Oxygenation impairment in patients with acute aortic dissection is associated with disorders of coagulation and fibrinolysis: a prospective observational study
Introduction
Despite recent advancements in medical technology, acute aortic dissection (AAD) is an age-dependent, clinically serious, urgent, and life-threatening cardiovascular disease associated with high morbidity and mortality due to potentially fatal complications (1). The mortality rate of AAD has been reported to be as high as 1–2%/h from the onset of symptoms, and the mortality rate is 33% within the first 24 h, 50% within the first 48 h, 75% within 2 weeks, and 90% after three months for patients who have not been treated promptly (2).
Acute lung injury (ALI) and acute respiratory distress syndrome (ARDS) are still life-threatening diseases in critically ill patients and are common complications during perioperative management and can seriously impact patient prognosis (3). The current overall mortality rate of ARDS is still more than 40% (4), and recent reports suggest that more than half of AAD patients will experience varying degrees of hypoxemia (5-7).
Prior investigations have indicated that inflammation (8-10), coagulation, and fibrinolysis (11-17) are associated with AAD pathogenesis and AAD-associated mortality. However, studies on the role of coagulation and fibrinolysis in AAD-induced ALI are still lacking. To the best of our knowledge, the correlation between the biomarkers of coagulation and fibrinolysis in bronchoalveolar lavage fluid (BALF) and AAD-induced ALI has not been reported.
Therefore, the purpose of this investigation was to assess the impact of preoperative ALI on postoperative oxygenation impairment and explore the correlation between preoperative ALI and tissue factor (TF), tissue factor pathway inhibitor (TFPI), and plasminogen activator inhibitor-1 (PAI-1) in blood and BALF. We also sought to clarify the associated factors of preoperative oxygenation impairment.
Methods
Design and participants
The investigation was a single-center, observational, prospective study, and was registered at www.clinicaltrials.gov (Identifier: NCT01894334; Date: June 8, 2013). A total of 53 Stanford type-A AAD patients were enrolled from October 2013 to July 2014 in Beijing Anzhen Hospital, an academic hospital in China. Inclusion criteria included patients aged 18–70 years old, those previously diagnosed Stanford type-A AAD, an onset time of fewer than two weeks, and patients scheduled for emergency surgery. Exclusion criteria included serious cardiopulmonary disease, chronic diseases of the liver, renal, and nervous system, coagulation disease, inflammatory disease, recent use of anti-inflammatory and anti-coagulant medication, and rejection of consent. Based on the presence or lack of ALI before surgery, the subjects were divided into the ALI group (group A, 22 subjects) or the control group (group C, 31 subjects). The primary outcome was patient oxygenation index and the secondary outcomes were concentrations of TF, TFPI, and PAI-1 in serum and BALF. All methods in this study were performed in accordance with relevant guidelines and regulations and were approved by the Clinical Research Ethics Committee of Beijing Anzhen Hospital (Identifier: 2012013). Written informed consent was obtained from all subjects before inclusion in this study.
Definitions
All subjects underwent contrast-enhanced computed tomography (CT) or magnetic resonance imaging (MRI) to confirm the diagnosis of Stanford type-A AAD according to the Stanford classification (18). All subjects underwent arterial blood gas analysis and chest radiograph for the diagnosis of ALI and ARDS, based on the combination of the criteria published by the American European Consensus Conference in 1994 (19), and the “Berlin criteria” published by the European Society of Intensive Care Medicine in 2012 (20). These criteria include the presence of risk factors, acute onset, oxygenation index (oxygen arterial pressure/oxygen inspired fraction, PaO2/FiO2) ≤300 mmHg for ALI, oxygenation index ≤200 mmHg for ARDS regardless of ventilator settings, appearance of bilateral pulmonary infiltration on chest radiograph, and oxygenation impairment not fully explained by cardiac failure.
Anesthesia and surgery
All subjects underwent established monitoring of radial artery pressure, dorsalis pedis artery pressure, and central venous pressure before surgery. The anesthesia protocol was standardized and included etomidate 0.3 mg/kg, rocuronium 0.6 mg/kg, and sufentanil 1–3 µg/kg for anesthesia induction, and midazolam, propofol, pancuronium, and sufentanil for anesthesia maintenance. The Bispectral index was maintained between 40 and 60 during surgery.
