred a 3.5-fold increased risk of hospitalization and 5.7-fold increased risk of death or transplantation (60). The risk of death or HTx also increases with the presence of increasing number of risk factors (58).
The waitlist mortality for patients with HCM is higher compared to those with DCM (14% vs. 11%), and identified risk factors for waitlist mortality in this cohort include UNOS status 1 and younger age (51,54). Waitlist mortality has been shown to be consistently higher in infants with HCM compared to other age groups with HCM (54,61). The 10-year survival post-transplant for patients with HCM in the PHTS registry is 47% which is significantly less than both the DCM cohort and the non-cardiomyopathy cohort in the registry, 72% and 63% respectively (51,54).
Restrictive cardiomyopathy (RCM)
RCM is the rarest form of pediatric cardiomyopathy and is characterized by “normal or decreased volume of both ventricles associated with biatrial enlargement, normal left ventricular wall thickness and atrioventricular valves, impaired ventricular filling with restrictive physiology, and normal (or near normal) systolic function.” (62). The incidence of RCM is 0.03-0.04 cases per 100,000 children (49,63) and accounts for 4.5% of pediatric cardiomyopathies (64). Analysis of the PCMR database demonstrated approximately 1/3 of patients with RCM had a mixed phenotype (RCM/HCM) (64). Historically, pediatric patients with RCM have been shown to have a poor prognosis with a mortality rate of 63% at 3 years from diagnosis (65) and 75% at 6 years from diagnosis (66). Syncope and evidence of ischemia are poor prognostic signs (67). This poor prognosis, along with the risk of progressive, irreversible pulmonary hypertension, thromboembolic events, sudden death, and the limited medical treatment options, has led to some centers listing for HTx at the time of diagnosis. This has skewed the assessment of the natural history of the disease, but recent review of the PCMR demonstrated the cumulative incidence of death was 20% at 5 years from diagnosis in the pure RCM group and 28% at 5 years from diagnosis in the RCM/HCM group (64). In addition, the cumulative incidence of HTx was 58% at 5 years from diagnosis in the pure RCM group and 30% at five years from diagnosis in the RCM/HCM group (64). Given the potential risks in this population, close observation is warranted and early listing for HTx should be considered.
Patients with RCM listed for HTx had 10% waitlist mortality, and identified risk factors for waitlist mortality were similar to the other cardiomyopathy cohorts and include younger age, ventilator dependence, UNOS status 1, ECMO, ventricular assist device, intra-aortic balloon pump, and inotrope use (55). The 10-year survival outcome for patients with RCM was better than those patients with HCM, but not as good as those with DCM, 63% vs. 47% vs. 72% respectively (51,54,55). Risk factors for death post-transplant included earlier era of transplant in the early phase and older age (10 vs. 5 years) and black race are in the constant phase (55).
Congenital heart disease (CHD)
As previously discussed, advances in surgical technique and outcomes continue to redefine the population of CHD patients undergoing HTx. This population includes infants with both unrepaired and palliated complex CHD and adults with palliated CHD who either have failed palliations or ventricular dysfunction. While ISHLT data continue to show cardiomyopathy is the most frequent indication for pediatric heart transplant worldwide (2), large-volume center data in the United States demonstrates increasing incidence of transplantation for CHD. Voeller et al. reported 57% (173/307) of their HTxs were for CHD, and of those, 80% had single-ventricle anatomy (48). In addition, for their most recent cohort [2002-2009], the most common indication for HTx in patients with CHD was failed single-ventricle palliation (48).
This is particularly relevant in patients with HLHS with several studies demonstrating improved outcomes in staged palliations for a disease that was previously considered frequently for primary transplantation (5,6,68). These improved outcomes have resulted in (I) decreased utilization of primary transplant as a treatment for HLHS (69,70); (II) utilization of transplantation at most institutions for patients with HLHS and complications such as significantly depressed right ventricular function or significant tricuspid valve regurgitation; and (III) an increase in the number of patients with HLHS with prior surgical interventions proceeding to HTx as many of these patients have had some form of prior palliation. In addition, the success with HLHS has led to utilization of HTx in other univentricular conditions such as pulmonary atresia with intact ventricular septum associated with right-ventricular dependent coronary circulation and ostial stenosis/atresia as well as complex heterotaxy syndromes (71-74). While most institutions proceed with palliation as an initial first step for complex CHD with univentricular physiology, Auerbach et al. demonstrated a better graft survival (median graft survival 18 years compared to 8 years) and decreased incidence of acute rejection in those patients with univentricular hearts that had not undergone prior surgical procedures (75). Despite this, many patients will require some form of palliation, especially neonates, in order to bridge to HTx given current wait times.
In addition to risk of transplant early in life, these patients are also at risk for transplantation following the superior cavopulmonary anastomosis (Glenn procedure) or following total cavopulmonary anastomosis (Fontan procedure). Of particular interest in recent years is the “failed-Fontan” patient. A failed-Fontan can manifest as systolic ventricular dysfunction, alterations in the structure and function of the pulmonary vascular bed, significant atrioventricular valve insufficiency, arrhythmia, plastic bronchitis, or protein-losing enteropathy (PLE) (76-80). Fontan conversion with arrhythmia surgery has been utilized in select patients (81-83). While the staged palliation approach, including the Fontan procedure, have increased transplant-free survival, it can increase risk for future transplant given the potential of allosensitization which has portended a worse outcome (84). In addition, UNOS status 1 at listing, ventilator support, and a time interval of less than 6 months from the initial Fontan palliation have been shown to be risk factors for death after listing for transplant in the failed-Fontan population (70). For those patients who develop plastic bronchitis, transplant may be considered given the risk for life-threatening events. While literature is limited, recent review of the PHTS data demonstrated these patients may have an increased short-term mortality (70% survival at 30 days) but comparable long-term outcomes by 5 years post-transplantation (85). For those with PLE, HTx has provided complete resolution of PLE (86-88). Unfortunately, PLE may recur in this patient population group suggesting they may be more sensitive to complications such as restrictive physiology in the setting of CAV, but repeat transplantation has also been shown to be potentially curative (89).