All surgical procedures were performed with the patient under general anesthesia, cardiopulmonary bypass (CPB), deep hypothermic circulatory arrest (DHCA), and selective cerebral perfusion using a modified elephant trunk technique named after Dr. Li-Zhong Sun. “Sun’s surgery” refers to a total arch replacement using a graft with four branches and implantation of a special stented endovascular graft.
Indicators and methods
The oxygenation index was calculated using the formula PaO2/FiO2. Blood was collected at four-time points: after hospital admission (T1), before surgery (T2), at the end of surgery (T3), and 12 h after surgery (T4). BALF was collected at T2 and T3. Briefly, the right main bronchus was located, and a bronchial occluder was inserted by fiberoptic bronchoscopy for BALF collection. Rapid infusion of 3 mL/kg sterile saline (37 °C) was performed, followed by the reclamation of lavage fluid using 50–100 mmHg negative pressure aspiration. The recovery rate was controlled at 40–60%, and then the recovered liquid was immediately filtered with double sterile gauze to remove mucus and to record the total amount for each sample. Finally, each sample was immediately centrifuged (1,500 ×g, 10 min) and stored in a refrigerator at −80 °C for later analysis. TF, TFPI, and PAI-1 were assayed using an ELISA kit (Lanyuan Biotechnology Co., Ltd, Shanghai, China). All ELISA assays were tested twice, and the mean value was used for analysis.
Statistical analysis
All statistical analyses were performed using IBM SPSS software version 23.0 (IBM Corporation, Armonk, NY, USA). Continuous variables are expressed as mean ± standard deviation and were analyzed with a Student’s t test for normally distributed data, and a Mann-Whitney U-test for data that did not exhibit a normal distribution. The categorical variables were expressed as percentages and analyzed with a Chi-squared test. Odds ratio (OR) and 95% confidence intervals (CI) were tested by a cross-table. Repeated-measures analysis of variance (ANOVA) was used to evaluate differences in parametric variables. The analyzed factors were phases (T1, T2, T3, and T4) and groups (group A and group C). The within-subjects factor was phase, and the between- subjects factor was group. If the effect of group and phase was significant, the simple main effects were tested. Mauchly’s test of sphericity was performed. If the sphericity assumption was violated, the degrees of freedom were corrected using the Greenhouse-Geisser epsilon. The Bonferroni post hoc test was used for pairwise comparisons. Variables showing a linear correlation in scatter plots with preoperative oxygenation index (T1) and having a P value <0.10 in Pearson linear correlation analysis were put into a multivariate logistic regression analysis model to identify associated factors for preoperative oxygenation impairment. A “Stepwise” methodology was employed and included variables in our multiple linear regression models that increased the probability of F by at least 0.05 and excluded variables if they increased F by less than 0.1. A two-tailed P value <0.05 was considered statistically significant.
Results
Subject demographics
There were no significant differences in subject demographics (Table 1). Study participants included 43 males and 10 females, aged from 23 to 66 years, with an average age of 46±10 years.
Subject perioperative data
Subject perioperative data are presented in Table 2. There were significant differences in intraoperative blood loss (1,524±458 versus 1,175±327 mL, P=0.040), mechanic ventilation time in the ICU (27.24±8.37 versus 17.33±7.36 h, P<0.001), stay in ICU (42.27±10.85 versus 33.45±9.05 h, P=0.002), and hospital stay (17.77±5.00 versus 13.48±3.97 days, P=0.001).
Oxygenation
The incidence of preoperative ALI for Stanford type-A AAD patients was 41.5% (22/53). Oxygenation index in group A and group C at T1, T2, T3, and T4 were 189.0±65.4 versus 366.3±56.8; 193.2±62.0 versus 361.5±46.2; 130.8±40.5 versus 299.6±48.4; and 104.6±31.7 versus 248.7±48.0 mmHg, respectively. Student’s t-test showed the oxygenation index in group A was lower than group C at each time point (P<0.001), and the oxygenation index of T3 andT4 was lower than T1 in both groups (P<0.001, Table 3).
Consecutive variables of oxygenation index at T4 were converted into binary variables representing ARDS and non-ARDS for assessing incidence. ARDS incidences were 86.36% (19/22) in group A and 25.81% (8/31) in group C. OR and 95% CI were calculated, OR =8.77 (95% CI, 2.14–35.96). Pearson Chi-square was also performed (Chi-square =10.62, P=0.001).
The concentrations of TF, TFPI, and PAI-1
The impacts of different groups and phases on the concentrations of TF, TFPI, and PAI-1 are presented in Table 4, and the profile plot shows the estimated mean values of TF, TFPI, and PAI-1 (Figure 1).
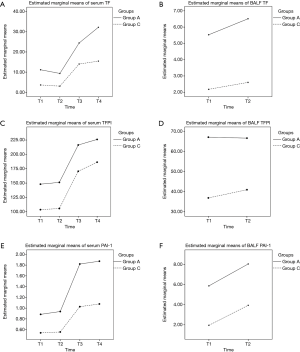
Serum concentrations of TF were significantly different across phases (F=134.23; P<0.001), and in both group A (F=96.09; P<0.001) and group C (F=91.61; P<0.001). They gradually increased and peaked at T4 in both groups. Serum concentrations of TF in group A were significantly higher than group C overall (F=133.67; P<0.001), and in all phases. There was a significant interaction between phase and group (F=8.57; P<0.001). BALF concentrations of TF had no difference among phases overall (F=3.32; P=0.074), and in both group A (F=2.00; P=0.163) and group C (F=1.53; P=0.222). BALF concentrations of TF in group A were significantly higher than group C overall (F=68.14; P<0.001), and in both phases. There was no interaction between phase and group (F=0.52; P=0.474).
Serum concentrations of TFPI showed significant differences among phases overall (F=58.97; P<0.001), and in both group A (F=17.87; P<0.001) and group C (F=44.87; P<0.001). They gradually increased and peaked at T4 in both groups. Serum concentrations of TFPI in group A were significantly higher than group C overall (F=31.98; P<0.001), and in all phases. There was no interaction between phase and group (F=0.07; P=0.944). BALF concentrations of TFPI had no difference among phases overall (F=1.04; P=0.313), and in both group A (F=0.01; P=0.927) and group C (F=1.45; P=0.235). BALF concentrations of TFPI in group A were significantly higher than group C overall (F=45.58; P<0.001), and in both phases. There was no interaction between phase and group (F=1.65; P=0.205).
Serum concentrations of PAI-1 had significant differences among phases overall (F=197.81; P<0.001), and in both group A (F=123.76; P<0.001) and group C (F=53.01; P<0.001). They gradually increased and peaked at T4 in both groups. Serum concentrations of PAI-1 in group A were significantly higher than group C overall (F=213.88; P<0.001), and in all phases. There was no interaction between phase and group (F=17.79; P=0.944). BALF concentrations of PAI-1 at T3 were significantly higher than T2 overall (F=83.11; P<0.001), and in both group A (F=17.01; P<0.001) and group C (F=35.36; P<0.001). BALF concentrations of PAI-1 in group A were significantly higher than group C overall (F=107.95; P<0.001), and in both phases. There was no interaction between phase and group (F=0.18; P=0.674).
The associated factors of preoperative oxygenation impairment
Multivariate linear regression analysis was used to evaluate which variables were associated with preoperative oxygenation impairment (Table 5). Serum TF (T1), serum TFPI (T1), serum PAI-1 (T1), BALF TF (T2), BALF TFPI (T2), and BALF PAI-1 (T2) had a linear correlation with preoperative oxygenation index (T1). We then put them into our multivariate linear regression analysis model. The adjusted R2 of our model is 0.786 with the R2 =0.811, indicating that the linear regression explained 78.6% of the variance in the data. The F-test was significant (AVONA: F6,46 =32.808, P<0.001), suggesting that the model explains a significant amount of the variance.

Full table
BALF PAI-1 (T2), serum TF (T1), and BALF TF (T2) were associated factors in our multivariate linear regression analysis model. The equation utilized was “Y =528.154−11.229 [BALF PAI-1 (T2)] –7.084 [serum TF (T1)] –7.818 [BALF TF (T2)]” (Table 6).
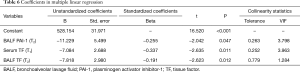
Full table
Discussion
Despite advancements in diagnostic and perioperative management modalities (21), AAD is associated with rapid progress course, complex treatment course, high complication incidence, and high morbidity and mortality rates (2).