Post-transplantation complications
Rejection
Rejection remains one of the main post-transplant complications limiting long-term graft survival, and it can occur at any point after placement of the graft. Data from the PHTS has shown that incidence and prevalence of rejection has decreased over time (study period January 1993-December 2005), but the incidence of rejection with hemodynamic compromise and mortality from rejection have remained stable (90). In the most recent era (July 2008-June 2012), 22% of children will experience rejection during the first year post-transplant, and this is decreased from 34% from the preceding era (July 2004 - June 2008) (Figure 3) (2). Data from the PHTS show demonstrate 64% of patients were free of rejection in the first year (36% of patients experiencing rejection) and a 5-year freedom from rejection of 52% (Figure 4) (42). This difference is likely related to the difference in how rejection is classified between the two databases. In addition, treated rejection during the first year post-transplantation has been shown to significantly decrease long-term survival (88% vs. 80% patient survival at 5 years post-transplant) (2). Additional data from the PHTS have shown that late rejection, occurring >1 year after transplant, has decreased in the recent era, but there has been no decrease in the association between late rejection and CAV and mortality (91). Older age, African-American race, and elevated PRA have been shown repeatedly to be risk factors for rejection (92-95), and early rejection has been shown to be a risk factor for late rejection (91). Not surprisingly, non-adherence has also been shown to be a risk factor for late-rejection (96).
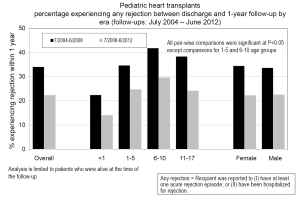
Interestingly, while the use of induction immunosuppression following HTx has increased, there has been no significant change in the amount of rejection. According to the most recent data from the ISHLT, 58% of children receiving a heart transplant between January 2001 and June 2012 received some form of induction immunosuppression, with approximately two-thirds of those receiving a polyclonal antilymphocyte or antithymocyte globulin and approximately one-third receiving an IL-2 receptor antagonist. This has increased from the data reported in 2003, where approximately 40% of children received some form of induction (97). In the most recent ISHLT Registry report, there were no differences in the percentages of patients experiencing rejection comparing induction, whether being a polyclonal or IL-2 receptor antagonist, to those who did not receive induction immunosuppression (2).
Maintenance immunosuppression has also impacted the incidence of rejection. In the most recent ISHT Registry report, tacrolimus has been shown to be associated with a lower incidence of rejection (when assessing for both any episode of rejection and for only treated episodes of rejection) compared to cyclosporine, whether with or without induction immunosuppression (2). A similar picture is seen when comparing tacrolimus and cyclosporine combined with either mycophenolic acid or mycophenolate mofetil, but no difference was noted when comparing tacrolimus to cyclosporine when combined with azathioprine (2).
Infections
Infection remains an important cause of morbidity and mortality and accounts for approximately 12% of deaths during the first year following transplantation (2). Immunosuppression to prevent rejection renders the host potentially susceptible to infection, particularly opportunistic infections. These infections can occur across all ages, but one single-center study demonstrated that infants were more likely to experience more severe and chronic infections (98). In current practice, most patients receive antibiotic prophylaxis, including both bacterial and viral [cytomegalovirus (CMV)] prophylaxis at least for a period of time.
Common bacterial infections include Staphylococcus species, Pseudomonas species, and Enterobacter cloacae, which are commonly encountered in the early post-transplant period and may be nosocomial (99,100). Streptococcus pneumoniae becomes a more common source of pulmonary and hematologic infection 1 year after transplantation (101,102). Unfortunately, various studies have shown that pediatric transplant recipients mount a lower response to pneumococcal vaccination (103,104).
CMV is the most common viral infection and has a peak hazard occurring 6-8 weeks after transplantation (99). While CMV can cause disease directly, it has also been show to play a role in acute rejection, graft vasculopathy, and post-transplant lymphoproliferative disease (PTLD) (105-107). Those patients who are CMV seronegative and receive a seropositive donor organ are the highest risk of developing infection. As such, prophylactic antiviral treatment, including ganciclovir, valganciclovir, or acyclovir, is recommended for 3 months in the high-risk recipient and 1-3 months for all other recipients (108). Other common viral infections include Epstein-Barr virus (EBV), herpes simplex virus (HSV), varicella zoster virus (VZV), and influenza viruses. EBV is a human herpes virus that causes a spectrum of disease, ranging from mononucleosis to PTLD, which will be discussed later (109). HSV typically affects the skin and oral mucosa but can involve other organs such as the lungs. This can be related to primary infection or reactivation after transplantation. Varicella infection post-transplantation has been shown to be nearly equally divided between both primary infection and reactivation (110). Acyclovir treatment is indicated for treatment of varicella infection, and administration of varicella zoster immunoglobulin within 48 hours of exposure is indicated for prevention.
Fungal infections are relatively uncommon following pediatric HTx. Based on PHTS registry data, fungal infections account for 6.8% of post-transplant infections (111). Most of these infections are attributable to Candida species followed by Aspergillus, while Pneumocystis jiroveci accounted for 13% of all fungal infections (111). The PHTS registry demonstrated P. jiroveci infection occurred in 1% of pediatric heart transplant recipients (112). Risk factors identified for fungal infections after multivariate analysis included previous surgery and mechanical support at the time of transplantation (111). Based on PHTS data, invasive fungal infections carry a mortality rate of 49% with all deaths occurring within the first 6 months following transplantation (111). Pneumocystis jiroveci has been shown to have a decreased mortality compared to other fungal infections (112). Current guidelines recommend prophylaxis against P. jiroveci with trimethoprim/sulfamethoxazole for 3-24 months (108).