The present investigation demonstrates that 41.5% of Stanford type-A AAD patients had suffered ALI before surgery. Sugano et al. (22) published a similar report claiming that 51% of patients evidence oxygenation impairment within 24 h after onset of AAD, while a cross-sectional clinical study from our institution (23) revealed that 53.8% of the patients undergoing Stanford type-A AAD surgery had preoperative hypoxemia. In addition, we found that if patients had suffered preoperative ALI, their oxygenation index would be lower, and they were more likely to develop to ARDS and had longer mechanic ventilation time in ICU longer stay in the ICU and longer hospital stay after surgery. Sheng et al. (7) also suggested that a preoperative PaO2/FiO2 ≤300 mmHg was an independent risk factor of hypoxemia after surgery for Stanford type-A AAD. Increased postoperative oxygenation impairment may occur due to an increased inflammatory response that is associated with the magnitude of the operative injury and the procedure (24-28). Therefore, taking measures to improve ALI earlier is crucial for the prognosis of Stanford type-A AAD patients.
Also, the current surgical treatment strategy for Stanford type-A AAD patients in our institution may be reevaluated and improved for the patients with preoperative oxygenation impairment. For example, the surgery time, CPB time, and circulatory arrest time should be shortened as much as possible by taking all feasible measures, and if the condition affords, a straightforward supra-coronary ascending aortic replacement should be performed instead of the total arch procedure to reduce the surgical trauma and CPB-induced inflammation.
Although a correlation between the degree of ALI in patients with AAD and inflammatory response has been demonstrated previously (29,30), the pathogenesis of AAD in conjunction with ALI, and the correlation of coagulation and fibrinolysis with ALI remains unclear. The meta-analysis by Shimony et al. (31) suggested that D-dimer is highly sensitive in the diagnosis of aortic dissection, and D-dimer <500 µg/L may assist in the exclusion of aortic dissection. The study of MacLaren et al. (17) also showed that intrapulmonary microthrombosis and fibrin deposition are two critical mechanisms associated with ALI due to the imbalance of coagulation and fibrinolysis.
The results of this investigation suggest that Stanford type-A AAD patients with preoperative ALI have persistently higher levels of TF and TFPI in serum and BALF. Importantly, TF in serum and BALF was measured before surgery and (T1) is an associated factor of preoperative oxygenation impairment for Stanford type-A AAD. TF is a 47-kD, membrane-bound protein and is a potent agonist of exogenous clotting cascade reactions. Under normal circumstances, the concentration of TF in blood is low, and the normal TF-dependent blood coagulation plays a major role in hemostasis after tissue injury (32). However, under abnormal circumstances, the abnormal TF-dependent coagulation activation can be the basis of some diseases including acute myocardial infarction and ALI (33,34). We inferred that when aortic dissection appears, the host defense response activates lymphocytes and macrophages, and endothelial cells release a large number of inflammatory cytokines that activate the transcription factor of neutrophils and macrophages, which will cause TF to be activated and released. Excessive TF in blood will be one of the main causes of preoperative oxygenation impairment.
In contrast, TFPI is composed of 276 amino acid residues and controls the natural anticoagulant protein in the priming phase of coagulation, which has a specific inhibitory effect on the TF pathway. We found that the concentrations of TFPI in serum and BALF were much lower than TF (concentration of TFPI was about 1% of that of TF), which caused that the TFPI pool in endothelial cells was exhausted and there was not enough existing TFPI to neutralize TF and reverse TF-induced activation of coagulation (35). Therefore, TFPI may play a relatively weak role in the regulation of coagulation and inflammation, but it cannot change the hypercoagulable state and has a limited effect on oxygenation for Stanford type-A AAD patients.