Cardiac allograft vasculopathy (CAV)
CAV remains one of the leading causes of mortality and allograft loss in late survivors following pediatric HTx affecting 34% of patients by 10 years post-transplantation (2). Utilizing the UNOS registry, Kobayashi et al. demonstrated the incidence of CAV at 10 and 15 years post-transplantation was 25% and 54%, respectively (113). CAV typically manifests as a loss of distal coronary vasculature via intimal and medial proliferation and results in diastolic dysfunction and graft failure. The most recent ISHLT registry data show no difference in freedom from CAV based on the use of induction immunosuppression or the choice of calcineurin inhibitor (2). Identified risk factors for the development of CAV include ages 1-18 years at the time of transplant (but not infants), re-transplantation, recipient African-American race, and donor cigarette use (113). Currently, the gold standard for diagnosis of CAV is coronary angiography, although studies utilizing intravascular ultrasound and rotational angiography have been published (114-117). Cardiac magnetic resonance imaging and computed tomography have yet to be validated in children. Current medical management for CAV is limited. The introduction of m-TOR inhibitors, rapamycin and everolimus, have shown promise in slowing the progression of CAV and potentially preventing the development of CAV compared to azathioprine, but azathioprine has been predominantly replaced by mycophenolic acid/mycophenolate mofetil in current practice (118,119). However, given these studies, it is not unusual to either replace mycophenolate mofetil with an m-TOR inhibitor or add an m-TOR inhibitor to the medical regimen. In addition to m-TOR inhibitors, statins, particularly pravastatin, have also been shown to be beneficial in the treatment and potential prevention of CAV and safe for use in pediatrics (120-123). For the patient with a focal, proximal stenosis, percutaneous coronary stent placement may be indicated and has been shown to be safe in a pediatric population (124). For severe disease or progressive disease, treatment is limited to retransplantation. Following the diagnosis of CAV, the 1- and 3-year graft survivals are 66-77% and 52-60%, respectively, across the studied age groups (2).
Malignancy and PTLD
Malignancy remains a relatively uncommon complication post-transplant. The incidence of malignancy in the ISHTL registry at 5 and 10 years following transplantation is 5% and 9.5%, respectively, with PTLD making up the vast majority (2). The incidence is similar in the PHTS registry with 6% and 10% of patients developing PTLD at 5 and 10 years (125). PTLD can manifest in variable forms ranging from benign lymphoid hyperplasia to aggressive lymphoma. PTLD is typically an abnormal proliferation of B cells, and it is most often related to EBV (up to 87% of cases), but this need not be the case (109). PTLD most commonly arises from the gastrointestinal tract or lungs, but can manifest anywhere lymphoid tissue exists (109). In some studies, the use of induction immunosuppression has not been found to correlate with the development of PTLD (2,126) while the use and duration of induction immunosuppression has been shown to be risk factor in other studies (127-129). However, donor-recipient EBV mismatch and EBV viral load have been shown to be risk factors for the development of PTLD (128,129). Treatment for PTLD is dependent upon the histology, i.e., monomorphic or polymorphic. Initial treatment has historically included reduced immunosuppression, including potential discontinuation of anti-metabolites and significant reduction in calcineurin inhibitor. Immunosuppression reduction alone has been shown to lead to long-term disease remission in 40-86% of cases of PTLD in pediatric patients (130-132). Unfortunately, reduction of immunosuppression may lead to potential rejection as demonstrated in a PHTS study in which 61% of patients developed acute cellular rejection in the first 6 months following diagnosis of PTLD (109). Thus, other therapies have been investigated and employed especially in those patients in whom the risk of lowering immunosuppression outweighs the potential benefit. Rituximab, a chimeric mouse/human monoclonal antibody against CD20, has been shown to be effective in the treatment of PTLD (133,134). In some cases, particularly monomorphic PTLD, chemotherapy is warranted. Despite treatment, survival after diagnosis of PTLD is poor 75% of patients surviving 1 year and 67% of patients surviving 5 years (109).
Renal disease
Renal dysfunction is typically a consequence of nephrotoxicity secondary to calcineurin inhibitors. At 10 years post-transplantation, severe renal dysfunction, defined as either creatinine >2.5 mg/dL, dialysis, or renal transplant, is seen in 4% of patients transplanted as infants, 5% of patients transplanted between 1 and 5 years of age, 16% of patients transplanted between 6 and 10 years of age, and 14% of patients transplanted between 11 and 17 years of age (2). However, analysis of the PHTS data found that 71% of patients 5 years post-transplant and 57% of patients 10 years post-transplant had renal dysfunction defined as an estimated GFR <60 mL/min/1.73 m2 (135). Based on the ISHLT registry, there is no difference between the use of tacrolimus or cyclosporine in the development of severe renal dysfunction (2). Risk factors for development of late renal dysfunction include earlier era of HTx, African-American race, and rejection with hemodynamic compromise in the first year post-transplant, but renal function at the time of transplant was not found to be a risk factor (135). In the PHTS cohort, 1.4% of patients progressed to require chronic dialysis or renal transplantation (135).
Retransplantation
Given the complications above, all patients undergoing HTx will need to be considered for retransplantation. For the last decade, retransplantation has accounted from approximately 25-30% of pediatric heart transplants reported to the ISHLT (2). Retransplantation is more common in the older pediatric population with <1% of infants undergoing retransplantation, whereas retransplantation accounts for 9% of transplants in children 11-17 years of age based on the most recent ISHLT data (2). CAV and graft failure remain the most common causes of death (2), and CAV was the most common indication for retransplantation when reviewing both UNOS and PHTS data (136,137). Both of the aforementioned studies have demonstrated inferior survival compared to primary transplantation at all-time points. In particular, the 1-year survival for both studies is ~80%, and the PHTS data demonstrated a 5-year survival of 60% while the UNOS data found a 53% survival at 5 years (136,137). Also, both studies found that a shorter time frame from primary transplantation was a risk factor of decreased survival after retransplantation (136,137). Mahle et al. also found that mechanical ventilation prior to retransplantation was a risk factor for decreased survival (136). Given the limited organ supply, it is worth considering these factors when evaluating children for repeat transplantation.
Conclusions
Pediatric HTx has continued to evolve since first performed in 1967. With advances in surgical strategies and medical therapies, the outcomes for pediatric heart transplant recipients have continually improved. While significant post-transplant complications remain, including rejection, infection, malignancy, and CAV, heart transplant remains a therapeutic option to improve both the quality and quantity of life for pediatric patients. With continued research from individual institutions and large registries, including the ISHLT and PHTS, collective experience and understanding of pediatric heart transplant will translate to practice evolution which will ultimately decrease morbidity and enhance patient and graft survival.