PAI-1 is the most important inhibitor of plasminogen activator and is an important component of the plasminogen/plasmin system. This investigation showed that Stanford type-A AAD patients with preoperative ALI have persistently higher levels of PAI-1, and the BALF PAI-1 at T2 was an associated factor of preoperative oxygenation impairment for Stanford type-A AAD. These results suggest that, in Stanford type-A AAD patients, the effect of PAI-1 on oxygenation may be achieved through the pulmonary alveoli instead of the systemic circulation. These results also explain the state of systemic fibrinolysis in AAD patients and the state of fibrinolysis inhibition caused by the increase of PAI-1. Acute fibrinolysis appeared in the early stage of AAD, which is associated with the increased plasminogen activator-induced by the activation of the host defense response (36). Following an early fibrinolysis reaction, there was a rapid and sustained production of PAI-1. However, the increase of PAI-1 in the blood is likely insufficient to completely “neutralize” the continuous increase of plasminogen activator-induced by the massive production of inflammatory cytokines and TF, which are still dominant in the blood of AAD patients. In addition, Montes et al. (37) reported that activated protein C could inhibit PAI-1, which may be another reason that fibrinolysis remains in the blood. We also found the concentration of PAI-1 in BALF was much higher than in the serum. This result was consistent with the findings of Prabhakaran et al. that illustrated the concentration of PAI-1in pulmonary edema fluid was higher than in blood for ALI patients, suggesting that there are alveolar origins for PAI-1, such as alveolar macrophages, alveolar type-2 epithelial cells, and fibroblasts (38-40). Moreover, local pulmonary medication is more efficacious than systemic medication (41). Therefore, PAI-1 may be an important indicator and cause of perioperative ALI for Stanford type-A AAD patients, but its effect is more likely in pulmonary alveoli, which further suggests that the choice of administration route is also crucial to the effectiveness of prophylaxis and treatment for ALI.
Therefore, moderate activation and disorder of coagulation and fibrinolysis in the pulmonary circulation can help the alveolar epithelial cells and repair of endothelial cell damage, while also reducing permeability. Unfortunately, excessive alveolar fibrin deposition will activate neutrophils and fibroblasts, release more inflammatory cytokines, increase vascular endothelial permeability, further promote thrombin generation and fibrin deposition, reduce alveolar surface activity substance production, and result in alveolar collapse and the occurrence of hypoxemia (13).
Despite its prospective design, this study was limited primarily by its small (n=53), single-center based population. We also cannot exclude the possibility that other factors may have impacted systemic oxygenation. Future investigations will examine other factors, such as key cytokines and chemokines in BALF, that may cause poor oxygenation. Furthermore, we did not collect BALF at T1 and T4 due to ethical and patient safety considerations. As a result, data that may be of value are lacking. Finally, this was an observational study that lacked an intervention. Despite the presence of these limitations, to our knowledge, this was the first investigation that exanimated TF, TFPI, and PAI-1 both in serum and in BALF, and compared them for Stanford type-A AAD patients with preoperative ALI and those without preoperative ALI. In addition, we found for the first time that preoperative BALF PAI-1 (T2), serum TF (T1), and BALF TF (T2) were associated factors of preoperative oxygenation impairment for Stanford type-A AAD. In the future, we will expand the sample size and give appropriate interventions, to further explore the mechanism of ALI, especially in coagulation and fibrinolysis. These studies will then provide a clinical basis for early intervention and may improve the prognosis of AAD patients.
In conclusion, preoperative ALI is associated with lower postoperative oxygenation index, higher postoperative ARDS incidence, longer mechanical ventilation time in the ICU, a longer stay in the ICU, longer total hospital stay, and persistently higher levels of TF, TFPI, and PAI-1 for Stanford type-A AAD patients. Preoperative BALF PAI-1, serum TF, and BALF TF are associated factors of preoperative oxygenation impairment for Stanford type-A AAD. Thus, activation and disorder of coagulation and fibrinolysis may help explain the association of the occurrence and progressive development of ALI with Stanford type-A AAD.
Acknowledgements
The authors thank Jeffrey Bortman for linguistic assistance during the preparation of this manuscript.
Footnote
Conflicts of Interest: The authors have no conflicts of interest to declare.
Ethical Statement: All methods in this study were performed in accordance with relevant guidelines and regulations and were approved by the Clinical Research Ethics Committee of Beijing Anzhen Hospital (Identifier: 2012013). Written informed consent was obtained from all subjects before inclusion in this study.