Acknowledgements
Disclosure: The authors declare no conflict of interest.
References
- Kantrowitz A, Haller JD, Joos H, et al. Transplantation of the heart in an infant and an adult. Am J Cardiol 1968;22:782-90. [PubMed]
- Dipchand AI, Kirk R, Edwards LB, et al. The Registry of the International Society for Heart and Lung Transplantation: Sixteenth Official Pediatric Heart Transplantation Report--2013; focus theme: age. J Heart Lung Transplant 2013;32:979-88. [PubMed]
- Mudge GH, Goldstein S, Addonizio LJ, et al. 24th Bethesda conference: Cardiac transplantation. Task Force 3: Recipient guidelines/prioritization. J Am Coll Cardiol 1993;22:21-31. [PubMed]
- Costanzo MR, Augustine S, Bourge R, et al. Selection and treatment of candidates for heart transplantation. A statement for health professionals from the Committee on Heart Failure and Cardiac Transplantation of the Council on Clinical Cardiology, American Heart Association. Circulation 1995;92:3593-612. [PubMed]
- Mahle WT, Spray TL, Wernovsky G, et al. Survival after reconstructive surgery for hypoplastic left heart syndrome: A 15-year experience from a single institution. Circulation 2000;102:III136-41. [PubMed]
- Tweddell JS, Hoffman GM, Mussatto KA, et al. Improved survival of patients undergoing palliation of hypoplastic left heart syndrome: lessons learned from 115 consecutive patients. Circulation 2002;106:I82-9. [PubMed]
- Kimberling MT, Balzer DT, Hirsch R, et al. Cardiac transplantation for pediatric restrictive cardiomyopathy: presentation, evaluation, and short-term outcome. J Heart Lung Transplant 2002;21:455-9. [PubMed]
- Russo LM, Webber SA. Idiopathic restrictive cardiomyopathy in children. Heart 2005;91:1199-202. [PubMed]
- Bruns LA, Chrisant MK, Lamour JM, et al. Carvedilol as therapy in pediatric heart failure: an initial multicenter experience. J Pediatr 2001;138:505-11. [PubMed]
- Azeka E, Franchini Ramires JA, et al. Delisting of infants and children from the heart transplantation waiting list after carvedilol treatment. J Am Coll Cardiol 2002;40:2034-8. [PubMed]
- Shaddy RE, Curtin EL, Sower B, et al. The Pediatric Randomized Carvedilol Trial in Children with Heart Failure: rationale and design. Am Heart J 2002;144:383-9. [PubMed]
- Shaddy RE, Boucek MM, Hsu DT, et al. Carvedilol for children and adolescents with heart failure: a randomized controlled trial. JAMA 2007;298:1171-9. [PubMed]
- Hunt SA, Baker DW, Chin MH, et al. ACC/AHA Guidelines for the Evaluation and Management of Chronic Heart Failure in the Adult: Executive Summary A Report of the American College of Cardiology/American Heart Association Task Force on Practice Guidelines (Committee to Revise the 1995 Guidelines for the Evaluation and Management of Heart Failure): Developed in Collaboration With the International Society for Heart and Lung Transplantation; Endorsed by the Heart Failure Society of America. Circulation 2001;104:2996-3007. [PubMed]
- Rosenthal D, Chrisant MR, Edens E, et al. International Society for Heart and Lung Transplantation: Practice guidelines for management of heart failure in children. J Heart Lung Transplant 2004;23:1313-33. [PubMed]
- Canter CE, Shaddy RE, Bernstein D, et al. Indications for heart transplantation in pediatric heart disease: a scientific statement from the American Heart Association Council on Cardiovascular Disease in the Young; the Councils on Clinical Cardiology, Cardiovascular Nursing, and Cardiovascular Surgery and Anesthesia; and the Quality of Care and Outcomes Research Interdisciplinary Working Group. Circulation 2007;115:658-76. [PubMed]
- Hawkins JA, Breinholt JP, Lambert LM, et al. Class I and class II anti-HLA antibodies after implantation of cryopreserved allograft material in pediatric patients. J Thorac Cardiovasc Surg 2000;119:324-30. [PubMed]
- O'Connor MJ, Lind C, Tang X, et al. Persistence of anti-human leukocyte antibodies in congenital heart disease late after surgery using allografts and whole blood. J Heart Lung Transplant 2013;32:390-7. [PubMed]
- Wright EJ, Fiser WP, Edens RE, et al. Cardiac transplant outcomes in pediatric patients with pre-formed anti-human leukocyte antigen antibodies and/or positive retrospective crossmatch. J Heart Lung Transplant 2007;26:1163-9. [PubMed]
- Mahle WT, Tresler MA, Edens RE, et al. Allosensitization and outcomes in pediatric heart transplantation. J Heart Lung Transplant 2011;30:1221-7. [PubMed]
- Eckman PM. Immunosuppression in the sensitized heart transplant recipient. Curr Opin Organ Transplant 2010;15:650-6. [PubMed]
- Holt DB, Lublin DM, Phelan DL, et al. Mortality and morbidity in pre-sensitized pediatric heart transplant recipients with a positive donor crossmatch utilizing peri-operative plasmapheresis and cytolytic therapy. J Heart Lung Transplant 2007;26:876-82. [PubMed]
- Ratkovec RM, Hammond EH, O’Connell JB, et al. Outcome of cardiac transplant recipients with a positive donor-specific crossmatch--preliminary results with plasmapheresis. Transplantation 1992;54:651-5. [PubMed]
- Clark B, Cole JY, Wortley A, et al. Intravenous immunoglobulin-induced panel reactive antibody A reduction: not all preparations are created equal. Transplantation 2003;75:242-5. [PubMed]
- Itescu S, Burke E, Lietz K, et al. Intravenous pulse administration of cyclophosphamide is an effective and safe treatment for sensitized cardiac allograft recipients. Circulation 2002;105:1214-9. [PubMed]
- Leech SH, Lopez-Cepero M, LeFor WM, et al. Management of the sensitized cardiac recipient: the use of plasmapheresis and intravenous immunoglobulin. Clin Transplant 2006;20:476-84. [PubMed]
- Balfour IC, Fiore A, Graff RJ, et al. Use of rituximab to decrease panel-reactive antibodies. J Heart Lung Transplant 2005;24:628-30. [PubMed]
- Trivedi HL, Terasaki PI, Feroz A, et al. Abrogation of anti-HLA antibodies via proteasome inhibition. Transplantation 2009;87:1555-61. [PubMed]
- Morrow WR, Frazier EA, Mahle WT, et al. Rapid reduction in donor-specific anti-human leukocyte antigen antibodies and reversal of antibody-mediated rejection with bortezomib in pediatric heart transplant patients. Transplantation 2012;93:319-24. [PubMed]
- Patel J, Everly M, Chang D, et al. Reduction of alloantibodies via proteasome inhibition in cardiac transplantation. J Heart Lung Transplant 2011;30:1320-6. [PubMed]
- Zangwill SD, Ellis TM, Zlotocha J, et al. The virtual crossmatch--a screening tool for sensitized pediatric heart transplant recipients. Pediatr Transplant 2006;10:38-41. [PubMed]
- Zangwill S, Ellis T, Stendahl G, et al. Practical application of the virtual crossmatch. Pediatr Transplant 2007;11:650-4. [PubMed]
- Chang D, Kobashigawa J. The use of the calculated panel-reactive antibody and virtual crossmatch in heart transplantation. Curr Opin Organ Transplant 2012;17:423-6. [PubMed]
- Mah D, Singh TP, Thiagarajan RR, et al. Incidence and risk factors for mortality in infants awaiting heart transplantation in the USA. J Heart Lung Transplant 2009;28:1292-8. [PubMed]
- Almond CS, Gauvreau K, Thiagarajan RR, et al. Impact of ABO-incompatible listing on wait-list outcomes among infants listed for heart transplantation in the United States: a propensity analysis. Circulation 2010;121:1926-33. [PubMed]
- Henderson HT, Canter CE, Mahle WT, et al. ABO-incompatible heart transplantation: analysis of the Pediatric Heart Transplant Study (PHTS) database. J Heart Lung Transplant 2012;31:173-9. [PubMed]
- Patel ND, Weiss ES, Scheel J, et al. ABO-incompatible heart transplantation in infants: analysis of the united network for organ sharing database. J Heart Lung Transplant 2008;27:1085-9. [PubMed]
- Dipchand AI. Equivalent outcomes for pediatric heart transplantation recipients: ABO-blood group incompatible versus ABO-compatible. Am J Transplant 2010;10:389-97. [PubMed]
- Urschel S, Larsen IM, Kirk R, et al. ABO-incompatible heart transplantation in early childhood: an international multicenter study of clinical experiences and limits. J Heart Lung Transplant 2013;32:285-92. [PubMed]
- http://optn.transplant.hrsa.gov/PoliciesandBylaws2/policies/pdfs/policy_4.pdf
- Conway J, Chrisant MR, West LJ, et al. Outcomes of fetal listed patients awaiting heart transplantation. Pediatr Transplant 2013;17:653-60. [PubMed]
- Pollock-Barziv SM, McCrindle BW, West LJ, et al. Waiting before birth: outcomes after fetal listing for heart transplantation. Am J Transplant 2008;8:412-8. [PubMed]
- Dipchand AI, Kirk R, Mahle WT, et al. Ten yr of pediatric heart transplantation: a report from the Pediatric Heart Transplant Study. Pediatr Transplant 2013;17:99-111. [PubMed]
- Conway J, Chin C, Kemna M, et al. Donors’ characteristics and impact on outcomes in pediatric heart transplant recipients. Pediatr Transplant 2013;17:774-81. [PubMed]
- Tosi L, Federman M, Markovic D, et al. The effect of gender and gender match on mortality in pediatric heart transplantation. Am J Transplant 2013;13:2996-3002. [PubMed]
- Almond CS, Thiagarajan RR, Piercey GE, et al. Waiting list mortality among children listed for heart transplantation in the United States. Circulation 2009;119:717-27. [PubMed]
- Jeewa A, Manlhiot C, Kantor PF, et al. Risk factors for mortality or delisting of patients from the pediatric heart transplant waiting list. J Thorac Cardiovasc Surg 2014;147:462-8. [PubMed]
- Singh TP, Almond CS, Taylor DO, et al. Decline in heart transplant wait list mortality in the United States following broader regional sharing of donor hearts. Circ Heart Fail 2012;5:249-58. [PubMed]
- Voeller RK, Epstein DJ, Guthrie TJ, et al. Trends in the indications and survival in pediatric heart transplants: a 24-year single-center experience in 307 patients. Ann Thorac Surg 2012;94:807-15; discussion 815-6. [PubMed]
- Lipshultz SE, Sleeper LA, Towbin JA, et al. The incidence of pediatric cardiomyopathy in two regions of the United States. N Engl J Med 2003;348:1647-55. [PubMed]
- Towbin JA, Lowe AM, Colan SD, et al. Incidence, causes, and outcomes of dilated cardiomyopathy in children. JAMA 2006;296:1867-76. [PubMed]
- Kirk R, Naftel D, Hoffman TM, et al. Outcome of pediatric patients with dilated cardiomyopathy listed for transplant: a multi-institutional study. J Heart Lung Transplant 2009;28:1322-8. [PubMed]
- Pietra BA, Kantor PF, Bartlett HL, et al. Early predictors of survival to and after heart transplantation in children with dilated cardiomyopathy. Circulation 2012;126:1079-86. [PubMed]
- Singh TP, Almond CS, Piercey G, et al. Current outcomes in US children with cardiomyopathy listed for heart transplantation. Circ Heart Fail 2012;5:594-601. [PubMed]
- Gajarski R, Naftel DC, Pahl E, et al. Outcomes of pediatric patients with hypertrophic cardiomyopathy listed for transplant. J Heart Lung Transplant 2009;28:1329-34. [PubMed]
- Zangwill SD, Naftel D, L’Ecuyer T, et al. Outcomes of children with restrictive cardiomyopathy listed for heart transplant: a multi-institutional study. J Heart Lung Transplant 2009;28:1335-40. [PubMed]
- Decker JA, Rossano JW, Smith EO, et al. Risk factors and mode of death in isolated hypertrophic cardiomyopathy in children. J Am Coll Cardiol 2009;54:250-4. [PubMed]
- Canter CE, Naftel DC. Recipient characteristics. Heart transplantation. In: Fine R, Webber S, Harmon W, et al. eds. Pediatrc solid organ transplantation. Oxford (UK): Blackwell, in press.