References
- Erbel R, Aboyans V, Boileau C, et al. 2014 ESC Guidelines on the diagnosis and treatment of aortic diseases: Document covering acute and chronic aortic diseases of the thoracic and abdominal aorta of the adult. The Task Force for the Diagnosis and Treatment of Aortic Diseases of the European Society of Cardiology (ESC). Eur Heart J 2014;35:2873-926. [Crossref] [PubMed]
- De León Ayala IA, Chen Y. Acute aortic dissection: an update. Kaohsiung J Med Sci 2012;28:299-305. [Crossref] [PubMed]
- Fanelli V, Vlachou A, Ghannadian S, et al. Acute respiratory distress syndrome: new definition, current and future therapeutic options. J Thorac Dis 2013;5:326-34. [PubMed]
- Villar J, Sulemanji D, Kacmarek RM. The acute respiratory distress syndrome: incidence and mortality, has it changed? Curr Opin Crit Care 2014;20:3-9. [Crossref] [PubMed]
- Wang Y, Xue S, Zhu H. Risk factors for postoperative hypoxemia in patients undergoing Stanford A aortic dissection surgery. J Cardiothorac Surg 2013;8:118. [Crossref] [PubMed]
- Nakajima T, Kawazoe K, Izumoto H, et al. Risk factors for hypoxemia after surgery for acute type A aortic dissection. Surg Today 2006;36:680-5. [Crossref] [PubMed]
- Sheng W, Yang H, Chi Y, et al. Independent risk factors for hypoxemia after surgery for acute aortic dissection. Saudi Med J 2015;36:940-6. [Crossref] [PubMed]
- Luo F, Zhou X, Li J, et al. Inflammatory response is associated with aortic dissection. Ageing Res Rev 2009;8:31-5. [Crossref] [PubMed]
- Li D, Ye L, Yu J, et al. Significance of the thrombo-inflammatory status-based novel prognostic score as a useful predictor for in-hospital mortality of patients with type B acute aortic dissection. Oncotarget 2017;8:79315-22. [PubMed]
- Wen D, Wu H, Jiang X, et al. Role of plasma C-reactive protein and white blood cell count in predicting in-hospital clinical events of acute type A aortic dissection. Chin Med J (Engl) 2011;124:2678-82. [PubMed]
- Tsai MT, Wu H, Roan JN, et al. Effect of false lumen partial thrombosis on repaired acute type A aortic dissection. J Thorac Cardiovasc Surg 2014;148:2140-6.e3. [Crossref] [PubMed]
- Nomura F, Tamura K, Yoshitatsu M, et al. Changes in coagulation condition, cytokine, adhesion molecule after repair of type A aortic dissection. Eur J Cardiothorac Surg 2004;26:348-50. [Crossref] [PubMed]
- Huang B, Tian L, Fan X, et al. Low admission platelet counts predicts increased risk of in-hospital mortality in patients with type A acute aortic dissection. Int J Cardiol 2014;172:e484-6. [Crossref] [PubMed]
- Wen D, Du X, Dong J, et al. Value of D-dimer and C reactive protein in predicting inhospital death in acute aortic dissection. Heart 2013;99:1192-7. [Crossref] [PubMed]
- Dong J, Bao J, Feng R, et al. Circulating microRNAs: a novel potential biomarker for diagnosing acute aortic dissection. Sci Rep 2017;7:12784. [Crossref] [PubMed]
- Dong J, Duan X, Feng R, et al. Diagnostic implication of fibrin degradation products and D-dimer in aortic dissection. Sci Rep 2017;7:43957. [Crossref] [PubMed]
- MacLaren R, Stringer KA. Emerging role of anticoagulants and fibrinolytics in the treatment of acute respiratory distress syndrome. Pharmacotherapy 2007;27:860-73. [Crossref] [PubMed]
- Daily PO, Trueblood HW, Stinson EB, et al. Management of acute aortic dissections. Ann Thorac Surg 1970;10:237-47. [Crossref] [PubMed]
- Bernard GR, Artigas A, Brigham KL, et al. The American-European Consensus Conference on ARDS. Definitions, mechanisms, relevant outcomes, and clinical trial coordination. Am J Respir Crit Care Med 1994;149:818-24. [Crossref] [PubMed]
- de Luis Cabezón N, Sanchez Castro I, Bengoetxea Uriarte UX, et al. Acute respiratory distress syndrome: a review of the Berlin definition. Rev Esp Anestesiol Reanim 2014;61:319-27. [PubMed]
- Patel PD, Arora RR. Pathophysiology, diagnosis, and management of aortic dissection. Ther Adv Cardiovasc Dis 2008;2:439-68. [Crossref] [PubMed]