- Lipshultz SE, Orav EJ, Wilkinson JD, et al. Risk stratification at diagnosis for children with hypertrophic cardiomyopathy: an analysis of data from the Pediatric Cardiomyopathy Registry. Lancet 2013;382:1889-97. [PubMed]
- Nugent AW, Daubeney PE, Chondros P, et al. Clinical features and outcomes of childhood hypertrophic cardiomyopathy: results from a national population-based study. Circulation 2005;112:1332-8. [PubMed]
- Maskatia SA, Decker JA, Spinner JA, et al. Restrictive physiology is associated with poor outcomes in children with hypertrophic cardiomyopathy. Pediatr Cardiol 2012;33:141-9. [PubMed]
- Colan SD, Lipshultz SE, Lowe AM, et al. Epidemiology and cause-specific outcome of hypertrophic cardiomyopathy in children: findings from the Pediatric Cardiomyopathy Registry. Circulation 2007;115:773-81. [PubMed]
- Maron BJ, Towbin JA, Thiene G, et al. Contemporary definitions and classification of the cardiomyopathies: an American Heart Association Scientific Statement from the Council on Clinical Cardiology, Heart Failure and Transplantation Committee; Quality of Care and Outcomes Research and Functional Genomics and Translational Biology Interdisciplinary Working Groups; and Council on Epidemiology and Prevention. Circulation 2006;113:1807-16. [PubMed]
- Nugent AW, Daubeney PE, Chondros P, et al. The epidemiology of childhood cardiomyopathy in Australia. N Engl J Med 2003;348:1639-46. [PubMed]
- Webber SA, Lipshultz SE, Sleeper LA, et al. Outcomes of restrictive cardiomyopathy in childhood and the influence of phenotype: a report from the Pediatric Cardiomyopathy Registry. Circulation 2012;126:1237-44. [PubMed]
- Cetta F, O’Leary PW, Seward JB, et al. Idiopathic restrictive cardiomyopathy in childhood: diagnostic features and clinical course. Mayo Clin Proc 1995;70:634-40. [PubMed]
- Denfield SW, Rosenthal G, Gajarski RJ, et al. Restrictive cardiomyopathies in childhood. Etiologies and natural history. Tex Heart Inst J 1997;24:38-44. [PubMed]
- Rivenes SM, Kearney DL, Smith EO, et al. Sudden death and cardiovascular collapse in children with restrictive cardiomyopathy. Circulation 2000;102:876-82. [PubMed]
- Azakie T, Merklinger SL, McCrindle BW, et al. Evolving strategies and improving outcomes of the modified norwood procedure: a 10-year single-institution experience. Ann Thorac Surg 2001;72:1349-53. [PubMed]
- Chinnock RE, Bailey LL. Heart transplantation for congenital heart disease in the first year of life. Curr Cardiol Rev 2011;7:72-84. [PubMed]
- Chrisant MR, Naftel DC, Drummond-Webb J, et al. Fate of infants with hypoplastic left heart syndrome listed for cardiac transplantation: a multicenter study. J Heart Lung Transplant 2005;24:576-82. [PubMed]
- Guleserian KJ, Armsby LB, Thiagarajan RR, et al. Natural history of pulmonary atresia with intact ventricular septum and right-ventricle-dependent coronary circulation managed by the single-ventricle approach. Ann Thorac Surg 2006;81:2250-7; discussion 2258. [PubMed]
- Rychik J, Levy H, Gaynor JW, et al. Outcome after operations for pulmonary atresia with intact ventricular septum. J Thorac Cardiovasc Surg 1998;116:924-31. [PubMed]
- Ashburn DA, Blackstone EH, Wells WJ, et al. Determinants of mortality and type of repair in neonates with pulmonary atresia and intact ventricular septum. J Thorac Cardiovasc Surg 2004;127:1000-7; discussion 1007-8. [PubMed]
- Larsen RL, Eguchi JH, Mulla NF, et al. Usefulness of cardiac transplantation in children with visceral heterotaxy (asplenic and polysplenic syndromes and single right-sided spleen with levocardia) and comparison of results with cardiac transplantation in children with dilated cardiomyopathy. Am J Cardiol 2002;89:1275-9. [PubMed]
- Auerbach SR, Smith JK, Gralla J, et al. Graft survival is better without prior surgery in cardiac transplantation for functionally univentricular hearts. J Heart Lung Transplant 2012;31:987-95. [PubMed]
- Marino BS. Outcomes after the Fontan procedure. Curr Opin Pediatr 2002;14:620-6. [PubMed]
- Hess J. Long-term problems after cavopulmonary anastomosis: diagnosis and management. Thorac Cardiovasc Surg 2001;49:98-100. [PubMed]
- Freedom RM, Hamilton R, Yoo SJ, et al. The Fontan procedure: analysis of cohorts and late complications. Cardiol Young 2000;10:307-31. [PubMed]
- Rychik J, Goldberg D, Rand E, et al. End-organ consequences of the Fontan operation: liver fibrosis, protein-losing enteropathy and plastic bronchitis. Cardiol Young 2013;23:831-40. [PubMed]
- Lastinger L, Zaidi AN. The adult with a fontan: a panacea without a cure? Review of long-term complications. Circ J 2013;77:2672-81. [PubMed]
- Backer CL, Deal BJ, Mavroudis C, et al. Conversion of the failed Fontan circulation. Cardiol Young 2006;16 Suppl 1:85-91. [PubMed]
- Mavroudis C, Deal BJ, Backer CL. The beneficial effects of total cavopulmonary conversion and arrhythmia surgery for the failed Fontan. Semin Thorac Cardiovasc Surg Pediatr Card Surg Annu 2002;5:12-24. [PubMed]
- Sheikh AM, Tang AT, Roman K, et al. The failing Fontan circulation: successful conversion of atriopulmonary connections. J Thorac Cardiovasc Surg 2004;128:60-6. [PubMed]
- Jacobs JP, Quintessenza JA, Chai PJ, et al. Rescue cardiac transplantation for failing staged palliation in patients with hypoplastic left heart syndrome. Cardiol Young 2006;16:556-62. [PubMed]
- Gossett JG, Almond CS, Kirk R, et al. Outcomes of cardiac transplantation in single-ventricle patients with plastic bronchitis: a multicenter study. J Am Coll Cardiol 2013;61:985-6. [PubMed]
- Brancaccio G, Carotti A, D’Argenio P, et al. Protein-losing enteropathy after Fontan surgery: resolution after cardiac transplantation. J Heart Lung Transplant 2003;22:484-6. [PubMed]
- Backer CL, Russell HM, Pahl E, et al. Heart transplantation for the failing Fontan. Ann Thorac Surg 2013;96:1413-9. [PubMed]
- Bernstein D, Naftel D, Chin C, et al. Outcome of listing for cardiac transplantation for failed Fontan: a multi-institutional study. Circulation 2006;114:273-80. [PubMed]
- Carlo WF, Pearce FB, Kirklin JK. Retransplantation as a successful therapy for post-transplant protein-losing enteropathy. J Heart Lung Transplant 2013;32:472-3. [PubMed]
- Gossett JG, Canter CE, Zheng J, et al. Decline in rejection in the first year after pediatric cardiac transplantation: a multi-institutional study. J Heart Lung Transplant 2010;29:625-32. [PubMed]
- Ameduri RK, Zheng J, Schechtman KB, et al. Has late rejection decreased in pediatric heart transplantation in the current era? A multi-institutional study. J Heart Lung Transplant 2012;31:980-6. [PubMed]
- Chin C, Naftel DC, Singh TP, et al. Risk factors for recurrent rejection in pediatric heart transplantation: a multicenter experience. J Heart Lung Transplant 2004;23:178-85. [PubMed]
- Webber SA, Naftel DC, Parker J, et al. Late rejection episodes more than 1 year after pediatric heart transplantation: risk factors and outcomes. J Heart Lung Transplant 2003;22:869-75. [PubMed]
- Pahl E, Naftel DC, Canter CE, et al. Death after rejection with severe hemodynamic compromise in pediatric heart transplant recipients: a multi-institutional study. J Heart Lung Transplant 2001;20:279-87. [PubMed]
- Everitt MD, Pahl E, Schechtman KB, et al. Rejection with hemodynamic compromise in the current era of pediatric heart transplantation: a multi-institutional study. J Heart Lung Transplant 2011;30:282-8. [PubMed]
- Ringewald JM, Gidding SS, Crawford SE, et al. Nonadherence is associated with late rejection in pediatric heart transplant recipients. J Pediatr 2001;139:75-8. [PubMed]
- Boucek MM, Edwards LB, Keck BM, et al. The Registry of the International Society for Heart and Lung Transplantation: Sixth Official Pediatric Report--2003. J Heart Lung Transplant 2003;22:636-52. [PubMed]
- Kulikowska A, Boslaugh SE, Huddleston CB, et al. Infectious, malignant, and autoimmune complications in pediatric heart transplant recipients. J Pediatr 2008;152:671-7. [PubMed]
- Schowengerdt KO, Naftel DC, Seib PM, et al. Infection after pediatric heart transplantation: results of a multiinstitutional study. The Pediatric Heart Transplant Study Group. J Heart Lung Transplant 1997;16:1207-16. [PubMed]
- Gajarski RJ, Smith EO, Denfield SW, et al. Long-term results of triple-drug-based immunosuppression in nonneonatal pediatric heart transplant recipients. Transplantation 1998;65:1470-6. [PubMed]
- Tran L, Hébert D, Dipchand A, et al. Invasive pneumococcal disease in pediatric organ transplant recipients: a high-risk population. Pediatr Transplant 2005;9:183-6. [PubMed]
- Stovall SH, Ainley KA, Mason EO Jr, et al. Invasive pneumococcal infections in pediatric cardiac transplant patients. Pediatr Infect Dis J 2001;20:946-50. [PubMed]
- Lin PL, Michaels MG, Green M, et al. Safety and immunogenicity of the American Academy of Pediatrics--recommended sequential pneumococcal conjugate and polysaccharide vaccine schedule in pediatric solid organ transplant recipients. Pediatrics 2005;116:160-7. [PubMed]
- Gennery AR, Cant AJ, Spickett GP, et al. Effect of immunosuppression after cardiac transplantation in early childhood on antibody response to polysaccharide antigen. Lancet 1998;351:1778-81. [PubMed]
- Fishman JA, Rubin RH. Infection in organ-transplant recipients. N Engl J Med 1998;338:1741-51. [PubMed]
- Rubin RH, Tolkoff-Rubin NE. Infection in the organ transplant patient; the Hegelian dialectic of transplantation. Trans Med Soc Lond 1994-1995;111:81-90. [PubMed]
- Rubin RH. Prevention and treatment of cytomegalovirus disease in heart transplant patients. J Heart Lung Transplant 2000;19:731-5. [PubMed]
- Costanzo MR, Dipchand A, Starling R, et al. The International Society of Heart and Lung Transplantation Guidelines for the care of heart transplant recipients. J Heart Lung Transplant 2010;29:914-56. [PubMed]
- Webber SA, Naftel DC, Fricker FJ, et al. Lymphoproliferative disorders after paediatric heart transplantation: a multi-institutional study. Lancet 2006;367:233-9. [PubMed]
- Pandya A, Wasfy S, Hébert D, et al. Varicella-zoster infection in pediatric solid-organ transplant recipients: a hospital-based study in the prevaricella vaccine era. Pediatr Transplant 2001;5:153-9. [PubMed]