- Sugano Y, Anzai T, Yoshikawa T, et al. Serum C-reactive protein elevation predicts poor clinical outcome in patients with distal type acute aortic dissection: association with the occurrence of oxygenation impairment. Int J Cardiol 2005;102:39-45. [Crossref] [PubMed]
- Pan X, Lu J, Cheng W, et al. Independent factors related to preoperative acute lung injury in 130 adults undergoing Stanford type-A acute aortic dissection surgery: a single-center cross-sectional clinical study. J Thorac Dis 2018;10:4413-23. [Crossref] [PubMed]
- Chen MF, Chen LW, Cao H, et al. Analysis of risk factors for and the prognosis of postoperative acute respiratory distress syndrome in patients with Stanford type A aortic dissection. J Thorac Dis 2016;8:2862-71. [Crossref] [PubMed]
- Watt DG, Horgan PG, McMillan DC. Routine clinical markers of the magnitude of the systemic inflammatory response after elective operation: a systematic review. Surgery 2015;157:362-80. [Crossref] [PubMed]
- Gu J, Hu J, Zhang H, et al. Time-dependent changes of plasma inflammatory biomarkers in type A aortic dissection patients without optimal medical management. J Cardiothorac Surg 2015;10:3. [Crossref] [PubMed]
- Komukai K, Shibata T, Mochizuki S. C-reactive protein is related to impaired oxygenation in patients with acute aortic dissection. Int Heart J 2005;46:795-9. [Crossref] [PubMed]
- Kurabayashi M, Okishige K, Azegami K, et al. Reduction of the PaO2/FiO2 ratio in acute aortic dissection - relationship between the extent of dissection and inflammation. Circ J 2010;74:2066-73. [Crossref] [PubMed]
- Orihashi K. Acute type a aortic dissection: for further improvement of outcomes. Ann Vasc Dis 2012;5:310-20. [Crossref] [PubMed]
- Guan X, Li J, Gong M, et al. The hemostatic disturbance in patients with acute aortic dissection: A prospective observational study. Medicine (Baltimore) 2016;95:e4710. [Crossref] [PubMed]
- Shimony A, Filion KB, Mottillo S, et al. Meta-analysis of usefulness of d-dimer to diagnose acute aortic dissection. Am J Cardiol 2011;107:1227-34. [Crossref] [PubMed]
- Mann KG, Brummel K, Butenas S. What is all that thrombin for? J Thromb Haemost 2003;1:1504-14. [Crossref] [PubMed]
- Broze GJ Jr. Tissue factor pathway inhibitor and the current concept of blood coagulation. Blood Coagul Fibrinolysis 1995;6 Suppl 1:S7-13. [Crossref] [PubMed]
- Miller DL, Welty-Wolf K, Carraway MS, et al. Extrinsic coagulation blockade attenuates lung injury and proinflammatory cytokine release after intratracheal lipopolysaccharide. Am J Respir Cell Mol Biol 2002;26:650-8. [Crossref] [PubMed]
- Abraham E, Reinhart K, Svoboda P, et al. Assessment of the safety of recombinant tissue factor pathway inhibitor in patients with severe sepsis: a multicenter, randomized, placebo-controlled, single-blind, dose escalation study. Crit Care Med 2001;29:2081-9. [Crossref] [PubMed]
- Renckens R, Roelofs JJ, Florquin S, et al. Endogenous tissue-type plasminogen activator is protective during Escherichia coli-induced abdominal sepsis in mice. J Immunol 2006;177:1189-96. [Crossref] [PubMed]
- Montes R, Rodriguez-Wilhelmi P, Hurtado V, et al. The endotoxin-induced plasminogen activator inhibitor-1 increase in rabbits is not tumor necrosis factor-alpha dependent and can occur in the absence of interleukin-1beta. Thromb Haemost 2002;88:639-43. [Crossref] [PubMed]
- Poole LG, Massey VL, Siow DL, et al. Plasminogen Activator Inhibitor-1 Is Critical in Alcohol-Enhanced Acute Lung Injury in Mice. Am J Respir Cell Mol Biol 2017;57:315-23. [Crossref] [PubMed]
- Prabhakaran P, Ware LB, White KE, et al. Elevated levels of plasminogen activator inhibitor-1 in pulmonary edema fluid are associated with mortality in acute lung injury. Am J Physiol Lung Cell Mol Physiol 2003;285:L20-8. [Crossref] [PubMed]
- Wygrecka M, Markart P, Ruppert C, et al. Cellular origin of pro-coagulant and (anti)-fibrinolytic factors in bleomycin-injured lungs. Eur Respir J 2007;29:1105-14. [Crossref] [PubMed]
- Murakami K, McGuire R, Cox RA, et al. Heparin nebulization attenuates acute lung injury in sepsis following smoke inhalation in sheep. Shock 2002;18:236-41. [Crossref] [PubMed]