- Zaoutis TE, Webber S, Naftel DC, et al. Invasive fungal infections in pediatric heart transplant recipients: incidence, risk factors, and outcomes. Pediatr Transplant 2011;15:465-9. [PubMed]
- Ng B, Dipchand A, Naftel D, et al. Outcomes of Pneumocystis jiroveci pneumonia infections in pediatric heart transplant recipients. Pediatr Transplant 2011;15:844-8. [PubMed]
- Kobayashi D, Du W, L’ecuyer TJ. Predictors of cardiac allograft vasculopathy in pediatric heart transplant recipients. Pediatr Transplant 2013;17:436-40. [PubMed]
- Costello JM, Wax DF, Binns HJ, et al. A comparison of intravascular ultrasound with coronary angiography for evaluation of transplant coronary disease in pediatric heart transplant recipients. J Heart Lung Transplant 2003;22:44-9. [PubMed]
- Kobashigawa JA, Tobis JM, Starling RC, et al. Multicenter intravascular ultrasound validation study among heart transplant recipients: outcomes after five years. J Am Coll Cardiol 2005;45:1532-7. [PubMed]
- Kuhn MA, Jutzy KR, Deming DD, et al. The medium-term findings in coronary arteries by intravascular ultrasound in infants and children after heart transplantation. J Am Coll Cardiol 2000;36:250-4. [PubMed]
- Gudausky TM, Pelech AN, Stendahl G, et al. Dual-axis rotational coronary angiography: a new technique for detecting graft coronary vasculopathy in pediatric heart transplant recipients. Pediatr Cardiol 2013;34:560-5. [PubMed]
- Mancini D, Pinney S, Burkhoff D, et al. Use of rapamycin slows progression of cardiac transplantation vasculopathy. Circulation 2003;108:48-53. [PubMed]
- Eisen HJ, Tuzcu EM, Dorent R, et al. Everolimus for the prevention of allograft rejection and vasculopathy in cardiac-transplant recipients. N Engl J Med 2003;349:847-58. [PubMed]
- Pahl E, Crawford SE, Wax DF, et al. Safety and efficacy of pravastatin in pediatric heart transplant recipients. J Heart Lung Transplant 2001;20:230. [PubMed]
- Kobashigawa JA, Moriguchi JD, Laks H, et al. Ten-year follow-up of a randomized trial of pravastatin in heart transplant patients. J Heart Lung Transplant 2005;24:1736-40. [PubMed]
- Kobashigawa JA, Katznelson S, Laks H, et al. Effect of pravastatin on outcomes after cardiac transplantation. N Engl J Med 1995;333:621-7. [PubMed]
- Chin C, Lukito SS, Shek J, et al. Prevention of pediatric graft coronary artery disease: atorvastatin. Pediatr Transplant 2008;12:442-6. [PubMed]
- Sachdeva R, Seib PM, Frazier EA, et al. Percutaneous coronary intervention using drug-eluting stents in pediatric heart transplant recipients. Pediatr Transplant 2009;13:1014-9. [PubMed]
- Chinnock R, Webber SA, Dipchand AI, et al. A 16-year multi-institutional study of the role of age and EBV status on PTLD incidence among pediatric heart transplant recipients. Am J Transplant 2012;12:3061-8. [PubMed]
- Gajarski RJ, Blume ED, Urschel S, et al. Infection and malignancy after pediatric heart transplantation: the role of induction therapy. J Heart Lung Transplant 2011;30:299-308. [PubMed]
- Opelz G, Döhler B. Lymphomas after solid organ transplantation: a collaborative transplant study report. Am J Transplant 2004;4:222-30. [PubMed]
- Manlhiot C, Pollock-Barziv SM, Holmes C, et al. Post-transplant lymphoproliferative disorder in pediatric heart transplant recipients. J Heart Lung Transplant 2010;29:648-57. [PubMed]
- Schubert S, Renner C, Hammer M, et al. Relationship of immunosuppression to Epstein-Barr viral load and lymphoproliferative disease in pediatric heart transplant patients. J Heart Lung Transplant 2008;27:100-5. [PubMed]
- Benkerrou M, Durandy A, Fischer A. Therapy for transplant-related lymphoproliferative diseases. Hematol Oncol Clin North Am 1993;7:467-75. [PubMed]
- Cacciarelli TV, Green M, Jaffe R, et al. Management of posttransplant lymphoproliferative disease in pediatric liver transplant recipients receiving primary tacrolimus (FK506) therapy. Transplantation 1998;66:1047-52. [PubMed]
- Newell KA, Alonso EM, Whitington PF, et al. Posttransplant lymphoproliferative disease in pediatric liver transplantation. Interplay between primary Epstein-Barr virus infection and immunosuppression. Transplantation 1996;62:370-5. [PubMed]
- Pescovitz MD. The use of rituximab, anti-CD20 monoclonal antibody, in pediatric transplantation. Pediatr Transplant 2004;8:9-21. [PubMed]
- Blaes AH, Peterson BA, Bartlett N, et al. Rituximab therapy is effective for posttransplant lymphoproliferative disorders after solid organ transplantation: results of a phase II trial. Cancer 2005;104:1661-7. [PubMed]
- Feingold B, Zheng J, Law YM, et al. Risk factors for late renal dysfunction after pediatric heart transplantation: a multi-institutional study. Pediatr Transplant 2011;15:699-705. [PubMed]
- Mahle WT, Vincent RN, Kanter KR. Cardiac retransplantation in childhood: analysis of data from the United Network for Organ Sharing. J Thorac Cardiovasc Surg 2005;130:542-6. [PubMed]
- Chin C, Naftel D, Pahl E, et al. Cardiac re-transplantation in pediatrics: a multi-institutional study. J Heart Lung Transplant 2006;25:1420-4. [PubMed]